-
PDF
- Split View
-
Views
-
Cite
Cite
Makoto Sasaki, Mitsuhiro Nakamura, Ryo Ashida, Manabu Nakata, Michio Yoshimura, Takashi Mizowaki, Assessing target localization accuracy across different soft-tissue matching protocols using end-exhalation breath-hold cone-beam computed tomography in patients with pancreatic cancer, Journal of Radiation Research, Volume 64, Issue 4, July 2023, Pages 711–719, https://doi.org/10.1093/jrr/rrad048
- Share Icon Share
Abstract
The purpose of this study was to retrospectively assess target localization accuracy across different soft-tissue matching protocols using cone-beam computed tomography (CBCT) in a large sample of patients with pancreatic cancer and to estimate the optimal margin size for each protocol. Fifty-four consecutive patients with pancreatic cancer who underwent 15-fraction volumetric modulated arc therapy under the end-exhalation breath-hold condition were enrolled. Two soft-tissue matching protocols were used according to the resectability classification, including gross tumor volume (GTV) matching for potentially resectable tumors and planning target volume (PTV) matching for borderline resectable or unresectable tumors. The tolerance of the target localization error in both matching protocols was set to 5 mm in any direction. The optimal margin size for each soft-tissue matching protocol was calculated from the systematic and random errors of the inter- and intrafraction positional variations using the van Herk formula. The inter- and intrafraction positional variations of PTV matching were smaller than those of GTV matching. The percentage of target localization errors exceeding 5 mm in the first CBCT scan of each fraction in the superior–inferior direction was 12.6 and 4.8% for GTV and PTV matching, respectively. The optimal margin sizes for GTV and PTV matching were 3.7 and 2.7, 5.4 and 4.1 and 3.9 and 3.0 mm in the anterior–posterior, superior–inferior and left–right directions, respectively. Target localization accuracy in PTV matching was higher than that in GTV matching. By setting the tolerance of the target localization error, treatment can be successful within the planned margin size.
INTRODUCTION
Pancreatic cancer is one of the most aggressive diseases, with a 5-year overall survival rate of <10%, and it is the fourth leading cause of cancer-related death in both men and women in Europe and the USA [1, 2]. Although surgical resection can lead to promising outcomes in terms of the long-term survival of patients with pancreatic cancer, 80% of patients present with unresectable (UR) pancreatic cancer at the time of diagnosis, owing to locally advanced disease and distant metastases [3].
For patients with UR pancreatic cancer, chemoradiotherapy (CRT) is recommended [4]. Recently, neoadjuvant CRT has been shown to be effective in patients with borderline resectable (BR) pancreatic cancer [5]. Moreover, a phase II clinical trial investigating neoadjuvant CRT for potentially resectable (PR) pancreatic cancer is ongoing [6]. According to ASTRO guidelines, conventionally fractionated radiotherapy (RT) or stereotactic body radiotherapy (SBRT) is recommended for patients with pancreatic cancer [7]. Regarding the image guidance methods, target matching based on fiducial markers is often used in SBRT [8–11]. However, a disadvantage of using fiducial markers is the invasiveness. By contrast, conventionally fractionated RT is still used in many institutions without fiducial markers. Due to several factors, it is difficult to deliver a definitive dose in the treatment of pancreatic cancer. First, the pancreas is surrounded by radiosensitive organs at risk (OARs), such as the stomach, duodenum, small bowel, large bowel, liver, kidneys and spinal cord. Second, the pancreas and adjacent OARs have large inter- and intrafractional variations [12–14]. If motion management techniques are not applied, the irradiation volume increases, potentially increasing toxicity in normal tissues.
To reduce the irradiation volume, the breath-hold (BH) technique is commonly used owing to its versatility [15]. When applying the BH technique in patients with pancreatic cancer, highly accurate target localization is essential; thus, cone-beam computed tomography (CBCT) has played an important role in this context [16–18].
