-
PDF
- Split View
-
Views
-
Cite
Cite
Yong-Ling Ruan, Danny J. Llewellyn, Robert T. Furbank, Prem S. Chourey, The delayed initiation and slow elongation of fuzz-like short fibre cells in relation to altered patterns of sucrose synthase expression and plasmodesmata gating in a lintless mutant of cotton, Journal of Experimental Botany, Volume 56, Issue 413, March 2005, Pages 977–984, https://doi.org/10.1093/jxb/eri091
- Share Icon Share
Abstract
Cotton (Gossypium hirsutum L.) seed develops single-celled long fibres (lint) from the seed-coat epidermis at anthesis. Previous studies have shown that the initiation and rapid elongation of these fibres requires the expression of sucrose synthase (Sus) and, potentially, a transient closure of plasmodesmata. This study extends the previous work to examine the patterns of Sus expression and plasmodesmata gating in fuzz-like short fibres of a mutant that shows delayed initiation and much slower and reduced elongation of the fibre cells. Immunolocalization studies revealed delayed expression of Sus in the mutant seed-coat epidermis that correlates temporally and spatially with the initiation of the fibre cells. Anatomically, these short fibres differed from the normal lint in that their basal ends enlarged immediately after initiation, while the majority of the normal lint on wild-type seed did not show this enlargement until the end of elongation. Suppression of Sus expression in the seed-coat epidermis of the transgenic plants reduced the length of both lint and short fuzz fibres at maturity, suggesting that the growth of short fibres also requires high levels of Sus expression. Confocal imaging of the membrane-impermeant fluorescent solute carboxyfluorescein (CF) revealed no closure of plasmodesmata during the entire elongation period of short fibres from the mutant seed. These results show (i) the delayed initiation of fuzz-like short fibres from the mutant seed correlates with delayed or insufficient expression of Sus in a subset of seed-coat epidermal cells destined to become fibres and (ii) the much shortened elongation of the fibres from the mutant may be related to their inability to close plasmodesmata.
This paper is available online free of all access charges (see http://jxb.oupjournals.org/open_access.html for further details)
The online version of this article has been published under an Open Access model. Users are entitled to use, reproduce, disseminate, or display the Open Access version of this article for non-commercial purposes provided that: the original authorship is properly and fully attributed; the Journal and the Society for Experimental Biology are attributed as the original place of publication with the correct citation details given; if an article is subsequently reproduced or disseminated not in its entirely but only in part or as a derivative work this must be clearly indicated. For commercial re-use, please contact [email protected]
Introduction
The distinctive feature of the seed of cotton (Gossypium hirsutum L. and related species) is that about 30% of its seed-coat epidermal cells develop into specialized single-celled fibres. Fibre initiation occurs in a wave from the chalazal end towards the micropylar end of the seed starting at or just before anthesis (Stewart, 1975). These first fibres, referred to as lint, elongate to about 2.5–3.0 cm by about 18 d after anthesis (DAA) and then synthesize massive amounts of secondary cell wall cellulose (Delmer and Amor, 1995; Ruan et al., 1997). This attribute of fibre production makes cotton the most important textile crop in the world (Basra and Malik, 1984). A second wave of fibre initiation takes place 5–10 DAA (Stewart, 1975; Beasley, 1977). These fibres, termed fuzz, elongate to less than 10% of the length of the lint (Fryxell, 1963) and remained attached to the seed after the lint is removed during ginning. These short fibres have little agronomic value although they can be used as an inert filler (e.g. in toothpaste) or paper manufacture.
Despite extensive research on cotton fibre biology over the last several decades, the mechanisms controlling fibre development are still poorly understood (see Ruan et al., 2003, and literature therein). In particular, there is little or no information about the basis of the delayed initiation and slowed elongation of the fuzz or fuzz-like short fibres. Elucidation of the cellular and molecular basis of the complex developmental programmes of fibres, for example, the initiation and elongation process, could identify potential targets for genetic manipulation of fibre properties such as the length, a key determinant of fibre yield and quality. This may eventually lead to the discovery of new approaches for the transformation of short fibre into the long lint, hence, the increase of fibre quality and productivity.
