-
PDF
- Split View
-
Views
-
Cite
Cite
Gerhard Kerstiens, Lukas Schreiber, Klaus J. Lendzian, Quantification of cuticular permeability in genetically modified plants, Journal of Experimental Botany, Volume 57, Issue 11, August 2006, Pages 2547–2552, https://doi.org/10.1093/jxb/erl015
- Share Icon Share
Abstract
More and more studies on genetically modified plants are identifying parts of the genetic code with putative involvement in creating the cuticular barrier. Unfortunately, many of these studies suffer from the inadequacy of the chosen methods to quantify, in a reasonably unambiguous way, if and how the efficacy of the cuticular barrier is affected by the genetic change. A short overview of relevant findings is given and a more stringent experimental approach to quantifying effects on cuticular permeability in genetically modified plants proposed.
Introduction
The advent of molecular biology has opened up new avenues for studying the role of individual cuticular elements or structures in building the barrier between primary green plant tissue and the environment. The cuticle acts as a barrier with regard to, for instance, water loss, uptake of nutrients or xenobiotics by leaves via their surface, invasion of leaves by bacterial and fungal micro-organisms, and penetration of UV and visible light (Kerstiens, 1996a). Only recently has it been realized that the deposition of an intact cuticle also forms a prerequisite for proper leaf development, as mutants defective in the normal development of a cuticle are characterized by post-genital fusion of leaf organs (Yephremov et al., 1999; Pruitt et al., 2000). While the overriding importance of cuticular waxes (a collective term for all cuticular compounds soluble in organic solvents) for the diffusion barrier is very well established in principle, it is not clear how it is affected by the chemical composition or the amount per unit surface area (coverage) of waxes or the cuticular polymer matrix they are embedded in and deposited onto.
For the functional characterization of putatively cuticle-relevant genes and their products at the level of the organ or organism it is, therefore, of paramount importance to determine the actual quality of the cuticular barrier and not rely on inferences from changes in cuticular thickness or amounts of waxes, as these are rather unlikely to translate directly into predictable changes in permeability. Quite a substantial number of recent studies have made an effort to do this, but unfortunately nearly all of them suffer from the transport experiments employed being rather ambiguous. Wherever stomata are present, diffusion across stomatal pores in parallel to diffusion across the cuticle is difficult to avoid or quantify. Only one report included an experiment that used a stomata-free system (tomato fruit). In all other studies the presence of stomata on all relevant surfaces (stem, leaf abaxial and adaxial) of the plants investigated presented a problem the authors did not seem to appreciate.
Reports of concomitant changes in cuticular make-up and permeability
Table 1 gives a short summary of relevant findings. Reports of changes in cuticular permeability in genetically modified plants that did not include studies of concomitant changes in cuticular make-up (i.e. structure or composition; Chen et al., 2004) have not been included.
Overview of reports of concomitant changes in cuticle (CM) make-up and permeability
Species Organs Reference | Genotype (genetic function affected) | Effect on cuticle make-up compared with wild type | Effect on putative cuticular function |
Arabidopsis thaliana Leaves Aharoni et al., 2004 | shn (transcription factor SHINE overexpressed) | •Total wax coverage: +500% •Alkane, secondary alcohol, ketone coverage: +800–1000% | •Rate of relative rosette fresh weight loss increased several-fold •Chlorophyll extracted more rapidly |
Arabidopsis thaliana Stems and leaves Chen et al., 2003 | wax2 mutant (WAX2 coding for a predicted 72.3 kD membrane protein) | •Stem CM thickness: +36% •Stem CM coverage: −20% and structurally disorganized •Stem wax coverage: −80% Leaf wax coverage: −80% •Clear changes in stem and leaf wax composition patterns | •Whole-plant transpiration in darkness (per g DW) approximately doubled •Faster desiccation of detached leaves •Chlorophyll extracted more rapidly •Higher susceptibility to foliarly applied herbicides •Post-genital fusion of aerial organs |
