-
PDF
- Split View
-
Views
-
Cite
Cite
Francisco J. L. Gordillo, José Aguilera, Carlos Jiménez, The response of nutrient assimilation and biochemical composition of Arctic seaweeds to a nutrient input in summer, Journal of Experimental Botany, Volume 57, Issue 11, August 2006, Pages 2661–2671, https://doi.org/10.1093/jxb/erl029
- Share Icon Share
Abstract
Twenty-one species of macroalgae (four Chlorophyta, eight Rhodophyta, and nine Phaeophyta) from the Kongsfjord (Norwegian Arctic) were examined for their response to nutrient enrichment (nitrate and phosphate) in the summer period. The enzymatic activities related to nutrient assimilation, external carbonic anhydrase (CAext, EC 4.2.1.1), nitrate reductase (NR, EC 1.6.6.1), and alkaline phosphatase (AP, EC 3.1.3.1), as well as the biochemical composition (total C and N, soluble carbohydrates, soluble proteins, and pigments) were measured. CAext activity was present in all species, and showed a general decrease after nutrient enrichment. Inversely, NR activity increased in most of the species examined. Changes in pigment ratios pointed to the implication of light harvesting system in the acclimation strategy. Despite enzymatic and pigmentary response, the Arctic seaweeds can be regarded as not being N-limited even in summer, as shown by the slight effect of nutrient enrichment on biochemical composition. The exception being the nitrophilic species Monostroma arcticum and, to a lesser extent, Acrosiphonia sp. For the rest of the species studied, changes in total internal C and N, soluble proteins, soluble carbohydrates, pigment content, and the internal pool of inorganic N were recorded only for particular species and no general pattern was shown. Acclimation to unexpected nutrient input seemed to ensure the maintenance of a stable biomass composition, rather than an optimized use of the newly available resource (except for the nitrophilic species). This indicates a high degree of resilience of the algal community to a disruption in the natural nutrient availability pattern.
Introduction
The Arctic coastal environment is characterized by relatively constant and near-freezing water temperatures (typically −2 to +4 °C) and strong seasonal variations in light and nutrient availability (Hanelt et al., 2001; Hop et al., 2002). The West Spitsbergen Current (WSC) has a strong Atlantic character and brings relatively warm and nutrient-rich waters to Kongsfjord (0 to +6 °C; Hanelt et al., 2004), supporting a relatively rich macroalgal community of around 50 species (Hop et al., 2002; Wiencke et al., 2004). In Kongsfjord both boreal and Arctic species are present, and their balance is influenced by oceanographic conditions (Atlantic versus Arctic) and glacial inputs. The influx of Atlantic water and melting of glaciers in this region has been linked to climate change (Svendsen et al., 2002) and thus algal communities of Kongsfjord function as a climate indicator at a local scale (Hop et al., 2002).
Two of the most relevant global phenomena that threaten ecosystems are the increase in atmospheric CO2 concentration (Ramanathan, 1998) and the increased load of nitrogen in both the terrestrial and aquatic environment (Galloway, 1998). Changing the availability of these nutrients will force a change in the acclimation strategies of the organisms. Seawater nitrate concentration in Kongsfjord is generally highest in winter and spring (c. 10 μmol l−1), but it drops rapidly following the sea-ice break up due to production of fast-growing microalgae, so that it becomes depleted from early summer to winter. Soluble reactive phosphorus (SRP) follows a similar pattern with maximum concentrations of c. 1 μmol l−1 (Aguilera et al., 2002). Nitrogen has long been recognized to limit macroalgal productivity (Lapointe and O'Conell, 1989). Nutrient uptake and assimilation characteristics of macroalgae from temperate waters have been shown to vary among populations and species in ways that optimize survival and growth under local nutrient supply conditions (Wheeler and Weidner, 1983; Hernández et al., 1993; Gordillo et al., 2001a). Arctic species are also reported to show nutritional strategies that allow them to cope with the long periods of darkness in winter and nutrient depletion in summer (Korb and Gerard, 2000a, b). Kelps living in the Arctic rely on stored photosynthates accumulated during the summer ice-free N-depleted period, supporting new growth during ice-covered N-sufficient winter. Traditionally, it was thought that this seasonal growth pattern was a direct consequence of N availability (Chapman and Lindley, 1980) as well as light availability (Henley and Dunton, 1997). However, it has become apparent that, at least in some species, this pattern is under the control of an endogenous free-running circannual rhythm entrained by a critical minimum daylength in autumn (Lüning, 1991; Schaffelke and Lüning, 1994), suggesting that the addition of nitrate-N to summer N-limited kelps would have only a marginal effect on growth and biochemical composition, presumably due to the prevailing internal clock (Henley and Dunton, 1997). As far as it is known this has never been tested at the community level in the Arctic, and it is still unknown whether the limited effect of N enrichment is only restricted to the few kelp species studied, or is a common characteristic in Arctic macroalgae.
Among the metabolic paths that are suspected to be most influenced by changing the nutritional conditions of the environment are the main nutrient assimilatory enzymes for CO2, nitrate and phosphate, as well as the light-harvesting strategy. External carbonic anhydrase (CAext) and nitrate reductase (NR) are well known as key regulatory enzymes for the C and N assimilation pathways, respectively. Carbonic anhydrases are involved in the conversion of to CO2, the only form of inorganic C used by Rubisco (Raven, 1991), as part of the carbon-concentrating mechanisms (CCMs). Many species shows CA activity due to an enzyme in the plasma membrane, referred to as external CA (CAext) independent from the internal CA which is present also in algae lacking a CCM. CAext is supressed by high CO2 levels, and enhanced by inorganic nitrogen enrichment (Jiménez del Río et al., 1995), so that changes in CAext activity due to nutrient enrichment are presumed to have a relevant (yet unknown) role in the acclimation strategy.
Nitrate reductase is usually regarded as the limiting step in nitrate assimilation, and is regulated by the external levels of nitrate (among other factors, such as light). The use of NR in an ecological context is particularly relevant for marine environments where nitrogen is often limiting, providing relevant information about the physiological N-status of the organisms (Hernández et al., 1993). The aim of this study was to provide an insight into whether an occasional input of nutrient (nitrate and phosphate) by Atlantic water during a period of external nutrient depletion would onset acclimation strategies that would lead to physiological disturbance, in representative species of the Arctic macroalgal community.
Materials and methods
Plant material and experimental set-up
Twenty-one species of macroalgae were collected from the Kongsfjord at Spitsbergen, Norwegian Arctic (78°55‘ N, 11°56’ E) during July 2002 at depths of 2–6 m; four Chlorophyta, eight Rhodophyta, and nine Phaeophyta (Table 1). The bathymetric and habitat location for each species are described in Wiencke et al. (2004) for most of the species studied. Visually healthy thalli, free from macroscopic epibiota were selected and incubated in 2.0 l aquaria for 72 h immediately after collection. Seawater was changed daily, and one set was enriched with 10 μM and 1 μM at the moment of water change (duplicate aquaria per set). Incubations were performed at 5 °C in continuous white fluorescent light of 30 μmol m−2 s−1. This irradiance was chosen according to the average daily irradiance recorded at depths of 1–5 m for this time of the year (Bischof et al., 2002). The aquaria were aerated at 0.5 l min−1. The enzymatic methods described below were applied to fresh material taken directly from the incubation aquaria. For species with differentiated tissue, the most active part of the thallus was chosen. Biochemical composition was analysed from lyophilized material stored at −80 °C.
List of species from the Kongsfjord examined, and their fresh weight to dry weight ratio (FW:DW)
Phylum | Name | Authority | FW:DW |
Chlorophyta | 1. Acrosiphonia sp. | C. Agardh | 4 |
2. Chaetomorpha melagonium | (F. Weber et Mohr) Kützing | 7.3 | |
3. Monostroma arcticum | Wittrock | 3.4 | |
4. Prasiola crispa | (Lightfoot) Kützing | 2.6 | |
Rhodophyta | 5. Ceramium strictum | (Kützing) Harvey | 6.1 |
6. Devaleraea ramentaceaa | (L.) Guiry | 6.3 | |
7. Odonthalia dentata | (L.) Lyngb. | 4 | |
8. Palmaria palmata | (L.) Kuntze | 6.6 | |
9. Phycodrys rubens | (L.) Batt. | 4.1 | |
10. Polysiphonia arctica | J. Agardh | 5 | |
11. Ptilota plumosa | (L.) C. Agardh | 3.6 | |
12. Rhodomela lycopodioides | (L.) C. Agardh | 6 | |
Phaeophyta | 13. Alaria esculenta | (L.) Grev. | 4 |
14. Chorda tomentosa | Lyngb. | 13.5 | |
15. Chordaria flagelliformis | (O.F.Muel.) C.Agardh | 7.2 | |
16. Desmarestia aculeata | (L.) Lamour. | 5.3 | |
17. Fucus distichus | L. | 4.8 | |
18. Laminaria saccharina | (L.) L. | 6.2 | |
19. Laminaria solidungulaa | J. Agardh | 6.5 | |
20. Scytosiphon lomentaria | (Lyngb.) Lynk | 13.7 | |
21. Sphacelaria plumosa | Lyngb. | 4.5 |
Phylum | Name | Authority | FW:DW |
Chlorophyta | 1. Acrosiphonia sp. | C. Agardh | 4 |
2. Chaetomorpha melagonium | (F. Weber et Mohr) Kützing | 7.3 | |
3. Monostroma arcticum | Wittrock | 3.4 | |
4. Prasiola crispa | (Lightfoot) Kützing | 2.6 | |
Rhodophyta | 5. Ceramium strictum | (Kützing) Harvey | 6.1 |
6. Devaleraea ramentaceaa | (L.) Guiry | 6.3 | |
7. Odonthalia dentata | (L.) Lyngb. | 4 | |
8. Palmaria palmata | (L.) Kuntze | 6.6 | |
9. Phycodrys rubens | (L.) Batt. | 4.1 | |
10. Polysiphonia arctica | J. Agardh | 5 | |
11. Ptilota plumosa | (L.) C. Agardh | 3.6 | |
12. Rhodomela lycopodioides | (L.) C. Agardh | 6 | |
Phaeophyta | 13. Alaria esculenta | (L.) Grev. | 4 |
14. Chorda tomentosa | Lyngb. | 13.5 | |
15. Chordaria flagelliformis | (O.F.Muel.) C.Agardh | 7.2 | |
16. Desmarestia aculeata | (L.) Lamour. | 5.3 | |
17. Fucus distichus | L. | 4.8 | |
18. Laminaria saccharina | (L.) L. | 6.2 | |
19. Laminaria solidungulaa | J. Agardh | 6.5 | |
20. Scytosiphon lomentaria | (Lyngb.) Lynk | 13.7 | |
21. Sphacelaria plumosa | Lyngb. | 4.5 |
Arctic endemic species.
List of species from the Kongsfjord examined, and their fresh weight to dry weight ratio (FW:DW)
Phylum | Name | Authority | FW:DW |
Chlorophyta | 1. Acrosiphonia sp. | C. Agardh | 4 |
2. Chaetomorpha melagonium | (F. Weber et Mohr) Kützing | 7.3 | |
3. Monostroma arcticum | Wittrock | 3.4 | |
4. Prasiola crispa | (Lightfoot) Kützing | 2.6 | |
Rhodophyta | 5. Ceramium strictum | (Kützing) Harvey | 6.1 |
6. Devaleraea ramentaceaa | (L.) Guiry | 6.3 | |
7. Odonthalia dentata | (L.) Lyngb. | 4 | |
8. Palmaria palmata | (L.) Kuntze | 6.6 | |
9. Phycodrys rubens | (L.) Batt. | 4.1 | |
10. Polysiphonia arctica | J. Agardh | 5 | |
11. Ptilota plumosa | (L.) C. Agardh | 3.6 | |
12. Rhodomela lycopodioides | (L.) C. Agardh | 6 | |
Phaeophyta | 13. Alaria esculenta | (L.) Grev. | 4 |
14. Chorda tomentosa | Lyngb. | 13.5 | |
15. Chordaria flagelliformis | (O.F.Muel.) C.Agardh | 7.2 | |
16. Desmarestia aculeata | (L.) Lamour. | 5.3 | |
17. Fucus distichus | L. | 4.8 | |
18. Laminaria saccharina | (L.) L. | 6.2 | |
19. Laminaria solidungulaa | J. Agardh | 6.5 | |
20. Scytosiphon lomentaria | (Lyngb.) Lynk | 13.7 | |
21. Sphacelaria plumosa | Lyngb. | 4.5 |
Phylum | Name | Authority | FW:DW |
Chlorophyta | 1. Acrosiphonia sp. | C. Agardh | 4 |
2. Chaetomorpha melagonium | (F. Weber et Mohr) Kützing | 7.3 | |
3. Monostroma arcticum | Wittrock | 3.4 | |
4. Prasiola crispa | (Lightfoot) Kützing | 2.6 | |
Rhodophyta | 5. Ceramium strictum | (Kützing) Harvey | 6.1 |
6. Devaleraea ramentaceaa | (L.) Guiry | 6.3 | |
7. Odonthalia dentata | (L.) Lyngb. | 4 | |
8. Palmaria palmata | (L.) Kuntze | 6.6 | |
9. Phycodrys rubens | (L.) Batt. | 4.1 | |
10. Polysiphonia arctica | J. Agardh | 5 | |
11. Ptilota plumosa | (L.) C. Agardh | 3.6 | |
12. Rhodomela lycopodioides | (L.) C. Agardh | 6 | |
Phaeophyta | 13. Alaria esculenta | (L.) Grev. | 4 |
14. Chorda tomentosa | Lyngb. | 13.5 | |
15. Chordaria flagelliformis | (O.F.Muel.) C.Agardh | 7.2 | |
16. Desmarestia aculeata | (L.) Lamour. | 5.3 | |
17. Fucus distichus | L. | 4.8 | |
18. Laminaria saccharina | (L.) L. | 6.2 | |
19. Laminaria solidungulaa | J. Agardh | 6.5 | |
20. Scytosiphon lomentaria | (Lyngb.) Lynk | 13.7 | |
21. Sphacelaria plumosa | Lyngb. | 4.5 |
Arctic endemic species.