The developing cotton fibre is a highly active sink cell that utilizes sucrose as the initial carbon source for various metabolic and biosynthetic processes. In this cell type, sucrose synthase (Sus, EC 2.4.1.13) is the major enzyme to degrade sucrose imported into the fibres from the phloem of the seed coat (Ruan et al., 1997, 2000). It catalyses a reversible reaction, but preferentially converts sucrose into fructose and UDP-glucose in planta (Geigenberger and Stitt, 1993). The reaction products can be used in energy generation and the biosynthesis of diverse products, including starch and cellulose (Ruan and Chourey, 2005). Recent work showed that suppression in transgenic cotton of Sus gene expression, specifically in the ovule epidermis, inhibits fibre initiation and elongation (Ruan et al., 2003). These plants still appeared to produce fuzz-like fibres, but they were not examined in any detail. It remains unclear whether Sus also plays a role in the short fibre development.
Fibre length is a multi-gene controlled trait. Thus, in addition to the role of Sus in fibre elongation, other proteins or cellular processes could also play roles in the elongation of the fibres. In this regard, previous studies revealed a transient closure of plasmodesmata of the fibres at the onset of the rapid elongation phase (Ruan et al., 2001). It has been hypothesized that closure of plasmodesmata is required to maintain a high turgor in the cells for driving the rapid elongation of the lint fibres (Ruan et al., 2001). It would be of significance to test whether the fuzz-like short fibres have similar or altered patterns of plasmodesmata gating, compared with that of the normal lint fibres.
The availability of various fibre-defective mutants provides opportunities to study the molecular genetic and cellular basis of fibre development (Ruan and Chourey, 1998; Du et al., 2001; Turley and Kloth, 2002). Among them, a lintless mutant (fls or SL1-7-1) is of particular interest, as it produces fuzz-like short fibres that also have delayed initiation (Ruan and Chourey, 1998; Ruan et al., 2000). In this study, this mutant was used to investigate (i) the possible role of Sus expression in the delayed initiation of the short fibres, by using immunolocalization analysis coupled with the measurements of fuzz fibre length in the transgenic cotton with Sus suppressed, and (ii) the gating of plasmodesmata of the short fibres of the mutant by using confocal imaging of the membrane-impermeant fluorescent solute carboxyfluorescein (CF).
Materials and methods
Plant material
Two lines of cotton (Gossypium hirsutum L.) var. Coker 315 as the wild type and fls as the lintless mutant were grown in a soil mixture under controlled conditions as previously described (Ruan et al., 2001). Seeds of the mutant stock fls (SL1-7-1) were a gift from Dr Jim Heitholt (USDA-ARS Cotton Physiology and Genetic Research, Stoneville, MS). This is a naturally occurring dominant lintless mutant of cotton carrying the dominant N1 naked seed allele, the recessive n3 allele (Turley and Kloth, 2002) as well as an undetermined number of recessive alleles determining lint production. It is listed as accession number 504 in the Mississippi Obsolete Variety Collection.
Cotton fruit age was determined by tagging each pedicel on the day of anthesis. For enzyme assay, samples were frozen in liquid N2 and stored at −70 °C. Fresh samples were used for immunolocalization analysis and loading of carboxyfluorescein (CF).
The Sus-suppressed transgenic plants were generated as previously described (Ruan et al., 2003).
Immunolocalization
Immunolocalization was conducted as previously described (Ruan and Chourey, 1998) using the HISTOGOLD Kit (ZYMED HISTOGOLD™ SYSTEM for Immuno-Histological Staining, ZYMED Laboratories, Inc., San Francisco, CA). Briefly, paraffin-embedded samples were cut (10 μm), affixed to slides, deparaffined, rehydrated, and washed with PBS. Thereafter, slides were incubated with serum blocking solution (provided by the kit) for 10 min followed by incubation with 1:1500 diluted cotton Sus polyclonal antibodies (Ruan et al., 1997) for 30 min. After washing with PBS, slides were incubated for 30 min in a solution of secondary antibody (goat anti-rabbit IgG linked to colloidal gold) provided by the HISTOGOLD Kit. Slides were then washed thoroughly with PBS, incubated for 4 min with freshly prepared silver enhancement reagents, and washed with excess distilled water. Slides were dehydrated in an ethanol series and permanently mounted in Permount for microscopic examination.
