-
PDF
- Split View
-
Views
-
Cite
Cite
M.A. Hannah, M.J. Iqbal, F.E. Sanders, Adaptation to long‐term cold‐girdling in genotypes of common bean (Phaseolus vulgaris L.), Journal of Experimental Botany, Volume 52, Issue 358, 1 May 2001, Pages 1123–1127, https://doi.org/10.1093/jexbot/52.358.1123
- Share Icon Share
Abstract
The effect of long‐term cold‐girdling on phloem transport and resource allocation in whole plants of common bean is described. Wide differences were found between genotypes, with some maintaining translocation when cold‐girdled. This provides evidence to support passive phloem transport. The possibilities that cold‐girdling may physically block transport and/or disrupt root–shoot signalling are discussed.
Introduction
Understanding of phloem transport remains an elusive goal despite a century of research. There has been considerable debate surrounding possible mechanisms, particularly regarding the involvement of energy in maintaining phloem transport. In spite of its shortcomings, the pressure‐driven mass flow hypothesis still remains the most credible.
It is well known that phloem transport can be inhibited by cooling sections of stem or petiole (Geiger and Sovonick, 1975, and references therein). The main features of this inhibition are that it depends on the rate of cooling and can be caused by only slight drops in temperature (Minchin and Thorpe, 1983), is reversible upon re‐warming (Minchin et al., 1983) and that a plant may acclimatize to either successive cooling cycles (Lang and Minchin, 1986) or within an extended cooling period (Swanson and Geiger, 1967, and references therein). It has been suggested that a blockage of the phloem may arise from the occlusion of sieve plate pores (Giaquinta and Geiger, 1973). The presence of an apoplastic assimilate pool in common bean (Phaseolus vulgaris L.) supports the hypothesis that the phloem pathway is leaky with passive unloading and active reloading (Minchin et al., 1984). Phloem translocation could thus be inhibited by cooling due to its effect on reloading (Lang and Minchin, 1986).
Inhibition of phloem transport by cooling has been particularly well investigated in P. vulgaris. Experiments on the responses of P. vulgaris and other species to cold‐girdling have tended to be of short duration (<24 h) and have focused on the movement of assimilate within the shoot. The experimental systems have mostly been simplified, with shoots often being isolated from the root system by a steam girdle. In this paper, the effect of long‐term cold‐girdling on phloem transport and resource allocation in whole plants of different P. vulgaris genotypes is described.
Materials and methods
Plant material
Two cultivars of P. vulgaris cv. Carioca (CNF 0554) and cv. Prelude (commercially available) were used in all experiments as reference genotypes. Other genotypes were obtained from commercial sources or selected (F5) from progeny of a cross between Carioca (female parent) and cv. Prince.
Plants were placed in tap water in 50 l hydroponic tanks (20 plants per tank) shortly after the hypocotyl had straightened. Standard nutrient stock solutions were added (1 ml stocks l−1 to give 1/10 of full strength complete nutrient solution (Long Ashton Formula; Hewitt, 1966)), with further additions to maintain solution conductivity at 600–800 μS for the duration of the experiments. All tanks were kept in a glasshouse at around 25 °C (range 18–33 °C). When necessary, natural daylight was supplemented to provide a 16 h photoperiod.
Seedlings of eight genotypes with five replicates were randomly arranged across four tanks. Cold‐girdled and non‐girdled control plants were in alternating rows of five, with two rows of each per tank. Plants for an initial harvest were randomly arranged in two separate tanks.
Cold‐girdling of stems
Cold‐girdling was started when the first trifoliolate leaves were visible (c. 15–20‐d‐old). Cotyledons were removed from all plants at the start of cold‐girdling.
Plants were cold‐girdled c. 1 cm below the cotyledonary node. Cooling jackets made from 5 cm sections of 1 cm internal diameter plastic tubing were fitted and sealed around the stems, and at right angles to them, so that the cooled regions were 1 cm long. Non‐toxic silicon sealant (Dow Corning 744) provided watertight seals. The five sections of plastic tubing in each row were joined by larger diameter plastic tubing. The eight rows were joined in parallel by equal lengths of branched pipework to ensure uniform water flow. Cold water (1–1.5 °C) was circulated (4.0 l min−1 total) through the tubing from a large reservoir for 1 week. All pipes were insulated.
Harvesting of plant material
There were two harvests, an initial harvest (H0) at the start of cold‐girdling and a final harvest (H1) after 1 week. Plants were separated into four component parts; stem+petioles, unifoliolate leaves, trifoliolate leaves and roots. These parts were blotted dry and individual fresh masses measured. Leaf areas were measured with a leaf area meter. All plant parts were dried in a fan oven at 80 °C for at least 24 h before dry masses were measured.
Starch staining
Plants were harvested, roots and leaves removed and the stems placed in methanol to remove chlorophyll. Stems were stained for 24 h in aqueous iodine solution (2% (w/v) potassium iodide, 0.2% (w/v) iodine), washed in distilled water and photographed.