External carbonic anhydrase assay
The external carbonic anhydrase (CAext, EC 4.2.1.1) activity was measured potentiometrically, according to Haglund et al. (1992). The assay was carried out at 0–2 °C determining the time taken for a linear drop in pH in the range 8.5 to 7.5 in a 3 ml volume cuvette containing a buffer (50 mM TRIS, 25 mM ascorbic acid, and 5 mM EDTA). Small pieces of algae weighing 150–250 mg FW were washed with distilled water and placed in the cuvette. The reaction was started by adding 1 ml of ice-cold CO2-saturated distilled water. One unit of relative enzymatic activity (REA) was defined as (t0/tc)–1, where t0 and tc are the time taken for the pH change in the absence and the presence of the alga, respectively. Typically, 5–6 independent replicates were assayed.
Nitrate reductase assay
Nitrate reductase (NR, EC 1.6.6.1) activity of fresh material was measured following the in situ method according to Corzo and Niell (1991), so that the enzyme is assayed in its original cellular location. The assay medium contained a buffer (0.2 M and 1 mM EDTA; pH 8), a compound able to permeabilize the membrane (0.1% propanol v/v), nitrate in excess (50 mM NaNO3), and a source of reducing power (10 μM glucose). The reaction was carried out by placing 30–70 mg FW of alga in a test tube containing 2 ml of the assay medium. The incubation time was 30 min at a temperature of 5 °C. The assay medium was bubbled with N2 gas for 2 min before and 2 min after placing the algal sample in order to remove the dissolved O2, that competes with in its reduction to . The incubation was performed in darkness to prevent further reduction of to . The activity is measured as the rate of produced. The in the assay medium was quantified spectrophotometrically according to Snell and Snell (1949). The observed activity is a potential measure of the NRA of the cell under the conditions prior to the assay. Typically, three independent replicates were assayed.
Alkaline phosphatase assay
The alkaline phosphatase (AP, EC 3.1.3.1 ) activity (APA) was assayed in 4–6 replicate samples of 50–100 mg FW using the artificial substrate p-nitrophenol phosphate (pNPP). This compound is cleaved by enzymatic hydrolysis rendering Pi and the coloured compound p-nitrophenyl (pNP) determined by absorbance at 410 nm. The procedure followed the method adapted for marine macrophytes by Hernández and Whitton (1996). The assay medium consisted of 2 ml of 500 μM pNPP (which enables maximum velocity of the enzymatic reaction) and 500 μM HEPES-NaOH buffer, pH 8.3, dissolved in P-free artificial seawater of 33 psu. The samples were incubated for 30 min at room temperature. Enzymatic activity was expressed as μmol pNP released g−1 FW h−1.
Carbon and nitrogen content
Total internal C and N were measured from lyophilized tissue samples using a C:H:N elemental analyser (Perkin-Elmer 2400CHN). Internal content of inorganic N (nitrate+nitrite) was estimated by grinding to powder lyophilized material (3–4 mg), and adding 10 ml deionized water. The extracts were incubated at 30 °C for 30 min, and then filtered (Whatman GF/C). Nitrate was determined in the aqueous fraction by using an automated autoanalyser (Traacs 800, BranLuebbe) using the method provided by the manufacturer.
Biochemical composition
Soluble carbohydrates, soluble proteins, phycobiliproteins, chlorophyll a, chlorophyll b, and total carotenoids were determined from material lyophilized right after collection from the incubation aquaria.
Soluble carbohydrates were estimated colorimetrically based on Kochert (1978) using phenol–sulphuric acid for colour development and glucose as standard.
Total soluble proteins from crude extracts (phosphate buffer, pH 6.5) were determined using a commercial protein assay (Bio-Rad), based on Bradford (1976). Protein content was determined spectrophotometrically at 595 nm and concentrations were calculated compared with a standard of bovine serum albumin. Phycoerythrin and phycocyanin were determined from the same extracts using the equations given by Beer and Eshel (1985) for spectrophotometrical quantification. Chlorophyll a, chlorophyll b, and total carotenoids were extracted in N, N dimethyl-formamide overnight (room temperature) after rehydrating lyophilized thalli. For spectrophotometric quantification the equations given by Wellburn (1994) were used. Tests performed in this laboratory demonstrated that the lyophilization process does not alter the absorbance characteristics of pigments, so that determination was not significantly affected (t test, P <0.01).
Data analyses
Replicate measurements (n=2–6 independent thalli) were tested for significance of differences (P <0.05) promoted by nutrient enrichment for each species using t tests. In addition, phylum-grouped species were tested for significance of differences by two-way ANOVAs followed by Tukey's paired tests (P <0.05). All tests were performed using the SigmaStat 2.03 statistical software (SPSS Inc.).
All variables measured for the 21 species studied (Tables 2–5345), except chlorophyll b and phycoerythrin, were included in two principal component analyses (PCA), for control and enriched conditions, respectively. The PCA is one of the best-known ordination methods. Mathematically, PCA consists of an eigenanalysis of a covariance or correlation matrix calculated on the original measurement data. Graphically, it can be described as a rotation of a swarm of data points in multidimensional space so that the longest axis (the axis with the greatest variance) is the first PCA axis, the second longest axis perpendicular to the first is the second PCA axis, and so forth. Thus the first few PCA axes represent the greatest amount of variation in the data set and usually contain some patterns of significance (for clarity, only the first two axes for each analysis is shown). Each new axis is a linear combination of the variables measured, i.e. each variable contributes a certain amount (called loading factor, Table 7) to the definition of each new axis. The analyses were performed using the software MVSP 3.12 (Kovach Computer Services).
Activity of the assimilating enzymes external carbonic anhydrase (CAext, REA g−1 FW), nitrate reductase (NRA, μmol g−1 FW h−1), and alkaline phosphatase (APA, μmol PNP g−1 FW h−1)
Phylum | Name | CAext | NRA | APA | |||
Control | Enriched | Control | Enriched | Control | Enriched | ||
Chlorophyta | Acrosiphonia sp. | 17.2 (1.4) | 12.3 (0.2)* | 2.67 (0.48) | 3.81 (0.91) | 0.39 (0.02) | 2.57 (0.32)* |
Chaetomorpha melagonium | 9.7 (2.3) | 15.8 (6.0) | 5.79 (0.80) | 3.97 (0.55)* | 0.29 (0.03) | 0.29 (0.03) | |
Monostroma arcticum | 33.4 (0.7) | 32.2 (2.3) | 5.16 (0.96) | 11.95 (1.43)* | 0.47 (0.25) | 0.32 (0.06) | |
Prasiola crispa | 59.0 (0.8) | 46.6 (3.3)* | 8.30 (0.47) | 8.94 (0.83) | 0.49 (0.06) | 0.89 (0.08)* | |
Rhodophyta | Ceramium strictum | 15.4 (0.8) | 16.4 (1.2) | 3.54 (0.39) | 4.95 (0.66)* | 1.45 (0.05) | 1.88 (0.05)* |
Devaleraea ramentacea | 3.6 (1.4) | 4.0 (0.4) | 1.16 (0.64) | 2.92 (0.50)* | 1.14 (0.16) | 0.79 (0.08)* | |
Odonthalia dentata | 11.9 (3.2) | 7.4 (2.3) | 8.48 (0.51) | 10.14 (0.31)* | 2.76 (0.40) | 2.06 (0.46) | |
Palmaria palmata | 3.9 (1.5) | 3.1 (1.4) | 1.48 (0.31) | 2.70 (1.11) | 0.75 (0.13) | 0.59 (0.05) | |
Phycodrys rubens | 24.9 (3.7) | 13.8 (0.1)* | 2.19 (0.49) | 1.57 (0.48) | 2.79 (0.83) | 2.33 (0.92) | |
Polysiphonia arctica | 11.6 (0.0) | 7.3 (0.1)* | 2.42 (0.61) | 5.37 (1.60)* | 2.69 (0.22) | 1.69 (0.22)* | |
Ptilota plumosa | 14.0 (0.7) | 10.8 (2.2) | 4.18 (0.54) | 3.92 (0.35) | 2.34 (0.35) | 1.59 (0.21)* | |
Rhodomela lycopodioides | 17.2 (1.6) | 6.4 (1.4)* | 2.19 (0.53) | 3.14 (0.59) | 2.67 (0.49) | 2.58 (0.32) | |
Phaeophyta | Alaria esculenta | 26.8 (1.3) | 14.5 (1.1)* | 2.34 (0.49) | 11.01 (2.35)* | 1.30 (0.73) | 0.57 (0.23) |
Chorda tomentosa | 32.0 (5.2) | 27.6 (3.9) | 1.53 (0.04) | 0.74 (0.02)* | 0.22 (0.02) | 0.43 (0.02)* | |
Chordaria flagelliformis | 14.7 (0.9) | 13.7 (1.3) | 1.03 (0.39) | 3.35 (1.06)* | 0.31 (0.06) | 0.34 (0.02) | |
Desmarestia aculeata | 18.8 (2.19) | 7.8 (1.3)* | 2.27 (0.28) | 1.97 (0.44) | 0.22 (0.02) | 0.43 (0.02)* | |
Fucus distichus | 8.4 (3.1) | 8.8 (1.2) | 1.22 (0.50) | 4.53 (0.35)* | 1.35 (0.27) | 0.74 (0.03)* | |
Laminaria saccharina | 23.6 (0.8) | 9.5 (1.4)* | 2.20 (0.38) | 2.35 (0.32) | 0.15 (0.03) | 0.21 (0.05) | |
Laminaria solidungula | 21.8 (0.6) | 8.0 (0.9)* | 3.44 (1.70) | 11.94 (1.17)* | 0.14 (0.01) | 0.08 (0.01)* | |
Scytosiphon lomentaria | 14.4 (2.4) | 15.3 (3.9) | 1.10 (0.32) | 1.66 (0.47) | 0.53 (0.05) | 0.46 (0.07) | |
Sphacelaria plumosa | 25.1 (0.6) | 24.7 (0.1) | 2.45 (0.06) | 3.53 (0.35) | 2.45 (0.32) | 3.20 (0.20)* |
Phylum | Name | CAext | NRA | APA | |||
Control | Enriched | Control | Enriched | Control | Enriched | ||
Chlorophyta | Acrosiphonia sp. | 17.2 (1.4) | 12.3 (0.2)* | 2.67 (0.48) | 3.81 (0.91) | 0.39 (0.02) | 2.57 (0.32)* |
Chaetomorpha melagonium | 9.7 (2.3) | 15.8 (6.0) | 5.79 (0.80) | 3.97 (0.55)* | 0.29 (0.03) | 0.29 (0.03) | |
Monostroma arcticum | 33.4 (0.7) | 32.2 (2.3) | 5.16 (0.96) | 11.95 (1.43)* | 0.47 (0.25) | 0.32 (0.06) | |
Prasiola crispa | 59.0 (0.8) | 46.6 (3.3)* | 8.30 (0.47) | 8.94 (0.83) | 0.49 (0.06) | 0.89 (0.08)* | |
Rhodophyta | Ceramium strictum | 15.4 (0.8) | 16.4 (1.2) | 3.54 (0.39) | 4.95 (0.66)* | 1.45 (0.05) | 1.88 (0.05)* |
Devaleraea ramentacea | 3.6 (1.4) | 4.0 (0.4) | 1.16 (0.64) | 2.92 (0.50)* | 1.14 (0.16) | 0.79 (0.08)* | |
Odonthalia dentata | 11.9 (3.2) | 7.4 (2.3) | 8.48 (0.51) | 10.14 (0.31)* | 2.76 (0.40) | 2.06 (0.46) | |
Palmaria palmata | 3.9 (1.5) | 3.1 (1.4) | 1.48 (0.31) | 2.70 (1.11) | 0.75 (0.13) | 0.59 (0.05) | |
Phycodrys rubens | 24.9 (3.7) | 13.8 (0.1)* | 2.19 (0.49) | 1.57 (0.48) | 2.79 (0.83) | 2.33 (0.92) | |
Polysiphonia arctica | 11.6 (0.0) | 7.3 (0.1)* | 2.42 (0.61) | 5.37 (1.60)* | 2.69 (0.22) | 1.69 (0.22)* | |
Ptilota plumosa | 14.0 (0.7) | 10.8 (2.2) | 4.18 (0.54) | 3.92 (0.35) | 2.34 (0.35) | 1.59 (0.21)* | |
Rhodomela lycopodioides | 17.2 (1.6) | 6.4 (1.4)* | 2.19 (0.53) | 3.14 (0.59) | 2.67 (0.49) | 2.58 (0.32) | |
Phaeophyta | Alaria esculenta | 26.8 (1.3) | 14.5 (1.1)* | 2.34 (0.49) | 11.01 (2.35)* | 1.30 (0.73) | 0.57 (0.23) |
Chorda tomentosa | 32.0 (5.2) | 27.6 (3.9) | 1.53 (0.04) | 0.74 (0.02)* | 0.22 (0.02) | 0.43 (0.02)* | |
Chordaria flagelliformis | 14.7 (0.9) | 13.7 (1.3) | 1.03 (0.39) | 3.35 (1.06)* | 0.31 (0.06) | 0.34 (0.02) | |
Desmarestia aculeata | 18.8 (2.19) | 7.8 (1.3)* | 2.27 (0.28) | 1.97 (0.44) | 0.22 (0.02) | 0.43 (0.02)* | |
Fucus distichus | 8.4 (3.1) | 8.8 (1.2) | 1.22 (0.50) | 4.53 (0.35)* | 1.35 (0.27) | 0.74 (0.03)* | |
Laminaria saccharina | 23.6 (0.8) | 9.5 (1.4)* | 2.20 (0.38) | 2.35 (0.32) | 0.15 (0.03) | 0.21 (0.05) | |
Laminaria solidungula | 21.8 (0.6) | 8.0 (0.9)* | 3.44 (1.70) | 11.94 (1.17)* | 0.14 (0.01) | 0.08 (0.01)* | |
Scytosiphon lomentaria | 14.4 (2.4) | 15.3 (3.9) | 1.10 (0.32) | 1.66 (0.47) | 0.53 (0.05) | 0.46 (0.07) | |
Sphacelaria plumosa | 25.1 (0.6) | 24.7 (0.1) | 2.45 (0.06) | 3.53 (0.35) | 2.45 (0.32) | 3.20 (0.20)* |
Standard deviation in brackets (n=3–5); significant differences indicated by an asterisk (P <0.05).