Measuring fibre length and Sus activity
The measurement of fibre length over the elongation period was conducted as previously described (Ruan et al., 2003). For the mature Sus-suppressed transgenic seed, some residual lint fibres were removed manually from the seed. The length of the fuzz fibre was then determined from the chalazal end of the seed by taking images of the seeds under a microscope followed by the measurement of the fuzz length from the enlargements of the images.
Sus activities were assayed as described in Ruan et al., 2003.
Loading of carboxyfluorescein and confocal laser scanning microscopy
The loading of carboxyfluorescein (CF) and confocal imaging of the movement of CF into fibres were carried out as previously described (Ruan et al., 2001) at an interval of 2–3 d after the initiation of the normal lint or the short fibres. For each time point, the imaging analysis was performed on 3–4 seeds with total of at least 15 optical sections (each with 10 μm in thickness). The results were reproducible and representative images have been recorded.
Results
Sus expression is delayed in the lintless mutant and coincides with the onset of the initiation of fuzz-like short fibres
Previous work showed that, in contrast to the abundant expression of Sus in the initiating lint fibres of wild-type ovules, the ovule epidermis of a lintless mutant (fls) showed no Sus expression and no fibre initiation on the day of anthesis (Ruan and Chourey, 1998). This lintless mutant, however, did develop some short fibres on the mature seed (Ruan et al., 2000). Figure 1A shows that, by 25 DAA, some fuzz-like short fibres were already visible on the mutant seed, although the seeds were virtually lintless when compared with the wild type (Fig. 1C). By maturity, the mutant seeds had only produced a few short fibres and the seeds were visible from the mature cotton bolls (Fig. 1B; also see Ruan et al., 2000). In striking contrast, the wild-type seeds were well covered by a large quantity of long lint fibres (Fig. 1D). In order to determine when the short fibre initiates from the mutant seed and whether this is related to changes in Sus expression on the seed epidermis, immunolocalization studies were conducted at intermediate time points. Figure 2B shows that, compared with the preimmune control (Fig. 2A), Sus protein was undetectable and no fibre initiation was observed in the epidermis of the mutant seed at 5 DAA. By contrast, Sus protein was labelled in the elongating lint fibres of the wild-type seed at the same stage (Fig. 2E), compared with the preimmune control (Fig. 2D). Importantly, Sus protein was barely detectable in other parts of the seed coat or nucellus (Fig. 2E), indicating the specificity of Sus expression in the fibres on the epidermis of the wild-type seed at 5 DAA. The lack of Sus expression and fibre initiation in the mutant seed was evident under high magnification (Fig. 2C). This contrasts with the abundance of Sus protein in the fibres of the wild-type seed (Fig. 2F). Similar results were obtained at 7 DAA, i.e. neither Sus protein and nor fibre initiation were observed in the epidermis of the fls mutant, while abundant Sus proteins were detected in the fibres from the wild-type seed (data not shown).

Seeds and fruit of the lintless mutant (A, B) and wild-type (C, D) cotton. (A) The mutant fruit cut longitudinally at 25 DAA, showing the individual seeds packed inside the fruit. Note, the presence of fuzz-like short fibres in the mutant seeds (arrows). (B) The mature fruit of the mutant, showing a few of the short fibres (arrows) on the seeds. (C) The wild-type fruit cut longitudinally at 25 DAA. Note, seeds were embedded under the lint fibres. (D) The mature fruit of the wild-type, showing massive amounts of long lint fibres. Note, seeds were totally covered by the fibres and therefore were invisible. Bar=0.8 cm in (A). The scale in (B), (C) and (D) is the same as in (A).

Immunogold localization of Sus protein in lintless mutant (A–C) and wild-type seed (D–F) at 5 DAA. The black signal represents Sus proteins. (A) Cross-section of the mutant seed, treated with preimmune serum. (B) A consecutive section of (A) but treated with polyclonal antibody against cotton Sus. Note, no Sus protein signal was detected in the section. Arrows show the seed epidermis where short fibres may initiate. (C) Magnified view of the epidermal region of (B). It shows evenly arranged epidermal cells (arrow) and no Sus labelling and no fibre initiation. (D) A cross-section of wild-type seed treated with preimmune serum. (E) A consecutive section of (D) but treated with the Sus antibody, showing strong Sus signal in the elongating fibre cells. Arrowheads point to the innermost layer of the seed coat bordering the nucellus. (F) Magnified view of the epidermis in (E) showing abundant Sus proteins in the expanding fibres. Key: f, fibre; isc, inner seed coat; osc, outer seed coat; n, nucellus. Bars (in μm)=250 in (A) and 125 in (C). The scale in (B), (D) and (E) is the same as in (A). The scale in (F) is the same as in (C).
