-
PDF
- Split View
-
Views
-
Cite
Cite
J. Digby, R.D. Firn, Light modulation of the gravitropic set‐point angle (GSA), Journal of Experimental Botany, Volume 53, Issue 367, 1 February 2002, Pages 377–381, https://doi.org/10.1093/jexbot/53.367.377
- Share Icon Share
Abstract
A study has been made of the means by which light influences the gravitropic set‐point angle (GSA) of the nodes of Tradescantia and the hypocotyls of the lazy‐2 mutant of tomato. In light‐grown Tradescantia there is a light‐regulated developmental change in the GSA with the magnitude of this change being dependent on the photon flux density of white light. The photosynthetic inhibitor DCMU abolished the effect of white light. Low fluence rates of red light had no significant effect on the GSA of Tradescantia. It was concluded that there is an interaction between photosynthesis and the GSA in Tradescantia. The light‐induced reduction of the GSA of the hypocotyl of lazy‐2 tomato has previously been assumed to be solely an action of light acting via phytochrome. However, it can be shown that the GSA of hypocotyls of lazy‐2 seedlings grown in white light is sensitive to DCMU and norflurazon treatment, hence the light effects on the GSA of an organ can be mediated via both phytochrome and photosynthesis. The implication of these findings to the study of gravitropism is discussed.
Introduction
It has previously been established that the angle in relation to the gravity vector that is adopted by a plant organ, the gravitropic set‐point angle (GSA), can be influenced by light (Myers et al., 1994; Digby and Firn, 1995). The older nodes of the hanging plants Tradescantia, Oplismenus and Zebrina grow vertically upwards (GSA=180°) in darkness or very low photon flux density, whereas at high photon flux density the equivalent nodes bend downwards (GSA=0–90°). Light is also known to influence the GSA of the hypocotyls of the lazy‐2 mutant of tomato, where young hypocotyls initially grow upwards (GSA=180°) when grown under light, but after a few days they start to grow downwards (GSA<45°), but only if maintained in light (Roberts, 1987). A number of tropical plant organs also show what might now be interpreted to be light‐induced GSA changes—they are less prostrate when grown in darkness or shade than when grown in full sun (Langham, 1941; Palmer, 1956). Older studies noted that runners, stolons or prostrate stems of many plants became more erect when shaded (De Vries, 1872; Czapek, 1895; Maige, 1900). Thus light‐induced changes in the GSA of plant organs are not uncommon but the mechanism by which light brings about these effects is unclear.
Experiments with isolated stolons of Bermudagrass (Cynodon dactylon) (Montaldi, 1969) and with tillers, rhizomes and stolons of the grass Paspalum vaginatum (Willmoes et al., 1988) have shown that horizontally growing organs in high light contain high levels of sucrose, whereas shaded or dark‐grown organs which are growing upright have much lower sucrose levels. In addition, if explants of stolons are placed horizontally in the dark, they rapidly show upward curvature, but if they are fed sucrose through the cut basal ends then the explants continue to grow horizontally. This effect seems to be restricted to sucrose; glucose and fructose do not produce the same result. It is not an osmotic effect of sucrose as mannitol and polyethylene glycol cannot substitute for sucrose. These observations that high light levels cause growth to be prostrate, whereas shade or darkness produce upright growth, could suggest that photosynthesis or photoassimilate levels (sucrose) can modify the GSA of an organ.
There is, however, evidence which can now be interpreted as showing that light can also modify the GSA of organs via phytochrome A and B. A number of studies of root gravitropism have shown that exposure to red light (acting via phytochrome) can make roots grow more vertically downwards (i.e. reduces the GSA of the roots) (Mandoli et al., 1984; Feldman and Briggs, 1987). The effect of red light on the gravitropic response of the hypocotyls of the lazy‐2 mutant of tomato has also been studied extensively and it was shown that red light caused hypocotyls to adopt the lazy habit (i.e. reduced the GSA of the hypocotyls) (Roberts, 1987; Gaiser and Lomax, 1993; Behringer and Lomax, 1999). It has also been reported that the hypocotyls of Arabidopsis seedlings grown in red light are rarely vertical (Liscum and Hangarter, 1993) and it has been shown that there is an effect of red light on hypocotyl orientation that is mediated by the combined action of phytochromes A and B (Robson and Smith, 1996).