Activity of the assimilating enzymes external carbonic anhydrase (CAext, REA g−1 FW), nitrate reductase (NRA, μmol g−1 FW h−1), and alkaline phosphatase (APA, μmol PNP g−1 FW h−1)
Phylum | Name | CAext | NRA | APA | |||
Control | Enriched | Control | Enriched | Control | Enriched | ||
Chlorophyta | Acrosiphonia sp. | 17.2 (1.4) | 12.3 (0.2)* | 2.67 (0.48) | 3.81 (0.91) | 0.39 (0.02) | 2.57 (0.32)* |
Chaetomorpha melagonium | 9.7 (2.3) | 15.8 (6.0) | 5.79 (0.80) | 3.97 (0.55)* | 0.29 (0.03) | 0.29 (0.03) | |
Monostroma arcticum | 33.4 (0.7) | 32.2 (2.3) | 5.16 (0.96) | 11.95 (1.43)* | 0.47 (0.25) | 0.32 (0.06) | |
Prasiola crispa | 59.0 (0.8) | 46.6 (3.3)* | 8.30 (0.47) | 8.94 (0.83) | 0.49 (0.06) | 0.89 (0.08)* | |
Rhodophyta | Ceramium strictum | 15.4 (0.8) | 16.4 (1.2) | 3.54 (0.39) | 4.95 (0.66)* | 1.45 (0.05) | 1.88 (0.05)* |
Devaleraea ramentacea | 3.6 (1.4) | 4.0 (0.4) | 1.16 (0.64) | 2.92 (0.50)* | 1.14 (0.16) | 0.79 (0.08)* | |
Odonthalia dentata | 11.9 (3.2) | 7.4 (2.3) | 8.48 (0.51) | 10.14 (0.31)* | 2.76 (0.40) | 2.06 (0.46) | |
Palmaria palmata | 3.9 (1.5) | 3.1 (1.4) | 1.48 (0.31) | 2.70 (1.11) | 0.75 (0.13) | 0.59 (0.05) | |
Phycodrys rubens | 24.9 (3.7) | 13.8 (0.1)* | 2.19 (0.49) | 1.57 (0.48) | 2.79 (0.83) | 2.33 (0.92) | |
Polysiphonia arctica | 11.6 (0.0) | 7.3 (0.1)* | 2.42 (0.61) | 5.37 (1.60)* | 2.69 (0.22) | 1.69 (0.22)* | |
Ptilota plumosa | 14.0 (0.7) | 10.8 (2.2) | 4.18 (0.54) | 3.92 (0.35) | 2.34 (0.35) | 1.59 (0.21)* | |
Rhodomela lycopodioides | 17.2 (1.6) | 6.4 (1.4)* | 2.19 (0.53) | 3.14 (0.59) | 2.67 (0.49) | 2.58 (0.32) | |
Phaeophyta | Alaria esculenta | 26.8 (1.3) | 14.5 (1.1)* | 2.34 (0.49) | 11.01 (2.35)* | 1.30 (0.73) | 0.57 (0.23) |
Chorda tomentosa | 32.0 (5.2) | 27.6 (3.9) | 1.53 (0.04) | 0.74 (0.02)* | 0.22 (0.02) | 0.43 (0.02)* | |
Chordaria flagelliformis | 14.7 (0.9) | 13.7 (1.3) | 1.03 (0.39) | 3.35 (1.06)* | 0.31 (0.06) | 0.34 (0.02) | |
Desmarestia aculeata | 18.8 (2.19) | 7.8 (1.3)* | 2.27 (0.28) | 1.97 (0.44) | 0.22 (0.02) | 0.43 (0.02)* | |
Fucus distichus | 8.4 (3.1) | 8.8 (1.2) | 1.22 (0.50) | 4.53 (0.35)* | 1.35 (0.27) | 0.74 (0.03)* | |
Laminaria saccharina | 23.6 (0.8) | 9.5 (1.4)* | 2.20 (0.38) | 2.35 (0.32) | 0.15 (0.03) | 0.21 (0.05) | |
Laminaria solidungula | 21.8 (0.6) | 8.0 (0.9)* | 3.44 (1.70) | 11.94 (1.17)* | 0.14 (0.01) | 0.08 (0.01)* | |
Scytosiphon lomentaria | 14.4 (2.4) | 15.3 (3.9) | 1.10 (0.32) | 1.66 (0.47) | 0.53 (0.05) | 0.46 (0.07) | |
Sphacelaria plumosa | 25.1 (0.6) | 24.7 (0.1) | 2.45 (0.06) | 3.53 (0.35) | 2.45 (0.32) | 3.20 (0.20)* |
Phylum | Name | CAext | NRA | APA | |||
Control | Enriched | Control | Enriched | Control | Enriched | ||
Chlorophyta | Acrosiphonia sp. | 17.2 (1.4) | 12.3 (0.2)* | 2.67 (0.48) | 3.81 (0.91) | 0.39 (0.02) | 2.57 (0.32)* |
Chaetomorpha melagonium | 9.7 (2.3) | 15.8 (6.0) | 5.79 (0.80) | 3.97 (0.55)* | 0.29 (0.03) | 0.29 (0.03) | |
Monostroma arcticum | 33.4 (0.7) | 32.2 (2.3) | 5.16 (0.96) | 11.95 (1.43)* | 0.47 (0.25) | 0.32 (0.06) | |
Prasiola crispa | 59.0 (0.8) | 46.6 (3.3)* | 8.30 (0.47) | 8.94 (0.83) | 0.49 (0.06) | 0.89 (0.08)* | |
Rhodophyta | Ceramium strictum | 15.4 (0.8) | 16.4 (1.2) | 3.54 (0.39) | 4.95 (0.66)* | 1.45 (0.05) | 1.88 (0.05)* |
Devaleraea ramentacea | 3.6 (1.4) | 4.0 (0.4) | 1.16 (0.64) | 2.92 (0.50)* | 1.14 (0.16) | 0.79 (0.08)* | |
Odonthalia dentata | 11.9 (3.2) | 7.4 (2.3) | 8.48 (0.51) | 10.14 (0.31)* | 2.76 (0.40) | 2.06 (0.46) | |
Palmaria palmata | 3.9 (1.5) | 3.1 (1.4) | 1.48 (0.31) | 2.70 (1.11) | 0.75 (0.13) | 0.59 (0.05) | |
Phycodrys rubens | 24.9 (3.7) | 13.8 (0.1)* | 2.19 (0.49) | 1.57 (0.48) | 2.79 (0.83) | 2.33 (0.92) | |
Polysiphonia arctica | 11.6 (0.0) | 7.3 (0.1)* | 2.42 (0.61) | 5.37 (1.60)* | 2.69 (0.22) | 1.69 (0.22)* | |
Ptilota plumosa | 14.0 (0.7) | 10.8 (2.2) | 4.18 (0.54) | 3.92 (0.35) | 2.34 (0.35) | 1.59 (0.21)* | |
Rhodomela lycopodioides | 17.2 (1.6) | 6.4 (1.4)* | 2.19 (0.53) | 3.14 (0.59) | 2.67 (0.49) | 2.58 (0.32) | |
Phaeophyta | Alaria esculenta | 26.8 (1.3) | 14.5 (1.1)* | 2.34 (0.49) | 11.01 (2.35)* | 1.30 (0.73) | 0.57 (0.23) |
Chorda tomentosa | 32.0 (5.2) | 27.6 (3.9) | 1.53 (0.04) | 0.74 (0.02)* | 0.22 (0.02) | 0.43 (0.02)* | |
Chordaria flagelliformis | 14.7 (0.9) | 13.7 (1.3) | 1.03 (0.39) | 3.35 (1.06)* | 0.31 (0.06) | 0.34 (0.02) | |
Desmarestia aculeata | 18.8 (2.19) | 7.8 (1.3)* | 2.27 (0.28) | 1.97 (0.44) | 0.22 (0.02) | 0.43 (0.02)* | |
Fucus distichus | 8.4 (3.1) | 8.8 (1.2) | 1.22 (0.50) | 4.53 (0.35)* | 1.35 (0.27) | 0.74 (0.03)* | |
Laminaria saccharina | 23.6 (0.8) | 9.5 (1.4)* | 2.20 (0.38) | 2.35 (0.32) | 0.15 (0.03) | 0.21 (0.05) | |
Laminaria solidungula | 21.8 (0.6) | 8.0 (0.9)* | 3.44 (1.70) | 11.94 (1.17)* | 0.14 (0.01) | 0.08 (0.01)* | |
Scytosiphon lomentaria | 14.4 (2.4) | 15.3 (3.9) | 1.10 (0.32) | 1.66 (0.47) | 0.53 (0.05) | 0.46 (0.07) | |
Sphacelaria plumosa | 25.1 (0.6) | 24.7 (0.1) | 2.45 (0.06) | 3.53 (0.35) | 2.45 (0.32) | 3.20 (0.20)* |
Standard deviation in brackets (n=3–5); significant differences indicated by an asterisk (P <0.05).
Biomass elemental composition as total C (% DW), total N (%DW), and atomic C:N ratio, as well as internal () concentration (mmol l−1 internal water)
Phylum | Name | Biomass C | Biomass N | C:N ratio | Internal | ||||
Control | Enriched | Control | Enriched | Control | Enriched | Control | Enriched | ||
Chlorophyta | Acrosiphonia sp. | 20.1 (2.8) | 17.9 (0.3) | 2.27 (0.02) | 2.08 (0.03) | 10.3 (1.5) | 10.0 (0.0) | 27.54 (4.05) | 42.71 (7.58)* |
Chaetomorpha melagonium | 29.7 (0.2) | 32.9 (0.9)* | 3.54 (0.10) | 3.65 (0.72) | 9.8 (0.3) | 10.5 (2.1) | 80.77 (8.14) | 62.80 (9.80) | |
Monostroma arcticum | 24.8 (0.5) | 26.3 (0.1)* | 1.49 (0.04) | 2.20 (0.16)* | 19.4 (0.2) | 14.5 (0.9)* | 3.31 (0.30) | 13.79 (0.59)* | |
Prasiola crispa | 30.6 (0.6) | 31.7 (1.6) | 3.09 (1.70) | 4.73 (0.15) | 8.3 (0.1) | 7.8 (0.1)* | 3.73 (1.05) | 3.11 (0.52) | |
Rhodophyta | Ceramium strictum | 25.5 (3.5) | 28.8 (1.3) | 3.00 (0.00) | 2.92 (0.82) | 9.9 (1.4) | 9.6 (1.1) | 26.06 (0.85) | 11.96 (1.72)* |
Devaleraea ramentacea | 25.5 (4.3) | 33.8 (0.3)* | 2.66 (0.12) | 2.57 (0.52) | 11.1 (1.4) | 15.7 (3.4) | 53.41 (3.10) | 41.11 (3.40)* | |
Odonthalia dentata | 35.3 (1.5) | 31.0 (3.3) | 2.49 (0.22) | 2.88 (0.62) | 16.6 (0.8) | 11.3 (3.2) | 11.27 (0.05) | 9.73 (0.17)* | |
Palmaria palmata | 27.5 (3.0) | 32.5 (2.0) | 1.82 (0.37) | 1.61 (0.30) | 17.8 (1.7) | 23.8 (3.0)* | 7.80 (2.12) | 2.83 (0.02)* | |
Phycodrys rubens | 30.1 (2.1) | 26.3 (0.6)* | 3.40 (0.05) | 3.33 (0.52) | 10.3 (0.9) | 9.4 (1.7) | 11.66 (0.30) | 14.73 (1.81)* | |
Polysiphonia arctica | 12.0 (0.5) | 30.5 (2.5)* | 1.31 (0.12) | 2.98 (0.38)* | 10.8 (1.4) | 12.0 (0.5) | 1.92 (0.62) | 1.47 (0.24) | |
Ptilota plumosa | 24.5 (0.6) | 27.9 (2.5) | 2.49 (0.18) | 3.35 (0.42)* | 11.5 (0.5) | 11.0 (0.9) | 6.78 (0.61) | 3.11 (1.74)* | |
Rhodomela lycopodioides | 27.0 (0.7) | 20.1 (3.3)* | 1.95 (1.16) | 2.28 (0.13) | 11.4 (0.7) | 10.3 (1.1) | 1.13 (0.06) | 1.35 (0.07)* | |
Phaeophyta | Alaria esculenta | 34.6 (2.3) | 32.9 (1.1) | 1.76 (0.07) | 1.25 (0.07)* | 23.0 (0.6) | 30.6 (0.7)* | 1.93 (0.38) | 1.96 (0.21) |
Chorda tomentosa | 16.0 (1.1) | 17.3 (0.5) | 1.46 (0.06) | 1.39 (0.05) | 12.8 (0.1) | 14.6 (0.1)* | 0.99 (0.03) | 0.91 (0.24) | |
Chordaria flagelliformis | 26.5 (4.6) | 22.2 (3.9) | 1.76 (0.33) | 1.70 (0.32) | 17.6 (0.2) | 15.3 (0.2)* | 2.86 (0.36) | 6.86 (0.24)* | |
Desmarestia aculeata | 37.2 (2.8) | 36.0 (1.0) | 2.29 (0.15) | 2.36 (0.02) | 19.0 (0.2) | 17.8 (0.3)* | 1.87 (0.39) | 1.58 (0.05) | |
Fucus distichus | 31.3 (2.5) | 31.0 (3.3) | 2.18 (0.86) | 1.70 (0.08) | 18.5 (8.6) | 21.3 (3.3) | 7.15 (1.04) | 1.58 (0.20)* | |
Laminaria saccharina | 26.2 (1.5) | 24.0 (2.6) | 1.25 (0.07) | 0.65 (0.02)* | 24.5 (0.1) | 42.9 (6.1)* | 2.85 (1.42) | 1.77 (0.35) | |
Laminaria solidungula | 25.4 (1.4) | 30.2 (2.4)* | 1.46 (0.22) | 1.48 (0.20) | 20.5 (0.9) | 24.2 (1.2)* | 2.70 (1.30) | 1.03 (0.28) | |
Scytosiphon lomentaria | 10.5 (1.6) | 13.1 (4.4) | 0.88 (0.12) | 1.06 (0.36) | 13.9 (0.2) | 14.4 (0.1) | 2.74 (0.25) | 3.14 (0.27) | |
Sphacelaria plumosa | 25.6 (2.7) | 29.6 (0.4)* | 2.89 (1.14) | 2.16 (0.33) | 14.3 (1.3) | 16.2 (2.7) | 4.54 (0.43) | 4.03 (0.28) |
Phylum | Name | Biomass C | Biomass N | C:N ratio | Internal | ||||
Control | Enriched | Control | Enriched | Control | Enriched | Control | Enriched | ||
Chlorophyta | Acrosiphonia sp. | 20.1 (2.8) | 17.9 (0.3) | 2.27 (0.02) | 2.08 (0.03) | 10.3 (1.5) | 10.0 (0.0) | 27.54 (4.05) | 42.71 (7.58)* |
Chaetomorpha melagonium | 29.7 (0.2) | 32.9 (0.9)* | 3.54 (0.10) | 3.65 (0.72) | 9.8 (0.3) | 10.5 (2.1) | 80.77 (8.14) | 62.80 (9.80) | |
Monostroma arcticum | 24.8 (0.5) | 26.3 (0.1)* | 1.49 (0.04) | 2.20 (0.16)* | 19.4 (0.2) | 14.5 (0.9)* | 3.31 (0.30) | 13.79 (0.59)* | |