However, by 10 DAA, some fibres had initiated from the mutant seed coat epidermis (Fig. 3A–C). Importantly, compared with the preimmune control (Fig. 3A), Sus was expressed in those fibres as well as in the phloem and transfer cells at the innermost layer of the seed coat and in some endosperm cells (Fig. 3B). Under high magnification, Sus protein was specifically localized in the initiating fibres but not in the adjacent normal epidermal cells (Fig. 3C) in a manner similar to that for the wild-type lint at similar developmental stages (Ruan et al., 2003). Also noteworthy are the enlarged basal ends of the fibre cells embedded beneath the epidermis of the outer seed coat (Fig. 3C), a phenomenon not seen in wild-type lint fibres at the initiation and early elongation periods (Ruan and Chourey, 1998; Ruan et al., 2000). In the wild-type seed, Sus protein was readily labelled in the normal lint fibres at 10 DAA (Fig. 3E), compared with the preimmune control (Fig. 3D). Sus was also detected in the phloem and transfer cells of the seed coat and some endosperm cells (data not shown). Interestingly, only a few of the lint fibres showed signs of enlargement at their basal region (Fig. 3E), although the fibres had elongated to about 1.2 cm by this stage. The majority of the lint fibre did not show this enlargement until about 25 DAA (Fig. 3F), at the end of the elongation phase and at the peak of intensive secondary cell wall cellulose synthesis (Ruan et al., 2003). Similar to observations in the short fibres of the mutant (Fig. 3C), Sus protein was specifically expressed in the normal lint fibres but not in the flanking epidermal cells of the wild-type seed (Fig. 3F).
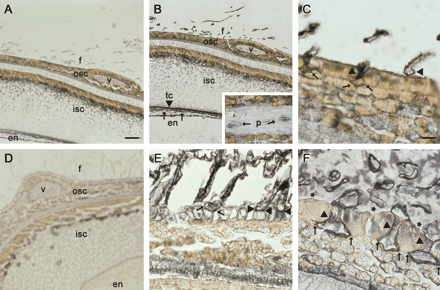
Immunogold localization of Sus protein in lintless mutant (A–C) and wild-type seed (D–F) at 10 DAA (A–E) and 25 DAA (F). The black signal represents Sus proteins. (A) Cross-section of the mutant seed, treated with preimmune serum. Note, some short fibres had initiated from the seed coat epidermis at this stage. (B) A consecutive section of (A), but treated with polyclonal antibody against cotton Sus. Sus protein signal was detected in the initiating fibres as well as in the seed coat transfer cells and endosperm. The insert shows the Sus signals in the phloem of the vascular bundle in the seed coat. (C) Magnified view of the epidermal region of (B). Note, strong Sus proteins were specifically expressed in the fibres (arrow heads), but not in the adjacent epidermal cells. Also note the enlarged basal ends of the fibres embedded underneath the epidermis (arrows). (D) A wild-type cross-section treated with preimmune serum. (E) A consecutive section of (D), but treated with the Sus antibody. It shows the region of the outer seed coat and fibres. Note the strong Sus signal in the elongating lint fibres, most of which did not show enlarged basal ends (arrow heads). One lint fibre that showed a sign of the enlargement is indicated with arrows. (F) A wild-type cross-section from 25 DAA seed, treated with the Sus antibody. Note the enlarged basal ends of the lint fibres (arrows) under the epidermal cells. Also note the specific labelling of the Sus protein in the fibres (arrow heads) but not in the neighbouring normal epidermal cells (stars). Key: en, endosperm; f, fibre; isc, inner seed coat; osc, outer seed coat; p, phloem; tc, transfer cells; v, vascular bundle. Bars (in μm)=250 in (A) and 125 in (C). The scale in (B), (D) and (E) is the same as in (A). The scale in (F) is the same as in (C).