Arabidopsis thaliana Leaves and inflorescences Lolle et al., 1997, Tanaka et al., 2004 | fdh-1 mutant (FIDDLEHEAD coding for putative long-chain fatty acid elongase) | •Cuticle discontinuous (plant takes up water-soluble dye via shoot surface) | •Chlorophyll extracted more rapidly •Post-genital fusion of aerial organs |
Arabidopsis thaliana Leaves and inflorescences Sieber et al., 2000 | Col-0/gl1 (plants expressing fungal cutinase) | •Stem CM of uneven thickness •Loose leaf CM structure •Wax deposition unimpaired | •Chlorophyll extracted more rapidly •Cutinase activity leached more rapidly in aqueous solution •Higher susceptibility to foliarly applied herbicides •Post-genital fusion of aerial organs |
Arabidopsis thaliana Plants Xiao et al., 2004 | att1 mutant (ATT1 coding for a putative fatty acid ω-hydroxylase, CYP86A2) | •Cutin coverage (inflorescence stem): −70% •Dominant monomer: 49→34% (hexadecane-1,16-dioic acid) •Cutin/cutan ratio: little change •Loose leaf CM structure | •Whole-plant transpiration in darkness (per g DW) approximately doubled •Greater susceptibility to virulent Pseudomonas syringiae pv. tomato |
Lycopersicon esculentum Leaves and fruits Vogg et al., 2004 | lecer6 (homozygous) [deficiency in CER6 (CUT1)-like very-long-chain fatty acid β-ketoacyl-CoA synthase] | •C ≥31-n-alkanes drastically reduced (in leaves and fruits) •Cyclic triterpenoids increased (only in fruits) •Strippable fruit waxes: −50% | •Proportion of fruits wrinkled: 4%→49% •Cuticular permeability (in intact fruits): +300% |
Medicago sativa Leaves Zhang et al., 2005 | Transgenic alfalfa (putative transcription factor WXP1 overexpressed) | •Wax coverage: +30% •Clear changes in wax development and composition patterns | •Slower desiccation of detached leaves •Chlorophyll extracted more slowly •Improved recovery from drought |
Sorghum bicolor Leaf blades Jenks et al., 1994 | bm-22 mutant (single-locus mutation affecting CM and waxes) | •CM thickness: −60% •CM coverage: −80% •CM ultra-structure altered •Wax coverage: −70% | •Whole-plant conductance in darkness: +150% •Necrosis through blight: +260% |
Zea mays Juvenile leaves Sturaro et al., 2005 | gl1 mutant (GLOSSY1 coding for a predicted membrane protein) | •CM thickness: −50% •CM ultra-structure altered •Wax coverage: −70% | •Chlorophyll extracted more rapidly |
Species Organs Reference | Genotype (genetic function affected) | Effect on cuticle make-up compared with wild type | Effect on putative cuticular function |
Arabidopsis thaliana Leaves Aharoni et al., 2004 | shn (transcription factor SHINE overexpressed) | •Total wax coverage: +500% •Alkane, secondary alcohol, ketone coverage: +800–1000% | •Rate of relative rosette fresh weight loss increased several-fold •Chlorophyll extracted more rapidly |
Arabidopsis thaliana Stems and leaves Chen et al., 2003 | wax2 mutant (WAX2 coding for a predicted 72.3 kD membrane protein) | •Stem CM thickness: +36% •Stem CM coverage: −20% and structurally disorganized •Stem wax coverage: −80% Leaf wax coverage: −80% •Clear changes in stem and leaf wax composition patterns | •Whole-plant transpiration in darkness (per g DW) approximately doubled •Faster desiccation of detached leaves •Chlorophyll extracted more rapidly •Higher susceptibility to foliarly applied herbicides •Post-genital fusion of aerial organs |
Arabidopsis thaliana Leaves and inflorescences Lolle et al., 1997, Tanaka et al., 2004 | fdh-1 mutant (FIDDLEHEAD coding for putative long-chain fatty acid elongase) | •Cuticle discontinuous (plant takes up water-soluble dye via shoot surface) | •Chlorophyll extracted more rapidly •Post-genital fusion of aerial organs |
Arabidopsis thaliana Leaves and inflorescences Sieber et al., 2000 | Col-0/gl1 (plants expressing fungal cutinase) | •Stem CM of uneven thickness •Loose leaf CM structure •Wax deposition unimpaired | •Chlorophyll extracted more rapidly •Cutinase activity leached more rapidly in aqueous solution •Higher susceptibility to foliarly applied herbicides •Post-genital fusion of aerial organs |