Data analysis
Root, leaf and stem fractions, specific leaf areas (SLA), leaf area ratios (LAR), and proportions of dry mass to fresh mass (% DM) were calculated. Allocation fractions were calculated as the mean changes in dry mass of each plant part as proportions of the mean changes in total plant dry mass (negative allocation fraction means part loses mass). Standard errors (SE) for allocation fractions were calculated, as described previously (Hannah et al., 2000), on ranked data by assuming a relation between plant masses at H0 and H1.
Results
There was a large difference between the responses of Carioca and Prelude to long‐term cold‐girdling. In Prelude, root growth was completely stopped with negative mass allocation to the root system in all cooling experiments (Fig. 1a–c). Root allocation fraction was found to be much less affected by cold‐girdling in Carioca with an average reduction of 25% and a range of 6–43% (Fig. 1a–c).
These responses were unlikely to be due to the presence of the cold‐girdling apparatus itself as an initial experiment circulating water at room temperature (control plants) and 1–2 °C (cooled plants) showed reductions in root allocation fractions for cooled plants (23%, Ca; 120%, Pd) not significantly different from those in subsequent experiments where water was not circulated past the uncooled controls.
The consistency of the cold‐girdling response is supported by results from the genotypes 59/8/b and 38/2/b with c. 50% and c. 60% reductions in root allocation fraction in experiment one and c. 40% and c. 50% in experiment two respectively (Fig. 1a, b).
There was a large variation in the spectrum of responses shown by the genotypes tested, but these were generally between the extremes shown by Prelude and Carioca. There were five genotypes including Prelude which showed a reduction in root allocation fraction of c. 90% or greater, with three of these >100% (Fig. 1a, b). Most of the genotypes tested showed a reduction in root allocation fraction of between c. 40–60% (Fig. 1a–c). There were five genotypes which were relatively unaffected by cold‐girdling; Carioca, Carioca (Itiuba), B7, GLPX 1281 and 2/4/11, which all showed a reduction in root allocation fraction of less than c. 30% (Fig. 1b, c).
The progeny of the Carioca×Prince cross showed a wide range of responses to cold‐girdling. If Carioca is used as a common reference between experiments then the following patterns can be seen. Genotypes 53/11/b, 40/3/9, 63/12/b, and 2/4/5 were clearly much more susceptible to cold‐girdling than either parent (Fig. 1a, b). Root allocation fraction was less affected in the line 2/4/11 than on average in Carioca (Fig. 1b). The other genotypes were similar to their parents in their responses (Fig. 1a, b).
In contrast to root allocation the only significant effects (t‐test, P<0.05) on total plant dry mass were reductions of c. 20–30% for Prelude (one trial only), 40/3/9 and 13/12/10 (data not shown).
Iodine staining indicated that starch accumulated above the girdle of cold‐girdled Prelude (Fig. 2). This accumulation of carbohydrate is likely to have caused the 75% increase in the %DM in the shoot observed in Prelude (data not shown). Leaf expansion was reduced in Prelude, which combined with the high %DM produced large decreases in SLA and LAR (data not shown). In striking contrast there was no starch accumulation in Carioca (Fig. 2) and no significant changes in %DM, SLA or LAR (data not shown).
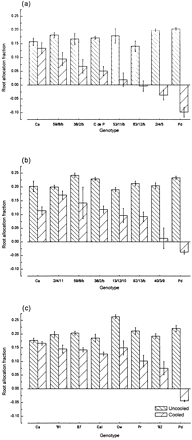
Root allocation fractions for cold‐girdled and non‐girdled P. vulgaris plants in three experiments. (a) Eight genotypes: Prelude (Pd), Carioca (Ca), Coco de Prague (C de P) and five genotypes from a Carioca×Prince cross (63/12/b, 59/8/b, 38/2/b, 2/4/5, 53/11/b). (b) Eight genotypes: Prelude (Pd), Carioca (Ca) and six genotypes from a Carioca×Prince cross (40/3/9, 92/13/b, 59/8/b, 38/2/b, 2/4/11, 13/12/10). (c) Eight genotypes: Prelude (Pd), Carioca (Ca), Canadian Wonder (Cw), Prince (Pr), Carioca (Itiuba) (CaI), GLPX 1281 (‘81) GLPX 192 (‘92), and 1 line from a Carioca×Prince cross (B7). Data are means±SE (mostly n=5).
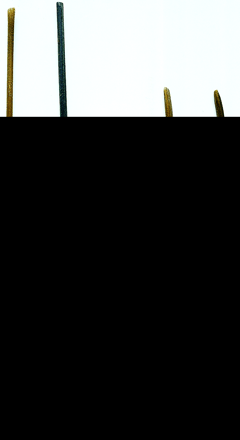
Starch distribution (dark areas), as revealed by iodine staining, for cold‐girdled and non‐girdled Prelude (Pd) and Carioca (Ca) plants. Arrows indicate upper and lower extents of cold‐girdle.
Discussion
These data show that there are wide differences between the susceptibility to long‐term cold‐girdling of P. vulgaris genotypes. The greatest difference in response was between Prelude and Carioca. Long‐term stem cold‐girdling always resulted in a negative root allocation fraction in Prelude, whilst Carioca was much less affected (Fig. 1a–c). The climatic origins of these cultivars, Andean and Mesoamerican, respectively, might have led to the opposite expectation.