It has been suggested (Willmoes et al., 1987; Beltrano et al., 1991) that the phytochrome effects on the gravitropic behaviour of the plants they studied could be due to the modification of photoassimilate partitioning—high sucrose levels causing an organ to grow more horizontally. The present study investigates the way in which white light affects the GSA of organs and demonstrates that light acting via a photosynthetic effect has a role in the establishment of the GSA.
Materials and methods
Tradescantia experiments
Cuttings of Tradescantia flumiensis were planted vertically in Levington's compost in 5 cm square pots and maintained in a growth chamber (25 °C for a 16 h day and at 20 °C for an 8 h night, approximately 80% relative humidity and a photon flux density of 12 μmol m−2 s−1 from Osram warm white fluorescent lamps during the day) for 7 d before use in experiments. The oldest node was denoted as node 1 and sequential numbers were given to later nodes. The angle with respect to the gravity vector (vertically downwards=0°, vertically upwards=180°) of each node was measured during the course of the experiments but, except where noted, only the data for node 3 are presented. This node was chosen because the node was present at the time of establishment of the cuttings and is representative of the developmental progression that any node undergoes. All experiments were repeated at least twice, with at least five replicates per treatment.
When DCMU (3‐(3,4‐dichlorophenyl)‐1,1‐dimethyl urea) was provided, cuttings were removed from the parent plant and placed vertically with their bases in vials of either water or an aqueous solution of 10−4 M DCMU for 5 d under low light. The cuttings were then potted into compost and watered with water or 10−4 M DCMU and kept vertical for 2 d before the start of the experiment.
Lazy‐2 tomato experiments
Tomato (Lycopersicon esculentum) were germinated and grown on filter paper in clear plastic boxes at 25 °C, initially in darkness. To expose to white light (12 μmol m−2 s−1), the boxes were rotated (2 rpm) horizontally between two Osram warm white fluorescent tubes to avoid any phototropic effects. DCMU (10−4 M) was applied to the germinated seedlings 12 h before the start of the experiment. Norflurazon (2×10−4 M) treatment was achieved by germinating and growing the seedlings in the presence of the herbicide. All experiments were repeated twice, with at least 10 replicates being used per treatment. For each hypocotyl, the angle of the most apical region with respect to the gravity vector was measured throughout the experiment.
Results
The effect of light on the GSA of Tradescantia
When cuttings of Tradescantia were grown in a range of fluence rates in a growth room the developmental progression of the GSA change of the individual nodes depended on the fluence rate (Fig. 1a). Light‐grown cuttings placed initially in a horizontal position in darkness bend upwards and within 5 d reach a GSA characteristic of a dark‐grown plant (Fig. 1b). Plants grown in light also show an initial upwards movement, but they then adjust their GSA at a rate that depends on the magnitude of illumination. At the highest fluence rate studied, some individuals begin to reduce their GSA after about 6 d. At the intermediate fluence rate the GSA decline begins a little later and at the lowest fluence rate plants eventually have a GSA that is not statistically significantly different from those in darkness. For any node after any interval the rank order for the average GSA value was: dark >12 μmol m−2 s−1>80 μmol m−2 s−1>120 μmol m−2 s−1. These effects of light were not directional: when horizontal shoots of Tradescantia were illuminated from below with white light (120 μmol m−2 s−1) they formed the same hanging habit as if the light was given from above (data not shown).