Arabidopsis thaliana Plants Xiao et al., 2004 | att1 mutant (ATT1 coding for a putative fatty acid ω-hydroxylase, CYP86A2) | •Cutin coverage (inflorescence stem): −70% •Dominant monomer: 49→34% (hexadecane-1,16-dioic acid) •Cutin/cutan ratio: little change •Loose leaf CM structure | •Whole-plant transpiration in darkness (per g DW) approximately doubled •Greater susceptibility to virulent Pseudomonas syringiae pv. tomato |
Lycopersicon esculentum Leaves and fruits Vogg et al., 2004 | lecer6 (homozygous) [deficiency in CER6 (CUT1)-like very-long-chain fatty acid β-ketoacyl-CoA synthase] | •C ≥31-n-alkanes drastically reduced (in leaves and fruits) •Cyclic triterpenoids increased (only in fruits) •Strippable fruit waxes: −50% | •Proportion of fruits wrinkled: 4%→49% •Cuticular permeability (in intact fruits): +300% |
Medicago sativa Leaves Zhang et al., 2005 | Transgenic alfalfa (putative transcription factor WXP1 overexpressed) | •Wax coverage: +30% •Clear changes in wax development and composition patterns | •Slower desiccation of detached leaves •Chlorophyll extracted more slowly •Improved recovery from drought |
Sorghum bicolor Leaf blades Jenks et al., 1994 | bm-22 mutant (single-locus mutation affecting CM and waxes) | •CM thickness: −60% •CM coverage: −80% •CM ultra-structure altered •Wax coverage: −70% | •Whole-plant conductance in darkness: +150% •Necrosis through blight: +260% |
Zea mays Juvenile leaves Sturaro et al., 2005 | gl1 mutant (GLOSSY1 coding for a predicted membrane protein) | •CM thickness: −50% •CM ultra-structure altered •Wax coverage: −70% | •Chlorophyll extracted more rapidly |
Effect sizes given are mostly as rounded and in some cases summary figures.
Overview of reports of concomitant changes in cuticle (CM) make-up and permeability
Species Organs Reference | Genotype (genetic function affected) | Effect on cuticle make-up compared with wild type | Effect on putative cuticular function |
Arabidopsis thaliana Leaves Aharoni et al., 2004 | shn (transcription factor SHINE overexpressed) | •Total wax coverage: +500% •Alkane, secondary alcohol, ketone coverage: +800–1000% | •Rate of relative rosette fresh weight loss increased several-fold •Chlorophyll extracted more rapidly |
Arabidopsis thaliana Stems and leaves Chen et al., 2003 | wax2 mutant (WAX2 coding for a predicted 72.3 kD membrane protein) | •Stem CM thickness: +36% •Stem CM coverage: −20% and structurally disorganized •Stem wax coverage: −80% Leaf wax coverage: −80% •Clear changes in stem and leaf wax composition patterns | •Whole-plant transpiration in darkness (per g DW) approximately doubled •Faster desiccation of detached leaves •Chlorophyll extracted more rapidly •Higher susceptibility to foliarly applied herbicides •Post-genital fusion of aerial organs |
Arabidopsis thaliana Leaves and inflorescences Lolle et al., 1997, Tanaka et al., 2004 | fdh-1 mutant (FIDDLEHEAD coding for putative long-chain fatty acid elongase) | •Cuticle discontinuous (plant takes up water-soluble dye via shoot surface) | •Chlorophyll extracted more rapidly •Post-genital fusion of aerial organs |
Arabidopsis thaliana Leaves and inflorescences Sieber et al., 2000 | Col-0/gl1 (plants expressing fungal cutinase) | •Stem CM of uneven thickness •Loose leaf CM structure •Wax deposition unimpaired | •Chlorophyll extracted more rapidly •Cutinase activity leached more rapidly in aqueous solution •Higher susceptibility to foliarly applied herbicides •Post-genital fusion of aerial organs |
Arabidopsis thaliana Plants Xiao et al., 2004 | att1 mutant (ATT1 coding for a putative fatty acid ω-hydroxylase, CYP86A2) | •Cutin coverage (inflorescence stem): −70% •Dominant monomer: 49→34% (hexadecane-1,16-dioic acid) •Cutin/cutan ratio: little change •Loose leaf CM structure | •Whole-plant transpiration in darkness (per g DW) approximately doubled •Greater susceptibility to virulent Pseudomonas syringiae pv. tomato |
Lycopersicon esculentum Leaves and fruits Vogg et al., 2004 | lecer6 (homozygous) [deficiency in CER6 (CUT1)-like very-long-chain fatty acid β-ketoacyl-CoA synthase] | •C ≥31-n-alkanes drastically reduced (in leaves and fruits) •Cyclic triterpenoids increased (only in fruits) •Strippable fruit waxes: −50% | •Proportion of fruits wrinkled: 4%→49% •Cuticular permeability (in intact fruits): +300% |