Prasiola crispa | 30.6 (0.6) | 31.7 (1.6) | 3.09 (1.70) | 4.73 (0.15) | 8.3 (0.1) | 7.8 (0.1)* | 3.73 (1.05) | 3.11 (0.52) | |
Rhodophyta | Ceramium strictum | 25.5 (3.5) | 28.8 (1.3) | 3.00 (0.00) | 2.92 (0.82) | 9.9 (1.4) | 9.6 (1.1) | 26.06 (0.85) | 11.96 (1.72)* |
Devaleraea ramentacea | 25.5 (4.3) | 33.8 (0.3)* | 2.66 (0.12) | 2.57 (0.52) | 11.1 (1.4) | 15.7 (3.4) | 53.41 (3.10) | 41.11 (3.40)* | |
Odonthalia dentata | 35.3 (1.5) | 31.0 (3.3) | 2.49 (0.22) | 2.88 (0.62) | 16.6 (0.8) | 11.3 (3.2) | 11.27 (0.05) | 9.73 (0.17)* | |
Palmaria palmata | 27.5 (3.0) | 32.5 (2.0) | 1.82 (0.37) | 1.61 (0.30) | 17.8 (1.7) | 23.8 (3.0)* | 7.80 (2.12) | 2.83 (0.02)* | |
Phycodrys rubens | 30.1 (2.1) | 26.3 (0.6)* | 3.40 (0.05) | 3.33 (0.52) | 10.3 (0.9) | 9.4 (1.7) | 11.66 (0.30) | 14.73 (1.81)* | |
Polysiphonia arctica | 12.0 (0.5) | 30.5 (2.5)* | 1.31 (0.12) | 2.98 (0.38)* | 10.8 (1.4) | 12.0 (0.5) | 1.92 (0.62) | 1.47 (0.24) | |
Ptilota plumosa | 24.5 (0.6) | 27.9 (2.5) | 2.49 (0.18) | 3.35 (0.42)* | 11.5 (0.5) | 11.0 (0.9) | 6.78 (0.61) | 3.11 (1.74)* | |
Rhodomela lycopodioides | 27.0 (0.7) | 20.1 (3.3)* | 1.95 (1.16) | 2.28 (0.13) | 11.4 (0.7) | 10.3 (1.1) | 1.13 (0.06) | 1.35 (0.07)* | |
Phaeophyta | Alaria esculenta | 34.6 (2.3) | 32.9 (1.1) | 1.76 (0.07) | 1.25 (0.07)* | 23.0 (0.6) | 30.6 (0.7)* | 1.93 (0.38) | 1.96 (0.21) |
Chorda tomentosa | 16.0 (1.1) | 17.3 (0.5) | 1.46 (0.06) | 1.39 (0.05) | 12.8 (0.1) | 14.6 (0.1)* | 0.99 (0.03) | 0.91 (0.24) | |
Chordaria flagelliformis | 26.5 (4.6) | 22.2 (3.9) | 1.76 (0.33) | 1.70 (0.32) | 17.6 (0.2) | 15.3 (0.2)* | 2.86 (0.36) | 6.86 (0.24)* | |
Desmarestia aculeata | 37.2 (2.8) | 36.0 (1.0) | 2.29 (0.15) | 2.36 (0.02) | 19.0 (0.2) | 17.8 (0.3)* | 1.87 (0.39) | 1.58 (0.05) | |
Fucus distichus | 31.3 (2.5) | 31.0 (3.3) | 2.18 (0.86) | 1.70 (0.08) | 18.5 (8.6) | 21.3 (3.3) | 7.15 (1.04) | 1.58 (0.20)* | |
Laminaria saccharina | 26.2 (1.5) | 24.0 (2.6) | 1.25 (0.07) | 0.65 (0.02)* | 24.5 (0.1) | 42.9 (6.1)* | 2.85 (1.42) | 1.77 (0.35) | |
Laminaria solidungula | 25.4 (1.4) | 30.2 (2.4)* | 1.46 (0.22) | 1.48 (0.20) | 20.5 (0.9) | 24.2 (1.2)* | 2.70 (1.30) | 1.03 (0.28) | |
Scytosiphon lomentaria | 10.5 (1.6) | 13.1 (4.4) | 0.88 (0.12) | 1.06 (0.36) | 13.9 (0.2) | 14.4 (0.1) | 2.74 (0.25) | 3.14 (0.27) | |
Sphacelaria plumosa | 25.6 (2.7) | 29.6 (0.4)* | 2.89 (1.14) | 2.16 (0.33) | 14.3 (1.3) | 16.2 (2.7) | 4.54 (0.43) | 4.03 (0.28) |
Standard deviation in brackets (n=2–5); significant differences indicated by an asterisk (P <0.05).
Biomass elemental composition as total C (% DW), total N (%DW), and atomic C:N ratio, as well as internal () concentration (mmol l−1 internal water)
Phylum | Name | Biomass C | Biomass N | C:N ratio | Internal | ||||
Control | Enriched | Control | Enriched | Control | Enriched | Control | Enriched | ||
Chlorophyta | Acrosiphonia sp. | 20.1 (2.8) | 17.9 (0.3) | 2.27 (0.02) | 2.08 (0.03) | 10.3 (1.5) | 10.0 (0.0) | 27.54 (4.05) | 42.71 (7.58)* |
Chaetomorpha melagonium | 29.7 (0.2) | 32.9 (0.9)* | 3.54 (0.10) | 3.65 (0.72) | 9.8 (0.3) | 10.5 (2.1) | 80.77 (8.14) | 62.80 (9.80) | |
Monostroma arcticum | 24.8 (0.5) | 26.3 (0.1)* | 1.49 (0.04) | 2.20 (0.16)* | 19.4 (0.2) | 14.5 (0.9)* | 3.31 (0.30) | 13.79 (0.59)* | |
Prasiola crispa | 30.6 (0.6) | 31.7 (1.6) | 3.09 (1.70) | 4.73 (0.15) | 8.3 (0.1) | 7.8 (0.1)* | 3.73 (1.05) | 3.11 (0.52) | |
Rhodophyta | Ceramium strictum | 25.5 (3.5) | 28.8 (1.3) | 3.00 (0.00) | 2.92 (0.82) | 9.9 (1.4) | 9.6 (1.1) | 26.06 (0.85) | 11.96 (1.72)* |
Devaleraea ramentacea | 25.5 (4.3) | 33.8 (0.3)* | 2.66 (0.12) | 2.57 (0.52) | 11.1 (1.4) | 15.7 (3.4) | 53.41 (3.10) | 41.11 (3.40)* | |
Odonthalia dentata | 35.3 (1.5) | 31.0 (3.3) | 2.49 (0.22) | 2.88 (0.62) | 16.6 (0.8) | 11.3 (3.2) | 11.27 (0.05) | 9.73 (0.17)* | |
Palmaria palmata | 27.5 (3.0) | 32.5 (2.0) | 1.82 (0.37) | 1.61 (0.30) | 17.8 (1.7) | 23.8 (3.0)* | 7.80 (2.12) | 2.83 (0.02)* | |
Phycodrys rubens | 30.1 (2.1) | 26.3 (0.6)* | 3.40 (0.05) | 3.33 (0.52) | 10.3 (0.9) | 9.4 (1.7) | 11.66 (0.30) | 14.73 (1.81)* | |
Polysiphonia arctica | 12.0 (0.5) | 30.5 (2.5)* | 1.31 (0.12) | 2.98 (0.38)* | 10.8 (1.4) | 12.0 (0.5) | 1.92 (0.62) | 1.47 (0.24) | |
Ptilota plumosa | 24.5 (0.6) | 27.9 (2.5) | 2.49 (0.18) | 3.35 (0.42)* | 11.5 (0.5) | 11.0 (0.9) | 6.78 (0.61) | 3.11 (1.74)* | |
Rhodomela lycopodioides | 27.0 (0.7) | 20.1 (3.3)* | 1.95 (1.16) | 2.28 (0.13) | 11.4 (0.7) | 10.3 (1.1) | 1.13 (0.06) | 1.35 (0.07)* | |
Phaeophyta | Alaria esculenta | 34.6 (2.3) | 32.9 (1.1) | 1.76 (0.07) | 1.25 (0.07)* | 23.0 (0.6) | 30.6 (0.7)* | 1.93 (0.38) | 1.96 (0.21) |
Chorda tomentosa | 16.0 (1.1) | 17.3 (0.5) | 1.46 (0.06) | 1.39 (0.05) | 12.8 (0.1) | 14.6 (0.1)* | 0.99 (0.03) | 0.91 (0.24) | |
Chordaria flagelliformis | 26.5 (4.6) | 22.2 (3.9) | 1.76 (0.33) | 1.70 (0.32) | 17.6 (0.2) | 15.3 (0.2)* | 2.86 (0.36) | 6.86 (0.24)* | |
Desmarestia aculeata | 37.2 (2.8) | 36.0 (1.0) | 2.29 (0.15) | 2.36 (0.02) | 19.0 (0.2) | 17.8 (0.3)* | 1.87 (0.39) | 1.58 (0.05) | |
Fucus distichus | 31.3 (2.5) | 31.0 (3.3) | 2.18 (0.86) | 1.70 (0.08) | 18.5 (8.6) | 21.3 (3.3) | 7.15 (1.04) | 1.58 (0.20)* | |
Laminaria saccharina | 26.2 (1.5) | 24.0 (2.6) | 1.25 (0.07) | 0.65 (0.02)* | 24.5 (0.1) | 42.9 (6.1)* | 2.85 (1.42) | 1.77 (0.35) | |
Laminaria solidungula | 25.4 (1.4) | 30.2 (2.4)* | 1.46 (0.22) | 1.48 (0.20) | 20.5 (0.9) | 24.2 (1.2)* | 2.70 (1.30) | 1.03 (0.28) | |
Scytosiphon lomentaria | 10.5 (1.6) | 13.1 (4.4) | 0.88 (0.12) | 1.06 (0.36) | 13.9 (0.2) | 14.4 (0.1) | 2.74 (0.25) | 3.14 (0.27) | |
Sphacelaria plumosa | 25.6 (2.7) | 29.6 (0.4)* | 2.89 (1.14) | 2.16 (0.33) | 14.3 (1.3) | 16.2 (2.7) | 4.54 (0.43) | 4.03 (0.28) |
Phylum | Name | Biomass C | Biomass N | C:N ratio | Internal | ||||
Control | Enriched | Control | Enriched | Control | Enriched | Control | Enriched | ||
Chlorophyta | Acrosiphonia sp. | 20.1 (2.8) | 17.9 (0.3) | 2.27 (0.02) | 2.08 (0.03) | 10.3 (1.5) | 10.0 (0.0) | 27.54 (4.05) | 42.71 (7.58)* |
Chaetomorpha melagonium | 29.7 (0.2) | 32.9 (0.9)* | 3.54 (0.10) | 3.65 (0.72) | 9.8 (0.3) | 10.5 (2.1) | 80.77 (8.14) | 62.80 (9.80) | |
Monostroma arcticum | 24.8 (0.5) | 26.3 (0.1)* | 1.49 (0.04) | 2.20 (0.16)* | 19.4 (0.2) | 14.5 (0.9)* | 3.31 (0.30) | 13.79 (0.59)* | |
Prasiola crispa | 30.6 (0.6) | 31.7 (1.6) | 3.09 (1.70) | 4.73 (0.15) | 8.3 (0.1) | 7.8 (0.1)* | 3.73 (1.05) | 3.11 (0.52) | |
Rhodophyta | Ceramium strictum | 25.5 (3.5) | 28.8 (1.3) | 3.00 (0.00) | 2.92 (0.82) | 9.9 (1.4) | 9.6 (1.1) | 26.06 (0.85) | 11.96 (1.72)* |
Devaleraea ramentacea | 25.5 (4.3) | 33.8 (0.3)* | 2.66 (0.12) | 2.57 (0.52) | 11.1 (1.4) | 15.7 (3.4) | 53.41 (3.10) | 41.11 (3.40)* | |
Odonthalia dentata | 35.3 (1.5) | 31.0 (3.3) | 2.49 (0.22) | 2.88 (0.62) | 16.6 (0.8) | 11.3 (3.2) | 11.27 (0.05) | 9.73 (0.17)* | |
Palmaria palmata | 27.5 (3.0) | 32.5 (2.0) | 1.82 (0.37) | 1.61 (0.30) | 17.8 (1.7) | 23.8 (3.0)* | 7.80 (2.12) | 2.83 (0.02)* | |
Phycodrys rubens | 30.1 (2.1) | 26.3 (0.6)* | 3.40 (0.05) | 3.33 (0.52) | 10.3 (0.9) | 9.4 (1.7) | 11.66 (0.30) | 14.73 (1.81)* | |
Polysiphonia arctica | 12.0 (0.5) | 30.5 (2.5)* | 1.31 (0.12) | 2.98 (0.38)* | 10.8 (1.4) | 12.0 (0.5) | 1.92 (0.62) | 1.47 (0.24) | |
Ptilota plumosa | 24.5 (0.6) | 27.9 (2.5) | 2.49 (0.18) | 3.35 (0.42)* | 11.5 (0.5) | 11.0 (0.9) | 6.78 (0.61) | 3.11 (1.74)* | |
Rhodomela lycopodioides | 27.0 (0.7) | 20.1 (3.3)* | 1.95 (1.16) | 2.28 (0.13) | 11.4 (0.7) | 10.3 (1.1) | 1.13 (0.06) | 1.35 (0.07)* | |
Phaeophyta | Alaria esculenta | 34.6 (2.3) | 32.9 (1.1) | 1.76 (0.07) | 1.25 (0.07)* | 23.0 (0.6) | 30.6 (0.7)* | 1.93 (0.38) | 1.96 (0.21) |
Chorda tomentosa | 16.0 (1.1) | 17.3 (0.5) | 1.46 (0.06) | 1.39 (0.05) | 12.8 (0.1) | 14.6 (0.1)* | 0.99 (0.03) | 0.91 (0.24) | |
Chordaria flagelliformis | 26.5 (4.6) | 22.2 (3.9) | 1.76 (0.33) | 1.70 (0.32) | 17.6 (0.2) | 15.3 (0.2)* | 2.86 (0.36) | 6.86 (0.24)* | |
Desmarestia aculeata | 37.2 (2.8) | 36.0 (1.0) | 2.29 (0.15) | 2.36 (0.02) | 19.0 (0.2) | 17.8 (0.3)* | 1.87 (0.39) | 1.58 (0.05) | |
Fucus distichus | 31.3 (2.5) | 31.0 (3.3) | 2.18 (0.86) | 1.70 (0.08) | 18.5 (8.6) | 21.3 (3.3) | 7.15 (1.04) | 1.58 (0.20)* | |
Laminaria saccharina | 26.2 (1.5) | 24.0 (2.6) | 1.25 (0.07) | 0.65 (0.02)* | 24.5 (0.1) | 42.9 (6.1)* | 2.85 (1.42) | 1.77 (0.35) | |
Laminaria solidungula | 25.4 (1.4) | 30.2 (2.4)* | 1.46 (0.22) | 1.48 (0.20) | 20.5 (0.9) | 24.2 (1.2)* | 2.70 (1.30) | 1.03 (0.28) | |
Scytosiphon lomentaria | 10.5 (1.6) | 13.1 (4.4) | 0.88 (0.12) | 1.06 (0.36) | 13.9 (0.2) | 14.4 (0.1) | 2.74 (0.25) | 3.14 (0.27) | |
Sphacelaria plumosa | 25.6 (2.7) | 29.6 (0.4)* | 2.89 (1.14) | 2.16 (0.33) | 14.3 (1.3) | 16.2 (2.7) | 4.54 (0.43) | 4.03 (0.28) |
Standard deviation in brackets (n=2–5); significant differences indicated by an asterisk (P <0.05).