It has been shown that rapidly cooling a stem or petiole section to below 10 °C almost immediately reduces translocation with total stoppage after 1 h (Minchin et al., 1983). Given the low temperature (1–1.5 °C) and rapid cooling in the experiments, an initial complete blockage of phloem translocation in all genotypes is almost certain. Starch distribution (Fig. 2) and reductions in root mass (up to 46%, data not shown) in cold‐girdled Prelude show that negative root allocation (meaning roots lose mass) was due to a blockage of phloem transport (to a level where transport was not detectable in this system).
It is likely that, in the short‐term, dry mass accumulation in a sink can continue due to buffering from the apoplastic assimilate pool (Minchin et al., 1983). Considering the estimated size of the apparent free space (4%) and the concentration of sucrose (25–60 mol m−3) in the stem of bean (Minchin and Thorpe, 1984), it is unrealistic to discuss the changes in root dry mass observed here (50–200 mg, data not shown) in terms of differences in the size of the apoplastic storage pool. It is more likely that the differences are due to different adaptations to an initial blockage of translocation. Such a blockage may arise from the occlusion of sieve plate pores (Giaquinta and Geiger, 1973). This could arise from callose deposition or the displacement of cytoplasmic material or p‐protein (Giaquinta and Geiger, 1973; Pickard and Minchin, 1990, 1992).
Alternatively, the presence of a stem apoplastic assimilate pool in P. vulgaris and responses shown to short‐term cold‐girdling have been used to support the hypothesis that active reloading of the phloem is inhibited (Lang and Minchin, 1986). Full recovery of transport would be expected once passive unloading was inhibited by rising sucrose concentration in the surrounding apoplast of the cooled region (Lang and Minchin, 1986). Continued large reductions of transport in some genotypes do not fit well to this model. If sucrose could be removed from the apoplast of the cooled region by the synthesis of storage carbohydrates in adjacent cells this could lead to long‐term inhibition of phloem transport, but since substantial accumulation of starch could not be detected in the cooled region of Prelude (Fig. 2) this also seems unlikely.
Another possibility not previously discussed, is the interruption by cold‐girdling of transport of an active signal affecting sink activity or the transport process itself. The DL gene system in P. vulgaris is thought to be important in the control of root–shoot partitioning through the interaction of alleles at loci expressed in the root and shoot, and there may be signalling incompatibilities between DL genotypes (Hannah et al., 2000). It has been suggested that these signalling mechanisms may influence root–shoot allocation by influencing C translocation (Hannah et al., 2000). Carioca and Prelude have different DL genotypes and it was originally hypothesized that this difference might be responsible for their different susceptibility to cooling. If signal compounds involved in the DL system were transported actively then cold‐girdling could affect root–shoot partitioning by interfering with transport of these signals. The DL genotypes of the other plant material used in these experiments were determined using the method described earlier (Hannah et al., 2000). In fact, there appears not to be any strong relationship between response to cooling and DL genotype although no definite conclusions can be made as yet (data not shown). As the results from iodine staining (Fig. 2) indicate that reduced transport to the root results from a local effect on phloem transport, it seems unlikely that cold‐girdling is interfering with the DL signalling system unless the latter is directly influencing the transport process itself.
There are examples of resumption of translocation with prolonged cold treatment (Swanson and Geiger, 1967, and references therein; Geiger and Sovonick, 1975), but these investigations have tended to use shorter timescales, simplified source–sink systems and chilling‐insensitive species. The ability of some genotypes of bean, a chilling‐sensitive species, to maintain normal root–shoot allocation despite having a stem section cooled for long periods to 1–1.5 °C provides further good evidence that the flow of assimilate along the phloem may not itself be an active process.
In summary, it has been confirmed that cold‐girdling can inhibit translocation in bean and shown that the effect is genotype‐dependent. These results are most consistent with cold‐induced occlusion of the sieve plate pores. The ability to overcome such blockage may therefore vary greatly in magnitude between different genotypes. The capacity of some genotypes to maintain phloem transport, despite a long‐term cold block, provides further evidence that flow along the phloem pathway itself may not be an active process.
To whom correspondence should be addressed. Fax: +44 113 233 2835. E‐mail: [email protected]
We wish to thank the University of Leeds and Government of Pakistan (MJI) for financial support, D Hardy and M Lappage for technical assistance and Dr LD Incoll and Dr DJ Pilbeam for valuable comments on the manuscript.
References
Geiger DR, Sovonick SA.
Giaquinta RT, Geiger DR.
Hannah MA, Iqbal MJ, Sanders FE.
Hewitt EJ.
Lang A, Minchin PEH.
Minchin PEH, Lang A, Thorpe MR.
Minchin PEH, Ryan KG, Thorpe MR.
Minchin PEH, Thorpe MR.
Minchin PEH, Thorpe MR.
Pickard WF, Minchin PEH.
Pickard WF, Minchin PEH.
Comments