Medicago sativa Leaves Zhang et al., 2005 | Transgenic alfalfa (putative transcription factor WXP1 overexpressed) | •Wax coverage: +30% •Clear changes in wax development and composition patterns | •Slower desiccation of detached leaves •Chlorophyll extracted more slowly •Improved recovery from drought |
Sorghum bicolor Leaf blades Jenks et al., 1994 | bm-22 mutant (single-locus mutation affecting CM and waxes) | •CM thickness: −60% •CM coverage: −80% •CM ultra-structure altered •Wax coverage: −70% | •Whole-plant conductance in darkness: +150% •Necrosis through blight: +260% |
Zea mays Juvenile leaves Sturaro et al., 2005 | gl1 mutant (GLOSSY1 coding for a predicted membrane protein) | •CM thickness: −50% •CM ultra-structure altered •Wax coverage: −70% | •Chlorophyll extracted more rapidly |
Species Organs Reference | Genotype (genetic function affected) | Effect on cuticle make-up compared with wild type | Effect on putative cuticular function |
Arabidopsis thaliana Leaves Aharoni et al., 2004 | shn (transcription factor SHINE overexpressed) | •Total wax coverage: +500% •Alkane, secondary alcohol, ketone coverage: +800–1000% | •Rate of relative rosette fresh weight loss increased several-fold •Chlorophyll extracted more rapidly |
Arabidopsis thaliana Stems and leaves Chen et al., 2003 | wax2 mutant (WAX2 coding for a predicted 72.3 kD membrane protein) | •Stem CM thickness: +36% •Stem CM coverage: −20% and structurally disorganized •Stem wax coverage: −80% Leaf wax coverage: −80% •Clear changes in stem and leaf wax composition patterns | •Whole-plant transpiration in darkness (per g DW) approximately doubled •Faster desiccation of detached leaves •Chlorophyll extracted more rapidly •Higher susceptibility to foliarly applied herbicides •Post-genital fusion of aerial organs |
Arabidopsis thaliana Leaves and inflorescences Lolle et al., 1997, Tanaka et al., 2004 | fdh-1 mutant (FIDDLEHEAD coding for putative long-chain fatty acid elongase) | •Cuticle discontinuous (plant takes up water-soluble dye via shoot surface) | •Chlorophyll extracted more rapidly •Post-genital fusion of aerial organs |
Arabidopsis thaliana Leaves and inflorescences Sieber et al., 2000 | Col-0/gl1 (plants expressing fungal cutinase) | •Stem CM of uneven thickness •Loose leaf CM structure •Wax deposition unimpaired | •Chlorophyll extracted more rapidly •Cutinase activity leached more rapidly in aqueous solution •Higher susceptibility to foliarly applied herbicides •Post-genital fusion of aerial organs |
Arabidopsis thaliana Plants Xiao et al., 2004 | att1 mutant (ATT1 coding for a putative fatty acid ω-hydroxylase, CYP86A2) | •Cutin coverage (inflorescence stem): −70% •Dominant monomer: 49→34% (hexadecane-1,16-dioic acid) •Cutin/cutan ratio: little change •Loose leaf CM structure | •Whole-plant transpiration in darkness (per g DW) approximately doubled •Greater susceptibility to virulent Pseudomonas syringiae pv. tomato |
Lycopersicon esculentum Leaves and fruits Vogg et al., 2004 | lecer6 (homozygous) [deficiency in CER6 (CUT1)-like very-long-chain fatty acid β-ketoacyl-CoA synthase] | •C ≥31-n-alkanes drastically reduced (in leaves and fruits) •Cyclic triterpenoids increased (only in fruits) •Strippable fruit waxes: −50% | •Proportion of fruits wrinkled: 4%→49% •Cuticular permeability (in intact fruits): +300% |
Medicago sativa Leaves Zhang et al., 2005 | Transgenic alfalfa (putative transcription factor WXP1 overexpressed) | •Wax coverage: +30% •Clear changes in wax development and composition patterns | •Slower desiccation of detached leaves •Chlorophyll extracted more slowly •Improved recovery from drought |
Sorghum bicolor Leaf blades Jenks et al., 1994 | bm-22 mutant (single-locus mutation affecting CM and waxes) | •CM thickness: −60% •CM coverage: −80% •CM ultra-structure altered •Wax coverage: −70% | •Whole-plant conductance in darkness: +150% •Necrosis through blight: +260% |
Zea mays Juvenile leaves Sturaro et al., 2005 | gl1 mutant (GLOSSY1 coding for a predicted membrane protein) | •CM thickness: −50% •CM ultra-structure altered •Wax coverage: −70% | •Chlorophyll extracted more rapidly |
Effect sizes given are mostly as rounded and in some cases summary figures.