Tissue content of soluble carbohydrates (mg Glc g−1 FW), soluble protein (mg g−1 FW) and phycoerythrin (μg g−1 FW)
Phylum | Name | Soluble carbohydrates | Soluble proteins | Phycoerythrin | |||
Control | Enriched | Control | Enriched | Control | Enriched | ||
Chlorophyta | Acrosiphonia sp. | 14.1 (3.3) | 12.5 (1.8) | 2.37 (0.54) | 0.84 (0.12)* | ||
Chaetomorpha melagonium | 25.0 (2.7) | 39.7 (2.1)* | 6.79 (0.61) | 5.91 (0.40) | |||
Monostroma arcticum | 15.0 (1.7) | 20.4 (3.9) | 1.34 (0.60) | 1.71 (0.25) | |||
Prasiola crispa | 8.1 (1.2) | 8.7 (2.1) | nd | nd | |||
Rhodophyta | Ceramium strictum | 14.0 (3.9) | 7.3 (1.4) | 3.96 (0.41) | 2.68 (0.49)* | 14 (7) | 29 (13) |
Devaleraea ramentacea | 17.6 (1.3) | 19.7 (2.5) | 9.02 (0.59) | 8.70 (0.31) | 249 (51) | 135 (47)* | |
Odonthalia dentata | 22.6 (2.4) | 18.3 (3.3) | 2.04 (0.23) | 1.97 (0.48) | 152 (59) | 180 (100) | |
Palmaria palmata | 36.1 (1.0) | 31.9 (0.1)* | 8.17 (0.46) | 9.46 (0.98) | 126 (67) | 60 (24) | |
Phycodrys rubens | 32.0 (4.0) | 33.5 (2.7) | 5.95 (0.97) | 5.11 (0.66) | 702 (325) | 427 (135) | |
Polysiphonia arctica | 13.2 (2.5) | 20.1 (0.8)* | 1.50 (0.38) | 1.61 (0.41) | 153 (65) | 60 (24) | |
Ptilota plumosa | 15.0 (4.5) | 23.6 (4.8) | 10.80 (0.04) | 7.13 (0.57)* | 92 (65) | 36 (5) | |
Rhodomela lycopodioides | 39.4 (4.4) | 28.2 (8.0) | nd | nd | nd | nd | |
Phaeophyta | Alaria esculenta | 45.7 (1.2) | 44.8 (1.3) | 2.05 (0.64) | 4.59 (0.15)* | ||
Chorda tomentosa | 6.9 (0.4) | 6.4 (0.6) | 0.52 (0.05) | 0.63 (0.19) | |||
Chordaria flagelliformis | 23.0 (2.8) | 12.7 (3.3)* | 3.98 (0.42) | 0.83 (0.02)* | |||
Desmarestia aculeata | 19.9 (0.4) | 26.0 (2.7)* | 8.24 (0.53) | 3.86 (0.20)* | |||
Fucus distichus | 31.8 (3.1) | 33.9 (2.7) | 9.07 (2.63) | 18.63 (0.67)* | |||
Laminaria saccharina | 18.7 (3.8) | 7.0 (0.7)* | 0.40 (0.01) | 0.51 (0.05)* | |||
Laminaria solidungula | 10.0 (0.4) | 8.0 (0.4)* | 1.08 (0.02) | 1.24 (0.09)* | |||
Scytosiphon lomentaria | 2.2 (0.1) | 1.5 (0.3)* | 0.35 (0.06) | 0.39 (0.13) | |||
Sphacelaria plumosa | 41.3 (8.3) | 38.7 (14.4) | 1.51 (0.37) | 0.76 (0.02)* |
Phylum | Name | Soluble carbohydrates | Soluble proteins | Phycoerythrin | |||
Control | Enriched | Control | Enriched | Control | Enriched | ||
Chlorophyta | Acrosiphonia sp. | 14.1 (3.3) | 12.5 (1.8) | 2.37 (0.54) | 0.84 (0.12)* | ||
Chaetomorpha melagonium | 25.0 (2.7) | 39.7 (2.1)* | 6.79 (0.61) | 5.91 (0.40) | |||
Monostroma arcticum | 15.0 (1.7) | 20.4 (3.9) | 1.34 (0.60) | 1.71 (0.25) | |||
Prasiola crispa | 8.1 (1.2) | 8.7 (2.1) | nd | nd | |||
Rhodophyta | Ceramium strictum | 14.0 (3.9) | 7.3 (1.4) | 3.96 (0.41) | 2.68 (0.49)* | 14 (7) | 29 (13) |
Devaleraea ramentacea | 17.6 (1.3) | 19.7 (2.5) | 9.02 (0.59) | 8.70 (0.31) | 249 (51) | 135 (47)* | |
Odonthalia dentata | 22.6 (2.4) | 18.3 (3.3) | 2.04 (0.23) | 1.97 (0.48) | 152 (59) | 180 (100) | |
Palmaria palmata | 36.1 (1.0) | 31.9 (0.1)* | 8.17 (0.46) | 9.46 (0.98) | 126 (67) | 60 (24) | |
Phycodrys rubens | 32.0 (4.0) | 33.5 (2.7) | 5.95 (0.97) | 5.11 (0.66) | 702 (325) | 427 (135) | |
Polysiphonia arctica | 13.2 (2.5) | 20.1 (0.8)* | 1.50 (0.38) | 1.61 (0.41) | 153 (65) | 60 (24) | |
Ptilota plumosa | 15.0 (4.5) | 23.6 (4.8) | 10.80 (0.04) | 7.13 (0.57)* | 92 (65) | 36 (5) | |
Rhodomela lycopodioides | 39.4 (4.4) | 28.2 (8.0) | nd | nd | nd | nd | |
Phaeophyta | Alaria esculenta | 45.7 (1.2) | 44.8 (1.3) | 2.05 (0.64) | 4.59 (0.15)* | ||
Chorda tomentosa | 6.9 (0.4) | 6.4 (0.6) | 0.52 (0.05) | 0.63 (0.19) | |||
Chordaria flagelliformis | 23.0 (2.8) | 12.7 (3.3)* | 3.98 (0.42) | 0.83 (0.02)* | |||
Desmarestia aculeata | 19.9 (0.4) | 26.0 (2.7)* | 8.24 (0.53) | 3.86 (0.20)* | |||
Fucus distichus | 31.8 (3.1) | 33.9 (2.7) | 9.07 (2.63) | 18.63 (0.67)* | |||
Laminaria saccharina | 18.7 (3.8) | 7.0 (0.7)* | 0.40 (0.01) | 0.51 (0.05)* | |||
Laminaria solidungula | 10.0 (0.4) | 8.0 (0.4)* | 1.08 (0.02) | 1.24 (0.09)* | |||
Scytosiphon lomentaria | 2.2 (0.1) | 1.5 (0.3)* | 0.35 (0.06) | 0.39 (0.13) | |||
Sphacelaria plumosa | 41.3 (8.3) | 38.7 (14.4) | 1.51 (0.37) | 0.76 (0.02)* |
Standard deviation in brackets (n=2–4); significant differences indicated by an asterisk (P <0.05). nd, not determined.
Tissue content of soluble carbohydrates (mg Glc g−1 FW), soluble protein (mg g−1 FW) and phycoerythrin (μg g−1 FW)
Phylum | Name | Soluble carbohydrates | Soluble proteins | Phycoerythrin | |||
Control | Enriched | Control | Enriched | Control | Enriched | ||
Chlorophyta | Acrosiphonia sp. | 14.1 (3.3) | 12.5 (1.8) | 2.37 (0.54) | 0.84 (0.12)* | ||
Chaetomorpha melagonium | 25.0 (2.7) | 39.7 (2.1)* | 6.79 (0.61) | 5.91 (0.40) | |||
Monostroma arcticum | 15.0 (1.7) | 20.4 (3.9) | 1.34 (0.60) | 1.71 (0.25) | |||
Prasiola crispa | 8.1 (1.2) | 8.7 (2.1) | nd | nd | |||
Rhodophyta | Ceramium strictum | 14.0 (3.9) | 7.3 (1.4) | 3.96 (0.41) | 2.68 (0.49)* | 14 (7) | 29 (13) |
Devaleraea ramentacea | 17.6 (1.3) | 19.7 (2.5) | 9.02 (0.59) | 8.70 (0.31) | 249 (51) | 135 (47)* | |
Odonthalia dentata | 22.6 (2.4) | 18.3 (3.3) | 2.04 (0.23) | 1.97 (0.48) | 152 (59) | 180 (100) | |
Palmaria palmata | 36.1 (1.0) | 31.9 (0.1)* | 8.17 (0.46) | 9.46 (0.98) | 126 (67) | 60 (24) | |
Phycodrys rubens | 32.0 (4.0) | 33.5 (2.7) | 5.95 (0.97) | 5.11 (0.66) | 702 (325) | 427 (135) | |
Polysiphonia arctica | 13.2 (2.5) | 20.1 (0.8)* | 1.50 (0.38) | 1.61 (0.41) | 153 (65) | 60 (24) | |
Ptilota plumosa | 15.0 (4.5) | 23.6 (4.8) | 10.80 (0.04) | 7.13 (0.57)* | 92 (65) | 36 (5) | |
Rhodomela lycopodioides | 39.4 (4.4) | 28.2 (8.0) | nd | nd | nd | nd | |
Phaeophyta | Alaria esculenta | 45.7 (1.2) | 44.8 (1.3) | 2.05 (0.64) | 4.59 (0.15)* | ||
Chorda tomentosa | 6.9 (0.4) | 6.4 (0.6) | 0.52 (0.05) | 0.63 (0.19) | |||
Chordaria flagelliformis | 23.0 (2.8) | 12.7 (3.3)* | 3.98 (0.42) | 0.83 (0.02)* | |||
Desmarestia aculeata | 19.9 (0.4) | 26.0 (2.7)* | 8.24 (0.53) | 3.86 (0.20)* | |||
Fucus distichus | 31.8 (3.1) | 33.9 (2.7) | 9.07 (2.63) | 18.63 (0.67)* | |||
Laminaria saccharina | 18.7 (3.8) | 7.0 (0.7)* | 0.40 (0.01) | 0.51 (0.05)* | |||
Laminaria solidungula | 10.0 (0.4) | 8.0 (0.4)* | 1.08 (0.02) | 1.24 (0.09)* | |||
Scytosiphon lomentaria | 2.2 (0.1) | 1.5 (0.3)* | 0.35 (0.06) | 0.39 (0.13) | |||
Sphacelaria plumosa | 41.3 (8.3) | 38.7 (14.4) | 1.51 (0.37) | 0.76 (0.02)* |
Phylum | Name | Soluble carbohydrates | Soluble proteins | Phycoerythrin | |||
Control | Enriched | Control | Enriched | Control | Enriched | ||
Chlorophyta | Acrosiphonia sp. | 14.1 (3.3) | 12.5 (1.8) | 2.37 (0.54) | 0.84 (0.12)* | ||
Chaetomorpha melagonium | 25.0 (2.7) | 39.7 (2.1)* | 6.79 (0.61) | 5.91 (0.40) | |||
Monostroma arcticum | 15.0 (1.7) | 20.4 (3.9) | 1.34 (0.60) | 1.71 (0.25) | |||
Prasiola crispa | 8.1 (1.2) | 8.7 (2.1) | nd | nd | |||
Rhodophyta | Ceramium strictum | 14.0 (3.9) | 7.3 (1.4) | 3.96 (0.41) | 2.68 (0.49)* | 14 (7) | 29 (13) |
Devaleraea ramentacea | 17.6 (1.3) | 19.7 (2.5) | 9.02 (0.59) | 8.70 (0.31) | 249 (51) | 135 (47)* | |
Odonthalia dentata | 22.6 (2.4) | 18.3 (3.3) | 2.04 (0.23) | 1.97 (0.48) | 152 (59) | 180 (100) | |
Palmaria palmata | 36.1 (1.0) | 31.9 (0.1)* | 8.17 (0.46) | 9.46 (0.98) | 126 (67) | 60 (24) | |
Phycodrys rubens | 32.0 (4.0) | 33.5 (2.7) | 5.95 (0.97) | 5.11 (0.66) | 702 (325) | 427 (135) | |
Polysiphonia arctica | 13.2 (2.5) | 20.1 (0.8)* | 1.50 (0.38) | 1.61 (0.41) | 153 (65) | 60 (24) | |
Ptilota plumosa | 15.0 (4.5) | 23.6 (4.8) | 10.80 (0.04) | 7.13 (0.57)* | 92 (65) | 36 (5) | |
Rhodomela lycopodioides | 39.4 (4.4) | 28.2 (8.0) | nd | nd | nd | nd | |
Phaeophyta | Alaria esculenta | 45.7 (1.2) | 44.8 (1.3) | 2.05 (0.64) | 4.59 (0.15)* | ||
Chorda tomentosa | 6.9 (0.4) | 6.4 (0.6) | 0.52 (0.05) | 0.63 (0.19) | |||
Chordaria flagelliformis | 23.0 (2.8) | 12.7 (3.3)* | 3.98 (0.42) | 0.83 (0.02)* | |||
Desmarestia aculeata | 19.9 (0.4) | 26.0 (2.7)* | 8.24 (0.53) | 3.86 (0.20)* | |||
Fucus distichus | 31.8 (3.1) | 33.9 (2.7) | 9.07 (2.63) | 18.63 (0.67)* | |||
Laminaria saccharina | 18.7 (3.8) | 7.0 (0.7)* | 0.40 (0.01) | 0.51 (0.05)* | |||
Laminaria solidungula | 10.0 (0.4) | 8.0 (0.4)* | 1.08 (0.02) | 1.24 (0.09)* | |||
Scytosiphon lomentaria | 2.2 (0.1) | 1.5 (0.3)* | 0.35 (0.06) | 0.39 (0.13) | |||
Sphacelaria plumosa | 41.3 (8.3) | 38.7 (14.4) | 1.51 (0.37) | 0.76 (0.02)* |
Standard deviation in brackets (n=2–4); significant differences indicated by an asterisk (P <0.05). nd, not determined.