At our institution, target localization in patients with pancreatic cancer has conventionally been determined via soft-tissue matching based on the orientation of the target, the location of OARs and the presence of normal tissues within the planning target volume (PTV) using end-exhalation (EE)-BH CBCT in image-guided radiotherapy (IGRT) [19, 20]. Recently, different protocols for soft-tissue matching have been employed to improve treatment outcomes according to the resectability classification described in clinical practice guidelines [21]. For example, stricter regulations in location should be applied when conducting target localization for patients with PR cancer than for patients with UR or BR cancer; in other words, matching focuses on local regions containing the gross tumor volume (GTV). Optimal matching with a focus on the target is preferable for delivering a prescribed dose as intended. However, the effect of different soft-tissue matching protocols on target localization accuracy has not been assessed.
Therefore, this study aimed to retrospectively assess the target localization accuracy across different soft-tissue matching protocols using CBCT in a large cohort of patients with pancreatic cancer and to determine the optimal margin size for each protocol.
MATERIALS AND METHODS
Patients
Data from 54 consecutive patients with pancreatic cancer who underwent 15-fraction volumetric modulated arc therapy (VMAT) under the EE-BH condition between July 2019 and July 2021 were retrospectively analyzed. Patient characteristics are summarized in Table 1. The prescribed dose and region of interest for soft-tissue matching using CBCT were determined according to the resectability classification. All patients fasted for >3 h and discontinued oral intake of water 1 h before treatment planning computed tomography (pCT) and daily treatment. All patients provided written informed consent. This study was approved by our institutional review board (approval number: P1440) and is adherent to the EQUATOR Network’s research reporting guidelines.
Resectability classification . | Number of patients (with biliary stent) . | Sex, male:female . | Median age (range) . | Location of pancreas, head:body:tail . | Prescribed dose . | Region of interest at soft-tissue matching . |
---|---|---|---|---|---|---|
PR | 18 (2) | 9:9 | 69 (48–77) | 7:7:4 | 45 Gy/15 fr. to cover 50% of GTV and 42 Gy/15 fr. to cover 95% of PTV | Local area containing GTV |
BR | 27 (6) | 17:10 | 70 (32–79) | 18:9:0 | 42 Gy/15 fr. to cover 95% of PTV | Within the margin of PTV |
UR | 9 (0) | 4:5 | 61 (54–77) | 5:4:0 | 48 Gy/15 fr. to cover 95% of PTV | Within the margin of PTV |
Resectability classification . | Number of patients (with biliary stent) . | Sex, male:female . | Median age (range) . | Location of pancreas, head:body:tail . | Prescribed dose . | Region of interest at soft-tissue matching . |
---|---|---|---|---|---|---|
PR | 18 (2) | 9:9 | 69 (48–77) | 7:7:4 | 45 Gy/15 fr. to cover 50% of GTV and 42 Gy/15 fr. to cover 95% of PTV | Local area containing GTV |
BR | 27 (6) | 17:10 | 70 (32–79) | 18:9:0 | 42 Gy/15 fr. to cover 95% of PTV | Within the margin of PTV |
UR | 9 (0) | 4:5 | 61 (54–77) | 5:4:0 | 48 Gy/15 fr. to cover 95% of PTV | Within the margin of PTV |
Resectability classification . | Number of patients (with biliary stent) . | Sex, male:female . | Median age (range) . | Location of pancreas, head:body:tail . | Prescribed dose . | Region of interest at soft-tissue matching . |
---|---|---|---|---|---|---|
PR | 18 (2) | 9:9 | 69 (48–77) | 7:7:4 | 45 Gy/15 fr. to cover 50% of GTV and 42 Gy/15 fr. to cover 95% of PTV | Local area containing GTV |
BR | 27 (6) | 17:10 | 70 (32–79) | 18:9:0 | 42 Gy/15 fr. to cover 95% of PTV | Within the margin of PTV |
UR | 9 (0) | 4:5 | 61 (54–77) | 5:4:0 | 48 Gy/15 fr. to cover 95% of PTV | Within the margin of PTV |