The picture arising from these studies is that an increase in cuticular permeability may be associated with a decrease (Jenks et al., 1994; Sturaro et al., 2005) or an increase (Chen et al., 2003) in cuticular thickness or coverage, as well as a decrease (Jenks et al., 1994; Chen et al., 2003; Vogg et al., 2004; Sturaro et al., 2005) or increase (Aharoni et al., 2004) in wax coverage, or change in cuticle polymer (Xiao et al., 2004) or wax (Aharoni et al., 2004; Vogg et al., 2004) composition. Only one study reported a decrease in permeability (Zhang et al., 2005). This could mean that the metabolism responsible for the formation of the cuticular barrier is very finely tuned and has very little scope to compensate for nearly any disturbance caused by genetic modification, or it could mean that not all these findings are reliable (or both).
The main problem for the interpretation of such results lies with the various ways in which cuticular permeability has been assessed. One method is the characterization of water permeability through measurement of mass loss of intact plants in darkness, when stomata were assumed to be completely closed. This assumption carries a high degree of uncertainty (Kerstiens, 1996b), and diffusion through even very narrow stomatal apertures (in the order of 0.1 μm) can contribute similar amounts to overall water loss as cuticular transpiration itself (Kerstiens, 1996c). Genetic changes that affect the cuticle may also affect stomata (Holroyd et al., 2002; Chen et al., 2003), and even very slight differences between genotypes in stomatal numbers and/or behaviour may mimic or mask effects on cuticular permeability.
A further cause of ambiguity is the unfortunate practice of referencing mass loss by transpiration to dry or fresh weight rather than surface area. This is a crucial omission as rates of water loss across the leaf surface will then seem to be different in leaves of different thickness even when they are actually the same per unit surface area. Therefore, in order to be able to compare surface permeability (or minimum conductance) of the wild type with that of the mutant, rates of water loss should be referenced to the surface area across which water transport has been measured. In not a single study listed in Table 1 is it stated whether the specific leaf area (i.e. the ratio of leaf area to leaf dry weight) and/or relative water content at the start of the measurement were the same in wild-type and genetically modified plants.
Extraction rates of chlorophyll from intact plant tissue by organic solvents (usually 80% ethanol) have also been used as supposed proxies for cuticular permeability. However, there is always a danger that organic solvents, even polar ones like ethanol or acetone, will dissolve some of the waxes, which before the measurement constituted the main diffusion barrier in cuticles, and/or affect the cuticular matrix by swelling. Moreover, the permeability of biomembranes within the tissues is clearly important for chlorophyll leaching, and it is evident that the main area of exchange between tissue and external solution will be the mesophyll surface rather than the external plant surface, as organic solvents such as ethanol or acetone spontaneously infiltrate leaves through open stomata.
Perhaps the ‘safest’ simple method is the analysis of transpiration decline curves of detached leaves or shoots, where the linear slope normally attained after about 30 min under well-ventilated conditions is proportional to the transpiration rate at maximum stomatal closure. However, just as with transpiration measurements on living plants in darkness, very substantial interference from very slight differences between genotypes in the stomata's ability to close can never be ruled out.
A more reliable method for assessing cuticular permeability
When reasonably large and flat pieces of cuticle can be isolated enzymatically from astomatous leaf or fruit surfaces, an array of methods is available for quantifying cuticular permeability to a wide range of substances. However, for most species used in research with genetically modified plants, such isolated cuticles are not available, and the relevant plant surfaces all carry stomata and often trichomes. Thus, there is a need for a meaningful and reliable method to be established and standardized that can be used to quantify cuticular permeability in such plants.