Phylum | Name | Chlorophyll a | Chlorophyll b | Total carotenoids | Accessory pigments:Chl. aa | ||||
Control | Enriched | Control | Enriched | Control | Enriched | Control | Enriched | ||
Chlorophyta | Acrosiphonia sp. | 588 (75) | 912 (50)* | 478 (27) | 776 (33)* | 178 (56) | 195 (62) | 1.12 | 1.07 |
Chaetomorpha melagonium | 1920 (290) | 1354 (208)* | 1377 (217) | 1130 (47) | 520 (68) | 390 (51) | 0.99 | 1.12 | |
Monostroma arcticum | 2578 (333) | 1511 (181)* | 2000 (218) | 1261 (59)* | 737 (32) | 356 (2)* | 1.06 | 1.07 | |
Prasiola crispa | 3589 (98) | 3083 (84)* | 901 (31) | 761 (26)* | 1011 (39) | 1211 (47)* | 0.53 | 0.64 | |
Rhodophyta | Ceramium strictum | 559 (35) | 885 (56)* | 69 (3) | 152 (18)* | 0.12 | 0.17 | ||
Devaleraea ramentacea | 129 (17) | 330 (27)* | 11 (2) | 60 (12)* | 0.08 | 0.18 | |||
Odonthalia dentata | 538 (46) | 1076 (92)* | 57 (6) | 133 (13)* | 0.11 | 0.12 | |||
Palmaria palmata | 1012 (7) | 689 (5)* | 189 (7) | 135 (5)* | 0.19 | 0.20 | |||
Phycodrys rubens | 918 (22) | 1239 (45)* | 141 (38) | 200 (31) | 0.15 | 0.16 | |||
Polysiphonia arctica | 1134 (19) | 1319 (22)* | 135 (5) | 194 (5)* | 0.12 | 0.15 | |||
Ptilota plumosa | 721 (62) | 726 (13) | 82 (4) | 95 (20) | 0.11 | 0.13 | |||
Rhodomela lycopodioides | 979 (13) | 999 (24) | 193 (2) | 182 (0)* | 0.20 | 0.18 | |||
Phaeophyta | Alaria esculenta | 1399 (200) | 632 (90)* | 271 (36) | 165 (2)* | 0.19 | 0.26 | ||
Chorda tomentosa | 1338 (191) | 614 (88)* | 243 (4) | 175 (3)* | 0.18 | 0.29 | |||
Chordaria flagelliformis | 1595 (61) | 2773 (76)* | 507 (8) | 824 (86)* | 0.32 | 0.30 | |||
Desmarestia aculeata | 1190 (19) | 1340 (56)* | 348 (3) | 454 (67) | 0.29 | 0.34 | |||
Fucus distichus | 1345 (52) | 1165 (36)* | 327 (4) | 321 (15) | 0.24 | 0.28 | |||
Laminaria saccharina | 403 (106) | 543 (143) | 105 (12) | 144 (4)* | 0.26 | 0.27 | |||
Laminaria solidungula | 1072 (66) | 777 (48)* | 152 (13) | 189 (8)* | 0.14 | 0.24 | |||
Scytosiphon lomentaria | 1517 (94) | 794 (49)* | 410 (11) | 105 (7)* | 0.27 | 0.13 | |||
Sphacelaria plumosa | 1510 (268) | 1915 (8)* | 487 (13) | 728 (44)* | 0.32 | 0.38 |
Phylum | Name | Chlorophyll a | Chlorophyll b | Total carotenoids | Accessory pigments:Chl. aa | ||||
Control | Enriched | Control | Enriched | Control | Enriched | Control | Enriched | ||
Chlorophyta | Acrosiphonia sp. | 588 (75) | 912 (50)* | 478 (27) | 776 (33)* | 178 (56) | 195 (62) | 1.12 | 1.07 |
Chaetomorpha melagonium | 1920 (290) | 1354 (208)* | 1377 (217) | 1130 (47) | 520 (68) | 390 (51) | 0.99 | 1.12 | |
Monostroma arcticum | 2578 (333) | 1511 (181)* | 2000 (218) | 1261 (59)* | 737 (32) | 356 (2)* | 1.06 | 1.07 | |
Prasiola crispa | 3589 (98) | 3083 (84)* | 901 (31) | 761 (26)* | 1011 (39) | 1211 (47)* | 0.53 | 0.64 | |
Rhodophyta | Ceramium strictum | 559 (35) | 885 (56)* | 69 (3) | 152 (18)* | 0.12 | 0.17 | ||
Devaleraea ramentacea | 129 (17) | 330 (27)* | 11 (2) | 60 (12)* | 0.08 | 0.18 | |||
Odonthalia dentata | 538 (46) | 1076 (92)* | 57 (6) | 133 (13)* | 0.11 | 0.12 | |||
Palmaria palmata | 1012 (7) | 689 (5)* | 189 (7) | 135 (5)* | 0.19 | 0.20 | |||
Phycodrys rubens | 918 (22) | 1239 (45)* | 141 (38) | 200 (31) | 0.15 | 0.16 | |||
Polysiphonia arctica | 1134 (19) | 1319 (22)* | 135 (5) | 194 (5)* | 0.12 | 0.15 | |||
Ptilota plumosa | 721 (62) | 726 (13) | 82 (4) | 95 (20) | 0.11 | 0.13 | |||
Rhodomela lycopodioides | 979 (13) | 999 (24) | 193 (2) | 182 (0)* | 0.20 | 0.18 | |||
Phaeophyta | Alaria esculenta | 1399 (200) | 632 (90)* | 271 (36) | 165 (2)* | 0.19 | 0.26 | ||
Chorda tomentosa | 1338 (191) | 614 (88)* | 243 (4) | 175 (3)* | 0.18 | 0.29 | |||
Chordaria flagelliformis | 1595 (61) | 2773 (76)* | 507 (8) | 824 (86)* | 0.32 | 0.30 | |||
Desmarestia aculeata | 1190 (19) | 1340 (56)* | 348 (3) | 454 (67) | 0.29 | 0.34 | |||
Fucus distichus | 1345 (52) | 1165 (36)* | 327 (4) | 321 (15) | 0.24 | 0.28 | |||
Laminaria saccharina | 403 (106) | 543 (143) | 105 (12) | 144 (4)* | 0.26 | 0.27 | |||
Laminaria solidungula | 1072 (66) | 777 (48)* | 152 (13) | 189 (8)* | 0.14 | 0.24 | |||
Scytosiphon lomentaria | 1517 (94) | 794 (49)* | 410 (11) | 105 (7)* | 0.27 | 0.13 | |||
Sphacelaria plumosa | 1510 (268) | 1915 (8)* | 487 (13) | 728 (44)* | 0.32 | 0.38 |
Standard deviation in brackets (n=2–4), and significant differences indicated by an asterisk (P <0.05).
(Chl. b+carotenoids):Chl.a for Chlorophyta; carotenoids:Chl. a for Rhodophyta and Phaeophyta.
Phylum | Name | Chlorophyll a | Chlorophyll b | Total carotenoids | Accessory pigments:Chl. aa | ||||
Control | Enriched | Control | Enriched | Control | Enriched | Control | Enriched | ||
Chlorophyta | Acrosiphonia sp. | 588 (75) | 912 (50)* | 478 (27) | 776 (33)* | 178 (56) | 195 (62) | 1.12 | 1.07 |
Chaetomorpha melagonium | 1920 (290) | 1354 (208)* | 1377 (217) | 1130 (47) | 520 (68) | 390 (51) | 0.99 | 1.12 | |
Monostroma arcticum | 2578 (333) | 1511 (181)* | 2000 (218) | 1261 (59)* | 737 (32) | 356 (2)* | 1.06 | 1.07 | |
Prasiola crispa | 3589 (98) | 3083 (84)* | 901 (31) | 761 (26)* | 1011 (39) | 1211 (47)* | 0.53 | 0.64 | |
Rhodophyta | Ceramium strictum | 559 (35) | 885 (56)* | 69 (3) | 152 (18)* | 0.12 | 0.17 | ||
Devaleraea ramentacea | 129 (17) | 330 (27)* | 11 (2) | 60 (12)* | 0.08 | 0.18 | |||
Odonthalia dentata | 538 (46) | 1076 (92)* | 57 (6) | 133 (13)* | 0.11 | 0.12 | |||
Palmaria palmata | 1012 (7) | 689 (5)* | 189 (7) | 135 (5)* | 0.19 | 0.20 | |||
Phycodrys rubens | 918 (22) | 1239 (45)* | 141 (38) | 200 (31) | 0.15 | 0.16 | |||
Polysiphonia arctica | 1134 (19) | 1319 (22)* | 135 (5) | 194 (5)* | 0.12 | 0.15 | |||
Ptilota plumosa | 721 (62) | 726 (13) | 82 (4) | 95 (20) | 0.11 | 0.13 | |||
Rhodomela lycopodioides | 979 (13) | 999 (24) | 193 (2) | 182 (0)* | 0.20 | 0.18 | |||
Phaeophyta | Alaria esculenta | 1399 (200) | 632 (90)* | 271 (36) | 165 (2)* | 0.19 | 0.26 | ||
Chorda tomentosa | 1338 (191) | 614 (88)* | 243 (4) | 175 (3)* | 0.18 | 0.29 | |||
Chordaria flagelliformis | 1595 (61) | 2773 (76)* | 507 (8) | 824 (86)* | 0.32 | 0.30 | |||
Desmarestia aculeata | 1190 (19) | 1340 (56)* | 348 (3) | 454 (67) | 0.29 | 0.34 | |||
Fucus distichus | 1345 (52) | 1165 (36)* | 327 (4) | 321 (15) | 0.24 | 0.28 | |||
Laminaria saccharina | 403 (106) | 543 (143) | 105 (12) | 144 (4)* | 0.26 | 0.27 | |||
Laminaria solidungula | 1072 (66) | 777 (48)* | 152 (13) | 189 (8)* | 0.14 | 0.24 | |||
Scytosiphon lomentaria | 1517 (94) | 794 (49)* | 410 (11) | 105 (7)* | 0.27 | 0.13 | |||
Sphacelaria plumosa | 1510 (268) | 1915 (8)* | 487 (13) | 728 (44)* | 0.32 | 0.38 |
Phylum | Name | Chlorophyll a | Chlorophyll b | Total carotenoids | Accessory pigments:Chl. aa | ||||
Control | Enriched | Control | Enriched | Control | Enriched | Control | Enriched | ||
Chlorophyta | Acrosiphonia sp. | 588 (75) | 912 (50)* | 478 (27) | 776 (33)* | 178 (56) | 195 (62) | 1.12 | 1.07 |
Chaetomorpha melagonium | 1920 (290) | 1354 (208)* | 1377 (217) | 1130 (47) | 520 (68) | 390 (51) | 0.99 | 1.12 | |
Monostroma arcticum | 2578 (333) | 1511 (181)* | 2000 (218) | 1261 (59)* | 737 (32) | 356 (2)* | 1.06 | 1.07 | |
Prasiola crispa | 3589 (98) | 3083 (84)* | 901 (31) | 761 (26)* | 1011 (39) | 1211 (47)* | 0.53 | 0.64 | |
Rhodophyta | Ceramium strictum | 559 (35) | 885 (56)* | 69 (3) | 152 (18)* | 0.12 | 0.17 | ||
Devaleraea ramentacea | 129 (17) | 330 (27)* | 11 (2) | 60 (12)* | 0.08 | 0.18 | |||
Odonthalia dentata | 538 (46) | 1076 (92)* | 57 (6) | 133 (13)* | 0.11 | 0.12 | |||
Palmaria palmata | 1012 (7) | 689 (5)* | 189 (7) | 135 (5)* | 0.19 | 0.20 | |||
Phycodrys rubens | 918 (22) | 1239 (45)* | 141 (38) | 200 (31) | 0.15 | 0.16 | |||
Polysiphonia arctica | 1134 (19) | 1319 (22)* | 135 (5) | 194 (5)* | 0.12 | 0.15 | |||
Ptilota plumosa | 721 (62) | 726 (13) | 82 (4) | 95 (20) | 0.11 | 0.13 | |||
Rhodomela lycopodioides | 979 (13) | 999 (24) | 193 (2) | 182 (0)* | 0.20 | 0.18 | |||
Phaeophyta | Alaria esculenta | 1399 (200) | 632 (90)* | 271 (36) | 165 (2)* | 0.19 | 0.26 | ||
Chorda tomentosa | 1338 (191) | 614 (88)* | 243 (4) | 175 (3)* | 0.18 | 0.29 | |||
Chordaria flagelliformis | 1595 (61) | 2773 (76)* | 507 (8) | 824 (86)* | 0.32 | 0.30 | |||
Desmarestia aculeata | 1190 (19) | 1340 (56)* | 348 (3) | 454 (67) | 0.29 | 0.34 | |||
Fucus distichus | 1345 (52) | 1165 (36)* | 327 (4) | 321 (15) | 0.24 | 0.28 | |||
Laminaria saccharina | 403 (106) | 543 (143) | 105 (12) | 144 (4)* | 0.26 | 0.27 | |||
Laminaria solidungula | 1072 (66) | 777 (48)* | 152 (13) | 189 (8)* | 0.14 | 0.24 | |||
Scytosiphon lomentaria | 1517 (94) | 794 (49)* | 410 (11) | 105 (7)* | 0.27 | 0.13 | |||
Sphacelaria plumosa | 1510 (268) | 1915 (8)* | 487 (13) | 728 (44)* | 0.32 | 0.38 |
Standard deviation in brackets (n=2–4), and significant differences indicated by an asterisk (P <0.05).