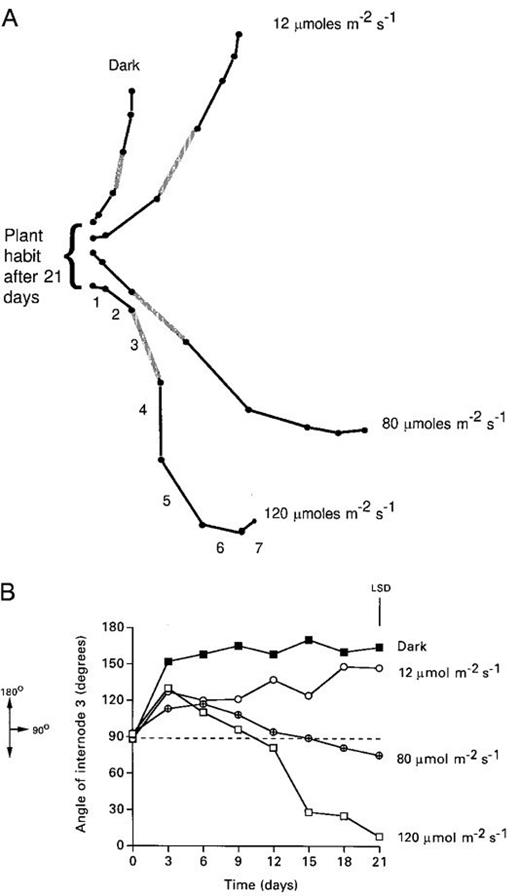
(a) Drawings of the orientation of the axes of individual nodes of a typical 21‐d‐old Tradescantia cutting grown under different light regimes. Node 1 is the oldest and is the anchoring point for each cutting. Node 3 for which data are presented is shown shaded. (b) The GSA of node 3 of Tradescantia cuttings is dependent on the fluence rate of white light. The data shown are for node 3 of individual plants that most closely matched the median value of the population on day 21. The LSD value shown applies to the means of the population on day 21 where ANOVA showed that there was a highly significant effect of light (P<0.001).
The effects of the photosynthetic inhibitor DCMU on the GSA of Tradescantia
Having shown that the GSA of an organ can be determined by the fluence rate of white light, the photoreceptor involved was probed. The fact that quite high fluence rates of white light were needed to allow the developmental progression of GSA to become evident suggested that the light effect was brought about by an effect on photosynthesis rather than an action via phytochrome. This deduction gained support from the finding that when Tradescantia cuttings were grown in the presence of the inhibitor of photosynthesis DCMU (10−4 M), a statistically significant effect on the gravitropic behaviour in the light was observed. After 21 d a light‐grown DCMU‐treated cutting had a similar form to a dark‐grown control cutting and was very different from the untreated control (Figs 1a, 2a). The fact that cuttings treated with DCMU showed an initial upward curvature at the start of the experiment demonstrates that DCMU had not influenced the ability of the plant to perceive or respond to gravity with differential elongation after gravitropic stimulation (Fig. 2b). This was confirmed with the observation that reorientation of DCMU‐treated cuttings after 21 d induced a new gravitropic response. DCMU applied as a foliar spray can also influence the GSA of cuttings. Cuttings were placed horizontally in the dark and were left until they had become vertical (as in Fig. 2b). At that time half the shoots were sprayed with 2×10−4 M DCMU and then all the plants were placed in high light (120 μmol m−2 s−1). During the next 10 d, the DCMU‐treated plants remained vertical while the control plants showed the decline in GSA similar to that reported in Fig. 1a and b.