To obtain more information on the role of Sus in short fibre development, three lines of Sus-suppressed transgenic cotton were reanalysed. These transgenic plants were characterized by repression of Sus expression specifically in the ovule epidermis at 0 DAA, leading to a lintless or lint-reduced phenotype (Ruan et al., 2003). After manually removing the residual lint fibres, the remaining short fibres (fuzz) appeared to be shorter in the transgenic seed (Fig. 4B) than that from the wild type (Fig. 4A). Further analysis of the mature seed from the selected transgenic lines shows that, in addition to the reduced lint length (Ruan et al., 2003), the reduction of Sus activity by 64–80% also reduced final fuzz fibre length by 36–60% (Table 1).

The delinted mature seed of the wild-type and a Sus-suppressed transgenic line. (A) The wild-type seed, note the remaining short fuzz fibres that covered the seed. (B) The seed from Sus-suppressed transgenic line, 147–2. Note, in comparison to the wild-type seed in (A), the fuzz fibres appeared to be shorter and thus the dark-brown seed coat became visible. The circled areas in (A) and (B) were the regions where the length of the fuzz fibres were measured.
Suppression of Sus expression in wild-type cotton seed reduced fuzz fibre length
Line . | Sus activity at 0 DAA (% of WT level) . | Fuzz length at maturity (mm) . |
---|---|---|
WT | 100.0 | 2.2±0.2a |
147-2 | 36.0 | 1.4±0.1 |
147-7 | 24.6 | 1.2±0.1 |
101-1-22 | 20.0 | 1.1±0.0 |
Line . | Sus activity at 0 DAA (% of WT level) . | Fuzz length at maturity (mm) . |
---|---|---|
WT | 100.0 | 2.2±0.2a |
147-2 | 36.0 | 1.4±0.1 |
147-7 | 24.6 | 1.2±0.1 |
101-1-22 | 20.0 | 1.1±0.0 |
Numbering of the lines is as described in Ruan et al. (2003).
Each value is the mean ±SE of six replicates.
Suppression of Sus expression in wild-type cotton seed reduced fuzz fibre length
Line . | Sus activity at 0 DAA (% of WT level) . | Fuzz length at maturity (mm) . |
---|---|---|
WT | 100.0 | 2.2±0.2a |
147-2 | 36.0 | 1.4±0.1 |
147-7 | 24.6 | 1.2±0.1 |
101-1-22 | 20.0 | 1.1±0.0 |
Line . | Sus activity at 0 DAA (% of WT level) . | Fuzz length at maturity (mm) . |
---|---|---|
WT | 100.0 | 2.2±0.2a |
147-2 | 36.0 | 1.4±0.1 |
147-7 | 24.6 | 1.2±0.1 |
101-1-22 | 20.0 | 1.1±0.0 |
Numbering of the lines is as described in Ruan et al. (2003).
Each value is the mean ±SE of six replicates.
The fuzz-like short fibres of the lintless mutant do not close their plasmodesmata over the elongation period
After initiation, the fibres of the mutant elongate much more slowly than the wild-type lint fibres (Fig. 5A). To examine whether the gating status of plasmodesmata in the fuzz-like short fibres differs from that of normal lint fibres, the phloem-mobile fluorescent probe, carboxyfluorescein (CF), was ester-loaded into shoots through their cut ends (Ruan et al., 2001). The subsequent unloading pattern of CF from the phloem of the outer seed coat to the fibre cells was monitored in situ using confocal laser scanning microscopy. Confocal analysis of the optical seed sections harvested at 2–3 d intervals revealed that the CF moved into the short fibres of the mutant seed throughout the developmental stages. In other words, the plasmodesmata of the short fibres remain open during the entire elongation period (Fig. 5A). This is in contrast to that of lint fibres in the wild-type seed where closure of plasmodesmata was observed from 10–15 DAA, coinciding with the rapid phase of elongation (Fig. 5A; Ruan et al., 2001). Figure 5B is a representative image of CF movement (green fluorescent signal) into the fuzz-like short fibres of the lintless mutant at 12 d after their initiation. Similar images were obtained at other stages examined, including 11, 13, and 15 DAI (data not shown). By contrast, in the wild-type seed at the same developmental stage, CF fluorescent signals were confined to the outer seed coat and were unable to move into the normal lint fibres (Fig. 5C). The same image was viewed at a different wavelength and superimposed on the CF fluorescent image to show the position of fibres and other parts of the seed coat due to the autofluorescence (orange colour) of phenolic compounds in those tissues (Fig. 5C).