(Chl. b+carotenoids):Chl.a for Chlorophyta; carotenoids:Chl. a for Rhodophyta and Phaeophyta.
Results
The experiments were carried out during the Arctic summer month of July. At that time of the year both ambient nitrate and phosphate concentrations were close to the detection limit (0.4 μM nitrate and 0.5 μM SRP). Ammonium levels were below detection limits (<0.1 μM). CO2 concentration in the seawater from Kongsfjord was calculated by using the CO2sys program (Lewis and Wallace, 1998). The calculated values were 110 μM , 2067 μM , and 20 μM CO2. Under these chemical conditions, all the 21 species examined showed significant CAext activity (Table 2). On average, green algae showed the highest activity, followed by the brown algae, and the red algae, respectively. The lowest values were obtained in the red algae P. palmata and D. ramentacea (3.1 and 3.6 REA g−1 FW, respectively) while the maximum was found in the green alga P. crispa (59 REA g−1 FW). Nine out of the 21 species studied (two green, three red, and four brown) significantly decreased their CAext activity when incubated in nutrient-enriched seawater, so that decreased CAext cannot be ascribed to any particular group (Table 6). None of the species tested showed a significant increase. This effect in CAext was accompanied by a general increase in NR activity (NRA, Tables 2, 6). Nitrate reductase activity ranged from 0.7 to 12 μmol g−1 FW h−1. Under control conditions (non-enriched), the highest values corresponded in general to green algae, and the lowest to brown algae; however, N enriched kelps A. esculenta and L. solidungula, showed the overall highest values (above 11 μmol g−1 FW h−1) together with the green M. arcticum. Twelve out of the 21 species examined significantly increased NRA under nutrient enrichment, while only Chorda tomentosa significantly decreased (Table 2). The highest percentage of induction was found in M. arcticum, D. ramentacea, P. arctica, A. esculenta, C. flagelliformis, F. distichus, and L. solidungula with NRA values more than double the values registered in non-enriched conditions. The results for APA, showed a significant increase in six species and a significant decrease in five species (Table 2). Values ranged from 0.08 to 3.20 μmol pNP g−1 FW h−1, the red algae showing highest activity.
Statistical significance of differences produced by nutrient enrichment in each variable measured (after standardization)
Chlorophyte | Rhodophyte | Phaeophyte | All | |
CAext | – | *↓ | *↓ | *↓ |
NRA | – | *↑ | *↑ | *↑ |
APA | – | – | – | – |
Total internal C | – | – | – | – |
Total internal N | – | – | – | – |
Internal C:N atomic ratio | – | – | *↑ | – |
Internal | – | *↓ | – | – |
Soluble carbohydrates | – | – | *↓ | – |
Soluble proteins | – | – | – | – |
Phycoerithrin | *↓ | |||
Chlorophyll a | – | *↑ | – | – |
Chlorophyll b | – | |||
Total carotenoids | – | *↑ | – | – |
Accessory pigments:Chlorophyll a | – | – | *↑ | *↑ |
Chlorophyte | Rhodophyte | Phaeophyte | All | |
CAext | – | *↓ | *↓ | *↓ |
NRA | – | *↑ | *↑ | *↑ |
APA | – | – | – | – |
Total internal C | – | – | – | – |
Total internal N | – | – | – | – |
Internal C:N atomic ratio | – | – | *↑ | – |
Internal | – | *↓ | – | – |
Soluble carbohydrates | – | – | *↓ | – |
Soluble proteins | – | – | – | – |
Phycoerithrin | *↓ | |||
Chlorophyll a | – | *↑ | – | – |
Chlorophyll b | – | |||
Total carotenoids | – | *↑ | – | – |
Accessory pigments:Chlorophyll a | – | – | *↑ | *↑ |
Species were grouped by phylum or considered altogether. Asterisk, significant (P <0.05); (–), not significant for Tukey's tests (phylum-grouped species), or two-way ANOVA tests (all species together). The direction of significant changes are indicated by arrows (↑, higher in enriched medium; ↓, lower in enriched medium).
Statistical significance of differences produced by nutrient enrichment in each variable measured (after standardization)
Chlorophyte | Rhodophyte | Phaeophyte | All | |
CAext | – | *↓ | *↓ | *↓ |
NRA | – | *↑ | *↑ | *↑ |
APA | – | – | – | – |
Total internal C | – | – | – | – |
Total internal N | – | – | – | – |
Internal C:N atomic ratio | – | – | *↑ | – |
Internal | – | *↓ | – | – |
Soluble carbohydrates | – | – | *↓ | – |
Soluble proteins | – | – | – | – |
Phycoerithrin | *↓ | |||
Chlorophyll a | – | *↑ | – | – |
Chlorophyll b | – | |||
Total carotenoids | – | *↑ | – | – |
Accessory pigments:Chlorophyll a | – | – | *↑ | *↑ |
Chlorophyte | Rhodophyte | Phaeophyte | All | |
CAext | – | *↓ | *↓ | *↓ |
NRA | – | *↑ | *↑ | *↑ |
APA | – | – | – | – |
Total internal C | – | – | – | – |
Total internal N | – | – | – | – |
Internal C:N atomic ratio | – | – | *↑ | – |
Internal | – | *↓ | – | – |
Soluble carbohydrates | – | – | *↓ | – |
Soluble proteins | – | – | – | – |
Phycoerithrin | *↓ | |||
Chlorophyll a | – | *↑ | – | – |
Chlorophyll b | – | |||
Total carotenoids | – | *↑ | – | – |
Accessory pigments:Chlorophyll a | – | – | *↑ | *↑ |
Species were grouped by phylum or considered altogether. Asterisk, significant (P <0.05); (–), not significant for Tukey's tests (phylum-grouped species), or two-way ANOVA tests (all species together). The direction of significant changes are indicated by arrows (↑, higher in enriched medium; ↓, lower in enriched medium).
Values for total internal C (Table 3) were similar for the three algal groups, averaging 26% DW. The lowest values were measured in the red P. arctica (12% DW), and the brown C. tomentosa (16% DW) and S. lomentaria (10.5% DW) for control conditions; while the highest corresponded to the red O. dentata (35% DW) and the brown A. esculenta (35% DW) and D. aculeata (37% DW), also for control conditions. Nutrient enrichment did promote some significant but discrete changes in general terms, with six species increasing and two species decreasing. Values under enriched conditions ranged from 13% DW in S. lomentaria to 36% DW in D. aculeata. When considered by phylum, no significant changes were recorded for any group (Table 6). Total internal N averaged 2.2% DW under control conditions and 2.3 under enriched conditions. Significant changes were only detected in five out of the 21 species studied. Total N content was slightly lower in brown algae (1.8% and 1.5% DW on average) than in green (2.6% and 3.2% DW on average) and red algae (2.4% and 2.7% DW on average), in control and enriched conditions, respectively. When considered by phylum, no significant changes were recorded for any group (Table 6). Changes in internal C and N were further evaluated by calculating the C:N ratio (Table 3). Values ranged from 8 in the green P. crispa to 42 in nutrient-enriched L. saccharina. The average group value was higher in brown (18 and 22) than in green (12 and 11) and in red (12 and 13) algae for control and enriched conditions, respectively. No general pattern of significant change after nutrient enrichment was found, with five species showing higher C:N values and four species showing lower values with respect to the control. The Laminariales were a special case. The three Laminariales species examined showed increased C:N values, resulting in the significant increase observed when considering the whole brown algae group (Table 6).
Nitrogen in the cell may be present as part of the biomass (organic) or stored in inorganic form as an internal reservoir for later use. Table 3 shows the concentrations for inorganic N (nitrate+nitrite) measured in the water contained in the thalli (the latter being the difference between FW and DW; Table 1). Stored inorganic N ranged from 1 mM in the brown C. tomentosa and enriched L. solidungula to 81 mM in the green C. melagonium. If winter inorganic N had been 10, that corresponded to accumulation factors from ×100 to ×8100 (assuming external concentration to be 10 μM inorganic N). On average, green algae showed the highest capacity to store inorganic N (29 mM) compared with red (15 mM) and brown (3 mM). In N-enriched thalli, averaged differences between groups were slightly higher (30 mM in green, 11 mM in red, and 2.5 mM in brown algae). Only five species increased the stored amount of inorganic N after nutrient enrichment, the green Acrosiphonia sp. and M. arcticum, the red P. rubens, and R. lycopodioides, and the brown C. flageliformis, while up to seven species showed significant reduction in their internal pool of inorganic N.
Soluble carbohydrate ranged from 1.5 to 45.7 mg Glc g−1 FW, both values being recorded in brown algae (Table 4). Nutrient enrichment promoted a different pattern for each group. Soluble carbohydrates increased in green algae (22% on average), changed little in red algae, and decreased in brown algae (15% on average). Soluble proteins ranged from 0.35 to 18 mg g−1 FW, the lowest values corresponding to brown algae, and the highest to red algae and the brown F. distichus and D. aculeata. Although by groups, changes in soluble protein were not significant (Table 6), four species showed increased protein content, including the fucoid and the three laminarians, while six others showed a significant decrease (Table 4). The phycobiliproteins phycoerythrin (Table 4), and phycocyanin (not shown) were measured in red algae. Phycoerythrin ranged from 14 to 700 μg g−1 FW, but no significant variations could be ascribed to nutrient enrichment.
Chlorophyll a content was highest in green algae (1942 μg g−1 FW on average) and lowest in red algae (828 μg g−1 FW on average; Table 5). Chlorophyll a was highly sensitive to nutrient enrichment, which promoted significant variations in 18 out of the 21 species studied. However, there was no general pattern, with nine species increasing and nine species decreasing their chlorophyll a content. The most consistent trend was found in red algae with an average increase of 38% (Table 6). Chlorophyll b in green algae showed no specific trend, with two out of four decreasing their values after nutrient enrichment (Table 5). Total carotenoids were highly variable, ranging from 11 μg g−1 FW in the red D. ramentacea to more than 1200 μg g−1 FW in the green P. crispa. Similarly to chlorophyll a, total carotenoids significantly varied after nutrient enrichment in most species (Table 5), but no general trend was found, with nine species showing an increase and six a decrease. The most regular pattern appeared again in red algae (Table 6) with a significant increase of 30% (on average). The ratio of accessory pigments to chlorophyll a was calculated and is shown in Table 5. Slight increases were recorded for all three groups, but it was significant only for brown algae (Table 6).
Figure 1 shows the results of the principal components analyses (PCAs) performed on data for non-enriched (upper panel) and enriched thalli (lower panel). In the analysis for non-enriched thalli, the three algal phyla were clearly separated in non-overlapping regions of the two-dimensional space formed by axis I and axis II. The separation of each phylum generated mainly in axis I, while axis II (and axis I to a lesser extent) served as a discriminant between species of the same phylum. Axis I was mainly defined by CAext activity, chlorophyll a, and total carotenoids; NRA, soluble carbohydrates and proteins, and the ratio accessory pigments:chlorophyll a were significant but had lower loading factors (Table 7). Axis II was most influenced by variables directly related to nitrogen; the main component was internal N followed by stored nitrate, the C:N ratio, and NRA. Both axes together explained 54% of the total variance of data.
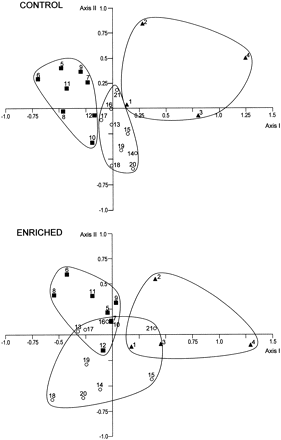
Principal components analyses performed using the 12 variables measured in all the 21 species studied, for both non-enriched (Control, upper panel) and nutrient-enriched thalli (lower panel). Species numbered as in Table 1. Curved lines were arbitrarily drawn to delimit regions for each phylum. Loading factors and percentage of variance for each axis are shown in Table 7. (filled squares) Chlorophytes; (open circles) Rhodophytes; (filled triangles) Phaeophytes.
Loading factors for each variable of principal components analyses shown in Fig. 1
Control | Enriched | |||
Axis I | Axis II | Axis I | Axis II | |
CAext | 0.414* | 0.019 | 0.424* | −0.159* |
NRA | 0.243* | 0.335* | 0.148* | 0.010 |
APA | −0.240* | 0.153* | 0.089 | 0.163* |
Total internal C | −0.010 | 0.293* | 0.027 | 0.442* |
Total internal N | −0.034 | 0.573* | 0.352* | 0.373* |
Internal C:N atomic ratio | 0.004 | −0.346* | −0.301* | −0.215* |
Internal | −0.086 | 0.399* | 0.091 | 0.296* |
Soluble carbohydrates | −0.258* | 0.234* | −0.036 | 0.514* |
Soluble proteins | −0.328* | 0.258* | −0.238* | 0.450* |
Chlorophyll a | 0.463* | 0.099 | 0.463* | −0.071 |
Total carotenoids | 0.464* | 0.112* | 0.451* | −0.082 |
Accessory pigments:Chlorophyll a | 0.316* | 0.166* | 0.302* | 0.040 |
Percentage of variance | 31.075 | 22.866 | 29.377 | 21.291 |
Cumulative percentage | 31.075 | 53.941 | 29.377 | 50.668 |
Control | Enriched | |||
Axis I | Axis II | Axis I | Axis II | |
CAext | 0.414* | 0.019 | 0.424* | −0.159* |
NRA | 0.243* | 0.335* | 0.148* | 0.010 |
APA | −0.240* | 0.153* | 0.089 | 0.163* |
Total internal C | −0.010 | 0.293* | 0.027 | 0.442* |
Total internal N | −0.034 | 0.573* | 0.352* | 0.373* |
Internal C:N atomic ratio | 0.004 | −0.346* | −0.301* | −0.215* |
Internal | −0.086 | 0.399* | 0.091 | 0.296* |
Soluble carbohydrates | −0.258* | 0.234* | −0.036 | 0.514* |
Soluble proteins | −0.328* | 0.258* | −0.238* | 0.450* |
Chlorophyll a | 0.463* | 0.099 | 0.463* | −0.071 |
Total carotenoids | 0.464* | 0.112* | 0.451* | −0.082 |
Accessory pigments:Chlorophyll a | 0.316* | 0.166* | 0.302* | 0.040 |
Percentage of variance | 31.075 | 22.866 | 29.377 | 21.291 |
Cumulative percentage | 31.075 | 53.941 | 29.377 | 50.668 |
The percentage of variance corresponding to each axis is also given. Significant loading factors are indicated by an asterisk (P <0.05).