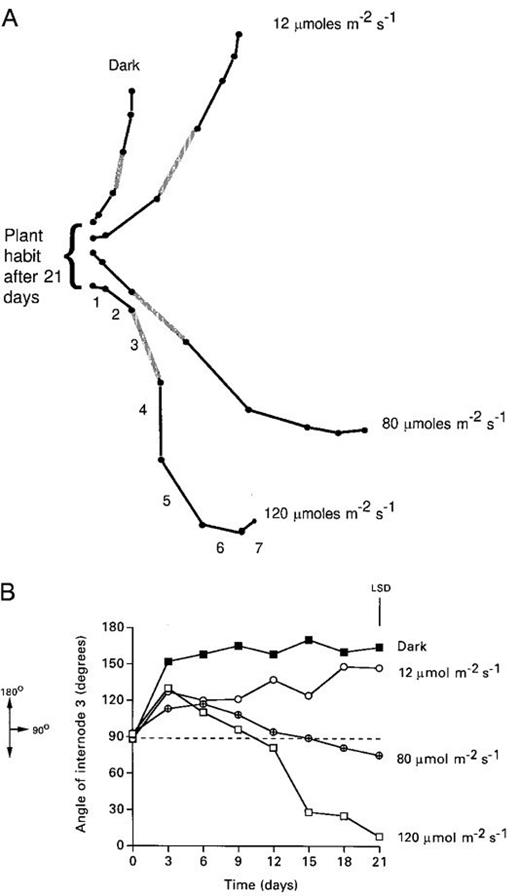
(a) Drawings of the orientation of the axes of individual nodes of Tradescantia cuttings grown with or without DCMU. (b) The photosynthetic inhibitor DCMU has an effect on the GSA of Tradescantia cuttings. The data shown are for individual plants that most closely matched the population median on day 21. Statistical analysis showed that the means of the DCMU and control populations (n=6) differed (P<0.001). The lack of effect of DCMU on the gravitropic response induced by displacement of node 3 away from its GSA after 21 d shows that DCMU does not influence gravity perception or the gravity‐induced differential growth.
The effect of low intensity red on the GSA in Tradescantia
Potted cuttings were grown vertically in dim white light (12 μmol m−2 s−1) for 7 d and then placed vertically either in the dark or in red light (15 μmol m−2 s−1). The nodes remained vertically upwards in both darkness and in red light (Fig. 3). When plants were reoriented to 90°, the dark‐grown and red‐light‐grown plants showed a similar upward gravitropic response: they had retained their high GSA values and were still capable of showing a gravitropic response.
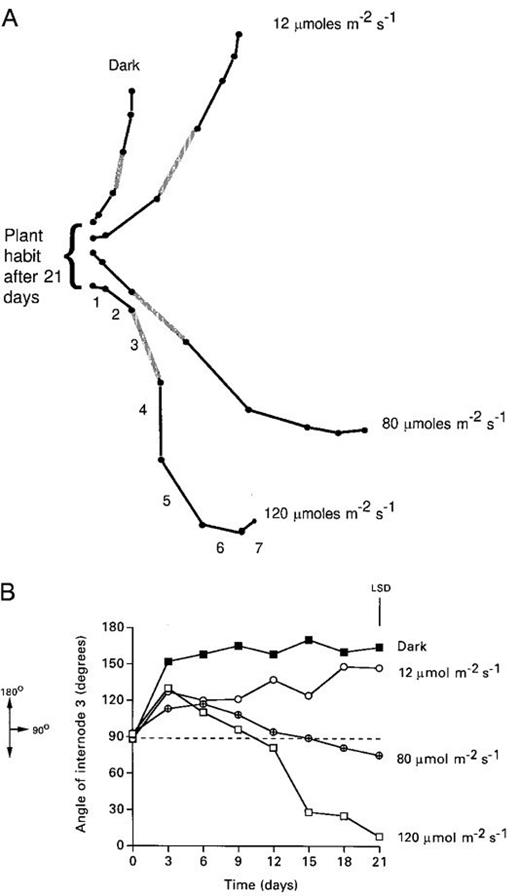
Exposure of Tradescantia cuttings to continuous red light (15 μmol m−2 s−1) has no statistically significant effect on the GSA of the nodes as illustrated by measurements of node 3. Each point is the mean of six replicates.
The effect of inhibiting photosynthesis on the GSA of lazy‐2 tomato
When dark‐grown seedings of the lazy‐2 tomato were placed vertically in white light (15 μmol m−2 s−1), they bend downwards to reach a GSA of 30–40° after 2 d. Seedlings treated with 10−4 M DCMU also bend downwards for the first 2 d but they then curve upwards to attain a mean GSA of 88±6° after 4 d compared to the mean of 48±6° for control seedlings (Fig. 4). DCMU had not abolished the ability of the tomato to sense or respond to gravity because 5 d after the start of the observations, when plants were reoriented upwards by 90°, it was found that the hypocotyls readjusted their orientation downwards during the next 24 h, showing a vigorous gravitropic response.