The proposed method consists of immersing the entire relevant part of the shoot in an aqueous solution buffered at pH 3.0 and containing a radiolabelled weak organic acid such as benzoic acid—with relatively high solubility in water and low volatility—and determining at regular intervals the amount of radioactivity that has entered the plant through a known surface area (which can be determined after the uptake experiment). Owing to the high surface tension of the solution, it will not enter through stomatal pores, and because of the low volatility of the test compound, very little of it will leave the solution at the interface with any potentially open stomata and enter the leaf in the gas phase. Because the solution in which the leaf or shoot is immersed contains no surfactants, with the result that air bubbles may adhere to the plant surface, the solution may not always be in complete contact with the leaf or stem. Therefore, it is possible that the actual permeability is somewhat underestimated. To minimize this effect, the solution should be gently stirred or shaken during the experiment. The driving force for penetration into and across the cuticle is the concentration of the undissociated acid.
Some uptake will occur across the cut surface if it is unavoidable to immerse it in the solution, but by discarding the 10–15 mm (depending on duration of the uptake experiment) of tissue closest to the wound the problem can be avoided (Schreiber and Schönherr, 1992). When plants grow under non-sterile conditions, epiphyllic microflora may absorb some of the test compound and mimic cuticular uptake (Schreiber and Schönherr, 1993). Bathing the stem in an aqueous solution of 0.01 M NaN3 for several hours prior to the experiment will sterilize the surface and remove the extraneous uptake compartment (Schreiber and Schönherr, 1993).
The plant is not damaged by immersion in the solution. A proportion of the immersed organs can still be used for further microscopic or analytical studies, except for an amount of plant material sufficiently large to detect the radioactivity taken up. The amount necessary can be adjusted by the choice of specific activity of the test compound and time allowed for scintillation counting. If the plant has broad leaves, an alternative to immersion is to supply the solution in glass cylinders sealed to the leaf surface. This experimental design allows distinguishing between uptake across the ad- and abaxial leaf surfaces (Kirsch et al., 1997).
The application of this technique to astomatous leaf surfaces (Schreiber, 1990; Kirsch et al., 1997) and isolated cuticles (Niederl et al., 1998; Schreiber, 2002) is well documented. It has also successfully been applied to stomatous surfaces of relatively broad leaves (Schreiber, 1990) and narrow grass blades (Schreiber and Schönherr, 1992). With leaves of Alnus glutinosa, Quercus robur, and Tilia cordata it was found (using 2,4-dichlorophenoxyacetic acid) that the permeability of the stomatous abaxial side was 1.5–4 times higher than that of the astomatous adaxial side, with no differences between experiments carried out in light or darkness (Schreiber, 1990).
A recently developed (and much more involved) technique for measuring water permeability of stomatous and astomatous isolated cuticles (Santrucek et al., 2004) now provides the opportunity to validate results from this method with independent findings. Santrucek et al. (2004) determined the true cuticular water vapour permeance (permeance being used as the variable name for the trait permeability) of ad- and abaxial isolated ivy (Hedera helix L.) leaf cuticles as 3.1×10−6 and 3.6×10−5 m s−1, respectively. That is, after deduction of the conductance of stomatal pores in the cuticle from the abaxial leaf surface its permeability was higher by a factor of 11–12 than that of the adaxial cuticle. With the approach proposed above, the permeance of the abaxial ivy leaf surface for benzoic acid was found to be 5.3 times that of the adaxial surface (Fig. 1). As this ratio is smaller than that found by Santrucek et al. (2004), there are no indications that any significant amount of benzoic acid may have entered the leaf through stomatal openings. The fact that the trend line of benzoic acid uptake into leaves went through the origin also indicates the absence of uptake through stomatal pores (Fig. 1).