Loading factors for each variable of principal components analyses shown in Fig. 1
Control | Enriched | |||
Axis I | Axis II | Axis I | Axis II | |
CAext | 0.414* | 0.019 | 0.424* | −0.159* |
NRA | 0.243* | 0.335* | 0.148* | 0.010 |
APA | −0.240* | 0.153* | 0.089 | 0.163* |
Total internal C | −0.010 | 0.293* | 0.027 | 0.442* |
Total internal N | −0.034 | 0.573* | 0.352* | 0.373* |
Internal C:N atomic ratio | 0.004 | −0.346* | −0.301* | −0.215* |
Internal | −0.086 | 0.399* | 0.091 | 0.296* |
Soluble carbohydrates | −0.258* | 0.234* | −0.036 | 0.514* |
Soluble proteins | −0.328* | 0.258* | −0.238* | 0.450* |
Chlorophyll a | 0.463* | 0.099 | 0.463* | −0.071 |
Total carotenoids | 0.464* | 0.112* | 0.451* | −0.082 |
Accessory pigments:Chlorophyll a | 0.316* | 0.166* | 0.302* | 0.040 |
Percentage of variance | 31.075 | 22.866 | 29.377 | 21.291 |
Cumulative percentage | 31.075 | 53.941 | 29.377 | 50.668 |
Control | Enriched | |||
Axis I | Axis II | Axis I | Axis II | |
CAext | 0.414* | 0.019 | 0.424* | −0.159* |
NRA | 0.243* | 0.335* | 0.148* | 0.010 |
APA | −0.240* | 0.153* | 0.089 | 0.163* |
Total internal C | −0.010 | 0.293* | 0.027 | 0.442* |
Total internal N | −0.034 | 0.573* | 0.352* | 0.373* |
Internal C:N atomic ratio | 0.004 | −0.346* | −0.301* | −0.215* |
Internal | −0.086 | 0.399* | 0.091 | 0.296* |
Soluble carbohydrates | −0.258* | 0.234* | −0.036 | 0.514* |
Soluble proteins | −0.328* | 0.258* | −0.238* | 0.450* |
Chlorophyll a | 0.463* | 0.099 | 0.463* | −0.071 |
Total carotenoids | 0.464* | 0.112* | 0.451* | −0.082 |
Accessory pigments:Chlorophyll a | 0.316* | 0.166* | 0.302* | 0.040 |
Percentage of variance | 31.075 | 22.866 | 29.377 | 21.291 |
Cumulative percentage | 31.075 | 53.941 | 29.377 | 50.668 |
The percentage of variance corresponding to each axis is also given. Significant loading factors are indicated by an asterisk (P <0.05).
In nutrient-enriched thalli, red and green algae occupied a region of the axis I–axis II space similar to the control situation; however, brown algae no longer occupied the middle region between red and green algae, but expanded mainly along axis I. Moreover, four out of nine brown species fell within the regions delimited by red (three species) and green (one species). The Laminariales shifted to the left hand side of the brown region (species 13, 18, and 19; Table 1). Regarding the variance along axis I, the pigment content contributed with the highest loading factor (Table 7) followed by CAext, internal N, the C:N ratio, and the ratio accessory pigments:chlorophyll a. Axis II was mainly influenced by variables directly related to C and N composition of the biomass, such as total internal carbon, carbohydrates, proteins, and total internal N. The relative position of species along axis II changed little upon nutrient enrichment.
Discussion
The presence of CAext has been hypothesized to relate to the habitat in which populations develops (Giordano and Maberly, 1989). Although no clear relationship has yet been established (Mercado et al., 1998), it is generally assumed that the presence of CAext has ecological relevance. A particular case is the high CAext value found for P. crispa. This species lives on land away from seawater and its high CAext can be ascribed to a need to convert CO2 to , providing the substrate for a presumed transporter. This mechanism was earlier suggested by Raven et al. (1982) and Badger (1987). In general, CAext seems to play a major role in the Arctic seaweeds community as evidenced from PCAs. The CAext loading factors for axis I (the most relevant) in both control and enriched situations were among the highest, together with pigment composition. Theoretically, the impact of low temperature on the photosynthesis of marine macrophytes is predicted to favour diffusive CO2 entry rather than a CO2-concentrating mechanism (Raven et al., 2002); however, the average value of CAext was much higher for Arctic species (19 REA g−1 FW ) than those reported for macroalgae from more temperate waters, such as those from Australia (6.4 REA g−1 FW; Graham and Smillie, 1976), Scotland (5.5 REA g−1 FW; Giordano and Maberly, 1989), and Southern Spain (3.6 REA g−1 FW; Mercado et al., 1998). We propose to call this ‘the Arctic paradox’ of the Ci uptake system. In principle, Arctic seawater has a higher concentration of dissolved CO2 than temperate waters, because of lower water temperature and lower salinity. Even though it is a well-known phenomenon that a higher concentration of CO2 represses CA activity, CAext is difficult to relate to the ability to use (Giordano and Maberly, 1989; Mercado et al., 1998), so that the high CAext values found in the present work may not be related to versus CO2-only users. The explanation for this paradox could be that high levels of CAext are present in Arctic species as part of a general strategy to cope with low temperatures. This strategy consists of a general increase in the cellular level of enzymes involved in photosynthesis, according to Davison (1987), and is invoked to operate in Arctic macroalgae (Korb and Gerard, 2000b). Furthermore, increased CAext as an acclimation to low temperature has recently been observed in macroalgae from a temperate environment (FJL Gordillo, unpublished results).
The general decrease in CAext activity following nutrient addition (Table 6) contradicts previous findings that N-supply enhances CAext activity (Jiménez del Río et al., 1995). These authors ascribe the increase to N-limitation prior to N addition. This may not be the case for Arctic macroalgae as discussed below. On the other hand, they added N in the form of instead of . The latter requires more energy in order to be incorporated into new biomass (eight extra electrons for each atom of N). CAext is involved in energy-costly CCMs, and in a nitrate-enriched situation, energetic demand may be enhanced by competitive mechanisms such as nitrate assimilation, and presumably, macromolecule biosynthesis may take place, decreasing the energetic investment into C assimilation (Gordillo et al., 2001c). This is consistent with the general increase in NRA observed (Tables 2, 6). The evidence that CAext plays a major (yet unknown) role in Arctic communities needs further and more detailed investigations, that fall beyond the scope of the present study.
Nitrate reductase acitvity (NRA) seems to be directly enhanced by nitrate addition, with relatively small feedback from the N status of the cell. Species showing the greatest increase in NRA (above 2-fold the values under control conditions) already had full internal inorganic N pools; the latter showed no response or even a decrease to N enrichment. Therefore, NRA can be considered to respond to external levels of nitrate regardless of stored N. The green algae M. arcticum and Acrosiphonia sp. (to a lesser extent) were the exception, but did show a marked nitrophilic response pattern. Increased NRA, protein synthesis and stored N, leading to lower C:N points towards optimum utilization of readily available external N. This behaviour is characteristic of nitrophilic metabolism in other green macroalgae such as Ulva (Gordillo et al., 2001c).
Efficient use of low ambient irradiance for C-fixation requires a high concentration of pigments (Falkowski and Raven, 1997), and the maintenance of metabolic rates at near-freezing temperatures requires a high concentration of enzymatic proteins (Machalek et al., 1996), all of which contain nitrogen. Survival under these conditions, even with slow growth, must require relatively high levels of nitrogenous nutrients, even in those species without a nitrophilic metabolism. Despite the widespread enzymatic response (lower CAext and higher NRA) to nutrient enrichment, biomass composition was not proportionally affected. According to these results, a community facing a summer input of nutrients would have, on average, 20% less CAext activity, and could assimilate 82% more N through NRA. However, biomass C and N composition did not vary beyond 8% and 4% on average, respectively, when all 21 species are considered together. The overall C:N ratio was only affected by 7%. The maintenance of a reasonably constant C:N ratio is a quite common priority for algae when acclimating to external nutritional disturbance. Different regulatory mechanisms have been proposed; for instance, organic carbon release has been shown to maintain the metabolic integrity of the cell (Fogg, 1983; Ormerod, 1983). According to Wood and Van Valen (1990), organic carbon release would protect the photosynthetic apparatus from an overload of products that cannot be used for growth or stored. In the green seaweed U. rigida, this mechanism seems to be repressed in response to high environmental CO2 levels; thus maintaining the internal C:N balance (Gordillo et al., 2001c). It is unknown whether Arctic seaweeds are able to regulate the release of organic carbon, but they seem to maintain their metabolic integrity through a stable C:N ratio rather than the accumulation of N-rich compounds.
It is well documented that kelps from seasonally low N environments accumulate internal N-reserves that support growth when external supply is low. Arctic kelp populations seem to survive, at least in part, due to their ability to store photosynthates during the N-depleted summer, using them for growth during the following winter when nutrients become available. Traditionally, it was thought that this seasonal growth pattern was a consequence of N availability (Chapman and Lindley, 1980). However, at least in some species, this pattern is known to be under the control of an endogenous free-running circannual rhythm entrained by a critical minimum daylength in the autumn (Lüning, 1991; Schaffelke and Lüning, 1994; Henley and Dunton, 1997). Henley and Dunton (1997) reported changes in the biochemical composition of L. solidungula (an Arctic endemic species) as an effect of light availability and the interaction light×N rather than the addition of N alone. These authors suggested that the addition of N would only have a marginal effect on growth and biochemical composition, presumably due to the prevailing internal clock. Kelps studied here showed no accumulation of N after nitrate addition. Neither total internal N nor stored inorganic N increased, although some degree of acclimation was observed. The increase in the light-harvesting antennae size (as derived from pigment ratio) and soluble protein suggest an enhanced photosynthetic ability. This is further supported by significantly higher C:N values under N-enriched conditions. Lack of N accumulation and enhanced photosynthetic ability would be in agreement with the internal clock prevailing over external nutrient conditions, which in summer would promote active photosynthesis over nutrient use, as mentioned above. This acclimation strategy could also be observed by principal component analysis. Under control conditions all brown species occupied a narrow region in axis I between the red and the green group (Fig. 1), mainly due to their pigment content and CAext activity (Table 7). After nutrient addition, the brown group spreads over axis I, overlapping with the red and green region. The three Laminariales are now placed in the most negative side of axis I for the brown region (together with F. distichus and S. lomentaria) and well away from S. plumosa and C. flagelliformis and the green nitrophilic species. The variables with the most significant loading factors along axis I are similar to control conditions, except that internal N and C:N become highly relevant variables after N enrichment.
The significant increase in pigment content observed in the red algal group (Table 7) may be responsible for its displacement towards the positive side of axis I in the PCA analysis for enriched thalli with respect to control conditions (Fig. 1). This displacement would also implicate pigments, and hence photosynthesis, in their acclimation to nutrient enrichment, as supported by the overlap observed for this group with the laminarian side of the brown region. The pigment content of Arctic seaweeds is known to be higher in winter than in summer (Aguilera et al., 2002). This pattern may be speculated to be a consequence of extreme low light and/or nutrient sufficiency in winter, the opposite occurring in summer. If low pigmentation in summer is a consequence of N limitation, it is expected that nutrient enrichment would promote pigment synthesis. This seems to be the case for most red species tested here, but is not a general trend of the whole community (Table 6). The discrete effect of N enrichment on pigment synthesis agrees with previous findings according to which, pigments in Arctic seaweeds are inversely related to light availability, increasing even in summer periods when water becomes turbid by ice melting (Aguilera et al., 2002). However, it was found that the increase in the ratio of accessory pigments:chlorophyll a is a general response to nutrient enrichment, indicating that external nutrient level influences light harvesting strategies. The increase in the ratio is generally related to bigger antenna size. The result of this acclimatory response is a decrease in photosynthesis per chlorophyll a (Gordillo et al., 2001b). This makes sense when taking into account that Arctic seaweeds need a strong co-ordination between nutrient availability (normally occurring in winter) and light availability during the summer.
PCA analysis confirmed that the effects of nutrient enrichment on biomass composition play a marginal role. Loading factors for total C, total N, internal inorganic N pool, soluble carbohydrates, and soluble proteins, are generally significant in axis II (which explains less variance), rather than axis I in both analyses (control and enriched conditions; Table 7). Furthermore, changes in the relative position of species along axis II following nutrient enrichment were only discrete.
From the evidence shown here, it is inferred that Arctic macroalgae in Kongsfjord are not N-limited, at least during the first half of the summer. This is further supported by the accumulation factor for inorganic N (the difference between internal and external concentration) averaging ×1200, which is in agreement with previously reported values for N-replete brown algae from more temperate regions (Gordillo et al., 2001a).
In conclusion, the minimal effect of nutrient enrichment previously reported on Laminariales has now been confirmed at the whole community level. This may well indicate that other groups present in the Arctic could have adapted by developing similar endogenous strategies. Acclimation to summer nutrient input seems to involve mechanisms that allow for the maintenance of a stable biomass composition, rather than a rapid assimilation of the newly available resource (except in the nitrophilic species). This indicates a high degree of resilience of the algal community to a disruption in the natural nutrient availability pattern. As far as is known, there is still a lack of evidence of progressive eutrophication in the West coast of Svalbard; however, the fact that the Spitsbergen current brings rich Atlantic waters from the south makes eutrophication a likely phenomenon. The ecological balance in Kongsfjord is influenced by oceanographic conditions (Atlantic versus Arctic) (Svendsen et al., 2002; Hop et al., 2002). Biodiversity could be compromised since fast-growing nitrophilic species are likely to show enhanced growth and outcompete resilient slow-growing species.
This work was financed by a European LSF grant (AWI-69/2001) and was performed at the Ny Ålesund International Research and Monitoring Facility on Spitsbergen (Svalbard, Norway). The authors are indebted to the Norwegian Polar Institute, the Alfred Wegener Institute for Polar and Marine Research (Germany), and to Kingsbay AS. Francisco JL Gordillo and José Aguilera were financed by the ‘Ramón y Cajal’ programme of the Spanish Ministry of Education and Science.
Comments