To support the conclusion that the effect of DCMU was caused by an action on photosynthesis, the photobleaching herbicide norflurazon was used to produce tomato seedlings which were lacking photosynthetic pigments, hence were incapable of photosynthesis. Seedlings treated with 2×10−4 M norflurazon (Fig. 5) showed a developmental change in their GSA which was similar to that shown in DCMU‐treated seedlings. Light exposure induced a significant reduction in GSA in both treated and control seedlings, but after 1 d exposure to light, the GSA of the norflurazon‐treated seedlings was always greater than that of the control seedlings. After 4 d the mean GSA of the norflurazon‐treated seedlings was 100±10° and the mean control GSA was 38±10°. Once again it could be demonstrated by reorientation experiments that norflurazon had not changed the ability of the seedlings to show a gravitropic response.
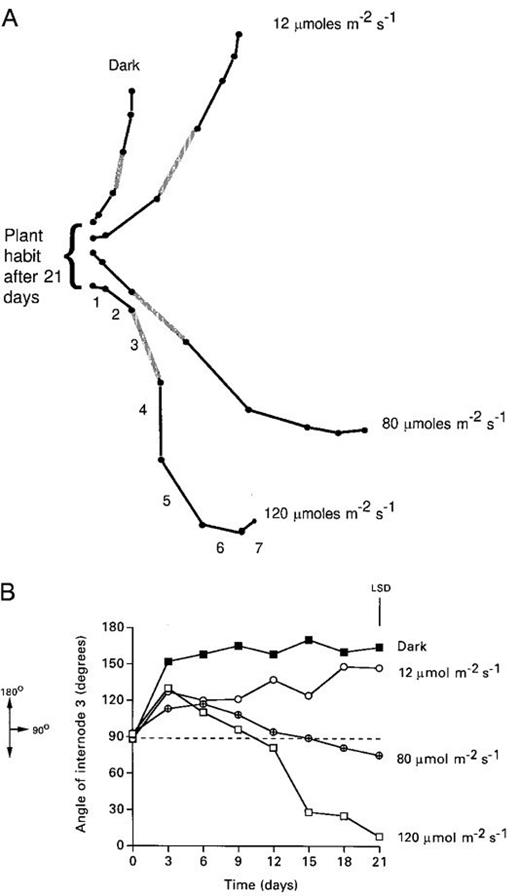
The photosynthetic inhibitor DCMU has a significant effect on the light‐induced GSA changes of lazy‐2 tomato hypocotyls. The data shown represent the behaviour of individual plants that most closely matched their population median on day 5. The population means (n=15) were significantly different (P<0.001) on days 3, 4, 5, 6.
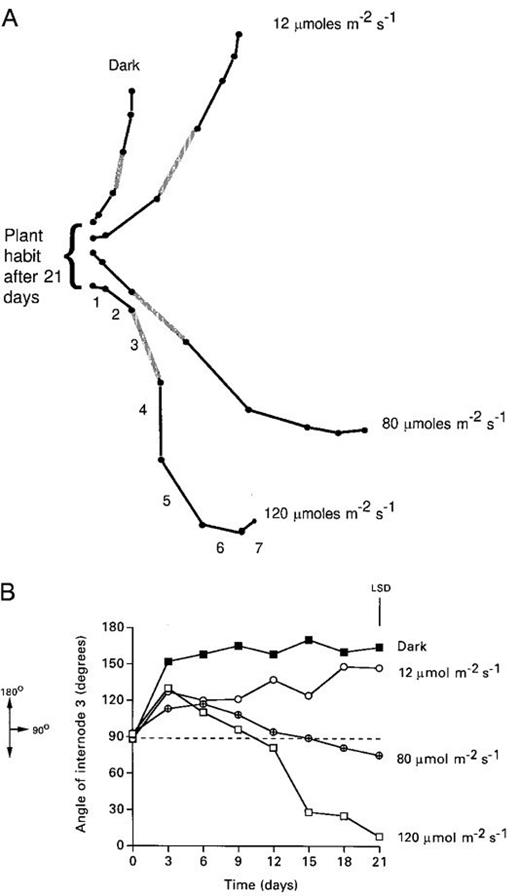
The photosynthetic inhibitor norflurazon has a significant effect on the light‐induced GSA changes of lazy‐2 tomato hypocotyls. The data shown represent the behaviour of individual plants that most closely matched their population median on day 5. The population means (n=8) were significantly different (P<0.001) after day 3.