The gating of plasmodesmata in the normal lint fibres from the wild-type and the fuzz-like short fibres of the mutant over the elongation period. (A) The time-course of fibre elongation. Black bar indicates the period of plasmodesmata closure. Note the closure of plasmodesmata occurs from 10–15 DAA in the lint fibres of the wild-type cotton, coinciding with the rapid phase of fibre elongation. Also note, no closure of plasmodesmata was observed in the short fibres of the lintless mutant and these fibres elongate much more slowly than the lint. Each value of the fibre length is the mean of six measurements with SE less than 9% of the mean. (B) A representative confocal image of the fuzz-like short fibres from the mutant at about 12 d after initiation, showing the movement of CF fluorescent signals (green) into a short fibre. (C) A representative confocal image of the normal lint fibres from the wild-type seed at 12 d after initiation, showing that the CF signals had moved throughout the outer seed coat but not into the lint fibres. The orange colour reflects the autofluorescence of the fibres. Key: f, fibre; osc, outer seed coat. Bar=0.1 and 0.2 cm in (B) and (C), respectively.
Discussion
The research on cotton fibre has been focused largely on the development of normal lint fibres (John, 1999; Ruan et al., 2003). By contrast, little work has been done in understanding the development of short fibres that exist in various mutants as well as in the wild-type seed. To date, there have been no publications about the identities of genes or cellular processes that regulate the development of fuzz or fuzz-like short fibres. Previous studies on the lint fibres of the wild-type seed have shown that the initiation and rapid elongation of these long fibres requires the expression of Sus (Ruan et al., 2003) and, potentially, a transient closure of plasmodasmata (Ruan et al., 2001). In this study, advantage was taken of a lintless mutant to explore the biochemical and cellular basis of the delayed initiation and slow elongation of fuzz-like short fibres in relation to possible altered patterns of Sus expression and plasmodesmata gating.
Here, immunolocalization analysis showed that the timing of the fuzz-like short fibre initiation on the mutant seeds coincides with that of the delayed expression of Sus in the seed coat epidermis (Figs 2, 3). Moreover, the cellular location of the initiation of the short fibres matches well with that of the Sus expression (Fig. 3). Further, in transgenic plants, suppression of Sus expression repressed the elongation not only of lint (Ruan et al., 2003) but also the short fuzz fibres (Table 1). These data suggest that fuzz-like short fibres, like lint, require Sus expression for initiation and elongation and, in this respect, are not different from lint fibre. Thus, short fibre initiation may be regulated by the timing and extent of expression of Sus and other genes essential for their development.
There are a number of reasons why the delay or reduction in Sus expression in short fibre initials or their precursors, for example, could lead to the inhibition of their initiation and elongation. Morphologically, the initiation of each fibre, regardless of type (normal lint or short fibres), is characterized by the spherical expansion and protrusion of one epidermal cell above the ovular surface (Basra and Malik, 1984). This process is achieved through the concerted action of increased cell wall extensibility and turgor pressure within the fibres (Ruan et al., 2000, 2001). Hexoses are major osmotically active solutes in the cotton fibre (Dhindsa et al., 1975; Ruan et al., 1997). Recent work showed that suppression of Sus expression significantly reduced hexose levels in the transgenic ovules, correlating with inhibited lint initiation and elongation (Ruan et al., 2003). The total absence of Sus expression seen in the mutant seed from 0 to 5 DAA (Fig. 2; Ruan and Chourey, 1998) would similarly result in reduced hexose levels in the seed coat epidermis. This would reduce the osmotic, hence turgor potential of the fibre precursors, resulting in the fibreless phenotype seen in the mutant seed at the early stages. Between 7–10 DAA, Sus is specifically expressed in some epidermal cells of the mutant seed, which may drive the initiation of the short fibres (Fig. 3). For the same reason, the partial suppression of Sus expression in the transgenic ovules may lower the turgor potential of the fuzz fibre and thus reduce their final length (Table 1).