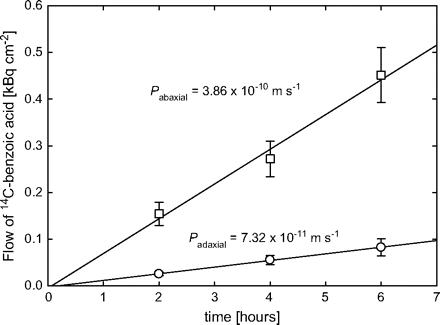
Uptake of radiolabelled benzoic acid into intact ivy (Hedera helix) leaves. The donor solution was applied to either the adaxial (circles) or abaxial leaf surface (squares). The permeances to benzoic acid (Pi with i=adaxial or abaxial) were calculated from the slopes (Bq m−2 s−1) divided by the driving force across the cuticle, i.e. the concentration C (Bq m−3) of undissociated acid in the donor solution, which was calculated as C=Ctotal/(1+104.2–pH) with Ctotal being the overall concentration of dissociated and undissociated benzoic acid at pH=3.0. Permeance to water vapour (Pwv in m s−1) at 25 °C and standard pressure, referenced to the gas phase as in much of the relevant literature, can be estimated from these data by the following equation: Pwv=43384×10(0.86×logPi–1.32), where the term in the exponent represents the empirical relationship (Niederl et al., 1998) between water permeance (referenced to the liquid phase) and benzoic acid permeance Pi, and the pre-exponential factor, the ratio of water density in the liquid phase and its saturation concentration in the gas phase at 25 °C (Becker et al., 1986).
It is difficult to predict to what extent benzoic acid will diffuse in aqueous pores in cuticles, which are important pathways for salts and may play a role in the transport of water as well (Schreiber, 2005; Schlegel et al., 2005; Schönherr, 2006). However, given that their size is near to that of the estimated pore diameters, the small volume of aqueous pores is relative to that of the bulk cuticle, the fraction of dissociated molecules at the experimental pH of 3.0 is only 6%, and the concentration of undissociated molecules in the cuticle is approximately 50 times greater than in water, it seems likely that this pathway plays no substantial role in the uptake of benzoic acid, even though mobility in the pore will be greater than in the solid cuticle. In fact, in a recent study comparing the behaviour of groups of lipophilic and hydrophilic organic compounds of similar size in ivy cuticles the indication was that benzoic acid (at pH 2.0) diffused solely within the lipophilic phase and not in aqueous pores at all (Popp et al., 2005). Therefore, this assay will probably not reveal any effects the genetic modifications may have on the network of aqueous pathways.
With this caveat in mind, it is possible to estimate a water vapour permeance from the permeance to benzoic acid by using the species-independent linear relationship (Niederl et al., 1998) between the logarithmatized permeabilities for benzoic acid and liquid water in a water–cuticle–water system [see Fig. 1; note that water permeance is not necessarily constant for a given cuticle and may depend on water content of the cuticle and other factors (Kerstiens, 1996a, 2006)]. This allows recalculating water permeance (referenced to water concentration in the gas phase; see Fig. 1 and Becker et al., 1986) to achieve direct comparability with data from Santrucek et al. (2004). The resulting values for the ad- and abaxial ivy leaf surface are 4.0×10−6 and 1.7×10−5 m s−1, respectively. Given that the experiments were carried out with different populations of ivy leaves, the correspondence between the results is more than acceptable. The values are also in good agreement with the results from transpiration decline curves of detached ivy leaves whose abaxial sides were either coated with paraffin wax or left untreated (Burghardt and Riederer, 2003); the permeances for the ad- and abaxial surface were 2.8×10−6 and 1.1×10−5 m s−1, respectively. This again indicates that uptake of benzoic acid through stomatal pores does not present a problem, and confirms that the proposed method should allow reliable quantification of differences in cuticular permeability between genotypes.
There may be reasons why transport along aqueous pathways is of interest in the system under investigation, for example, because the composition of cutin and hence the fixed charges in the membrane are affected by genetic manipulation, or because there is reason to think that pores may play a significant role in, say, cuticular transpiration of that particular plant system, or because uptake of salts is of particular importance. In such cases one should consider an analogue experimental assay using radiolabelled CaCl2 instead of benzoic acid (Schönherr, 2000).
Conclusions
In order to use the full potential of genetically modified plants as research tools for understanding the role of cuticle structure and its components in assembling the cuticular barrier, it is crucial to use appropriate methods to quantify barrier quality, at least in relative terms, leaving as little room for ambiguity as possible. By immersing leaves or stems in a buffered and mixed solution of a radiolabelled weak organic acid of low volatility, such an assessment is achievable with relatively modest experimental effort.
The authors wish to thank Professor Dr Jörg Schönherr for organizing the workshop from which this paper originated, for his critical comments on the manuscript and valuable suggestions. The paper is dedicated to Professor Schönherr, on the occasion of his retirement, in appreciation of his enormous lifetime contribution to understanding transport in plant cuticles.
Comments