Discussion
The work reported confirms that one of the ways in which light alters the gravitropic behaviour of plant organs is to change the GSA of an organ. There was already evidence in the literature that red light acting via phytochrome could influence the angle at which an organ was maintained (Tepfer and Bonnet, 1972; Mandoli et al., 1984; Feldman and Briggs, 1987; Liscum and Hangarter, 1993; Robson and Smith, 1996) and it is now possible to re‐interpret much of that evidence as supporting the view that phytochrome can influence the GSA of some organs (Firn and Digby, 1997). However, the present study has now demonstrated that photosynthesis can also influence the GSA of a plant organ. The relative importance of the photosynthetic effect on GSA and the effect mediated directly via phytochrome would seem to be variable and the balance of these controlling factors may depend on the species, the developmental history of the plant and the prevailing conditions. Under the conditions employed for this study, in Tradescantia the predominant effect of light on the GSA was via photosynthesis and there was also a very significant photosynthetic effect on the GSA of tomato hypocotyls. Previous studies of the effect of light on the gravitropic response of tomato have concentrated on effects exerted via phytochrome and may have chosen conditions where the photosynthetic effect was less pronounced.
Previous work concluded that the sucrose concentrations in organs can influence the gravitropic behaviour of organs (Montaldi, 1969; Willmoes et al., 1988) and it is proposed here that these photosynthetic effects arise from an alteration of the GSA of an organ. Whether the photosynthetic effect on the GSA is mediated by sucrose or by some other means will require further study. Given that it has recently been reported that sucrose can modify the expression of genes that are regulated by phytochrome A (Dijkwel et al., 1997) and that phytochrome A is involved in the effect of red light on the GSA in Arabidopsis, it is possible that light mediates its effects on the GSA of some organs by a combined effect via photosynthesis and via phytochrome.
The fact that sucrose concentrations in some compartment in an organ could influence the GSA of that organ should now be taken into consideration in the design and interpretation of gravitropism experiments. For example, many experiments have been conducted on gravitropic sensing, where the starch levels have been manipulated experimentally or genetically in order to influence the size of the supposed gravity sensors (starch statoliths). In such experiments it is usually assumed that any effect observed is a consequence of an effect on the amyloplasts. It is now evident that such experimental manipulations may have produced unforeseen effects on the GSA of the organs via the manipulated sucrose levels, consequently there may have been confounding effects on the gravitropic response of the organs independent of any effect mediated via the amyloplasts and gravisensing.
To whom correspondence should be addressed. Fax: +44 (0)1904 432860. E‐mail: jd15@york.ac.uk
We wish to thank Professor J Roberts, University of Nottingham, Sutton Bonington, for providing seeds of the lazy‐2 tomato.
References
Behringer FJ, Lomax TL.
Beltrano J, Willmoes J, Montaldi ER, Barreiro R.
Czapek F.
De Vries
Dijkwel PP, Huijser C, Weisbeck PJ, Chua N, Smeekens SCM.
Digby J, Firn RD.
Firn RD, Digby J.
Feldman LJ, Briggs WR.
Gaiser CJ, Lomax TL.
Langham DE.
Liscum E, Hangarter RP.
Maige MB.
Mandoli DF, Tepperman J, Huala E, Briggs WR.
Montaldi ER.
Myers AB, Firn RD, Digby J.
Palmer JH.
Roberts JA.
Robson PRH, Smith H.
Tepfer DA, Bonnet HT.
Willmoes J, Beltrano J, Montaldi ER.
Willmoes J, Beltrano J, Montaldi ER.
Comments