In addition, or alternatively, absence or suppression of Sus expression may inhibit cell wall biosynthesis by reducing the supply of UDP-glucose, the substrate for the synthesis of cellulosic and many non-cellulosic cell wall compounds (Buchala, 1999) needed for cell wall synthesis during the expansion of the fibre initials. This may also contribute to the failure to initiate fibres from the seed coat epidermis of the mutant seed from 0 to 5 DAA (Fig. 2).
It is of interest to note the morphological difference between the short fibres from the mutant and the lint fibres of the wild-type seed in the timing of the enlargement of their basal part (Fig. 3). The enlarged basal region buried among the surrounding epidermal cells has been termed as the ‘foot’ by Fryxell (1963). The foot has been observed in both lint and fuzz fibres in matured seed of wild-type cotton (Fryxell, 1963). However, it has been unknown whether the foot occurs at the same developmental stage between the two types of fibres. Here, in contrast to the lint fibre that did not show the foot structure until the end of the elongation period (Fig. 3F), the foot on the short fibres of the mutant appeared immediately after initiation (Fig. 3C). This observation provides a new difference in the development of the fuzz-like short fibres versus the normal lint fibres. The significance of the development of the foot is unclear, but has been suggested (i) to anchor the fibre cells securely among the epidermal cells and (ii) to enhance nutrient uptake from the seed coat (Fryxell, 1963).
Another important observation in this study is that, in contrast to the lint fibre, the fuzz-like short fibres of the lintless mutant show no closure of plasmodesmata during the elongation period (Fig. 5). Both the lint and the short fibres interconnect the underlying seed coat epidermal cells at their basal ends where influx of nutrients, water and other molecules occur either through plasmodesmata or across plasma membranes. In the lint fibres of the wild-type seed, their plasmodesmata temporarily close at the onset of rapid phase of elongation (Fig. 5A). This closure of plasmodesmata coincides with the elevated expression of plasma membrane K+ and sucrose transporters and increases in turgor potential (Ruan et al., 2001). Interestingly, the plasmodesmata of the lint fibres are reopened at about 16 DAA, which follows the release of turgor and termination of elongation (Ruan et al., 2001). These observations lead to the suggestion that closure of the plasmodesmata is required for the fibres to maintain high turgor to drive the rapid elongation process (Ruan et al., 2001; Pfluger and Zambryski, 2001). The lack of symplastic isolation in the fuzz-like short fibres of the mutant (Fig. 5) may make it difficult or even impossible for these cells to maintain a high turgor. Consequently, these short fibres may be unable to elongate at the rate and extent of the normal lint fibres (Fig. 5).
In summary, this study provides novel insights into the biochemical and cellular basis of short fibre development in relation to Sus expression and plasmodesmata gating. The results obtained suggest (i) that the initiation of the fuzz-like short fibre may be regulated by the delayed or insufficient expression of Sus in a subset of seed coat epidermal cells destined to become fibres, and (ii) the much reduced elongation of the short fibres from the lintless mutant may be related to the absence of plasmodesmata closure during development. Given the sparse information available on short fibre development in the literature, these results represent an important advance in the understanding of the mechanisms controlling the development of fuzz-like fibres.
We thank Dr Jim Heitholt for the lintless mutant and Dr Rosemary White for assistance in confocal microscopy. The work was partly supported by the Australian Cotton Research and Development Cooperation.
References
Beasley CA.
Buchala AJ.
Du XM, Pan JJ, Wang RH, Zhang TZ, Shi YZ.
Fryxell PA.
Geigenberger P, Stitt M.
John ME.
Pfluger J, Zambryski PC.
Ruan Y-L, Chourey PS.
Ruan Y-L, Chourey PS.
Ruan Y-L, Chourey PS, Delmer PD, Perez-Grau L.
Ruan Y-L, Llewellyn DJ, Furbank RT.
Ruan Y-L, Llewellyn DJ, Furbank RT.
Ruan Y-L, Llewellyn DJ, Furbank RT.
Stewart JM.
Comments