Resectability classification . | Number of patients (with biliary stent) . | Sex, male:female . | Median age (range) . | Location of pancreas, head:body:tail . | Prescribed dose . | Region of interest at soft-tissue matching . |
---|---|---|---|---|---|---|
PR | 18 (2) | 9:9 | 69 (48–77) | 7:7:4 | 45 Gy/15 fr. to cover 50% of GTV and 42 Gy/15 fr. to cover 95% of PTV | Local area containing GTV |
BR | 27 (6) | 17:10 | 70 (32–79) | 18:9:0 | 42 Gy/15 fr. to cover 95% of PTV | Within the margin of PTV |
UR | 9 (0) | 4:5 | 61 (54–77) | 5:4:0 | 48 Gy/15 fr. to cover 95% of PTV | Within the margin of PTV |
Treatment pCT
An immobilization system was individually prepared for each patient using a vacuum pillow (Body Fix system; Elekta, Stockholm, Sweden), with patients in the supine position and their arms raised. The Real-time Position Management system (RPM; Varian Medical Systems, Palo Alto, CA, USA) was used as the respiratory management system. Before treatment pCT, patients underwent BH training several times according to our institutional BH protocol; briefly, an operator instructed the patients to ‘breathe in and breathe out’ while monitoring their respiratory signals using the RPM system and to ‘hold the breath’ during the EE condition. No visual feedback was provided for patients in our institution.
The pCT scans with and without contrast enhancement were performed under the EE-BH condition using a 64-slice computed tomography (CT) scanner (SOMATOM Definition AS; Siemens Healthineers, Erlangen, Germany). The CT scans were acquired at 120 kVp, with a slice thickness and slice interval of 2.0 mm and a field of view of 500 mm; they ranged from the superior border of the liver to the iliac crest, and imaging took 10 s to complete. The contrast-enhanced CT was performed 40 s after injection.
Treatment planning
The GTV and clinical target volume (CTV) were determined from non-contrast CT images imported into the radiation treatment planning system (Eclipse version 15.6; Varian Medical Systems) by radiation oncologists. The CTV was defined as the area of the GTV plus a 5-mm margin. The retro-pancreatic space and para-aortic lymph nodes between the root of the celiac trunk and superior mesenteric artery were also included in the CTV. The CTV definition was the same regardless of the resectability classification. The PTV was set by adding a 5-mm isotropic margin to the CTV. Contouring of OARs, including the stomach, duodenum, intestine, liver, kidneys and spinal cord, was carried out by medical physicists, and the planning OAR volume (PRV) was determined by adding 5–10-mm isotropic margins to the stomach, duodenum and spinal cord. The plan details including the dose–volume constraints have been previously described [20].
A single full-arc coplanar VMAT plan was created using the TrueBeam STx system (Varian Medical Systems) for all patients. Patients with BR and UR cancer were prescribed doses of 42 and 48 Gy, respectively, in 15 fractions to cover 95% of the PTV (subtracting the overlapping part of the PRVs) and ≥36 Gy to cover 98% of the PTV simultaneously. Patients with PR cancer were prescribed a dose of 45 Gy in 15 fractions to cover 50% of the GTV (subtracting the overlapping part of the PRVs), 42 Gy to cover 95% of the PTV (subtracting the overlapping part of the PRVs) and ≥ 36 Gy to cover 98% of the PTV simultaneously.
Daily workflow of IGRT for patients with pancreatic cancer
First, patients aligned with skin markers and wall-mounted lasers. An orthogonal kV X-ray imaging system (ExacTrac X-ray system, version 6.2.1; Brainlab, Feldkirchen, Germany) was then used to correct the initial 3D setup errors based on bony structures. The couch correction in six degrees of freedom was not applied in ExacTrac because the CBCT system in our institution (TrueBeam STx) was not able to calculate the errors of the Roll and Pitch angles. Therefore, if the rotational error in any angle exceeded 3° with ExacTrac, the re-setup of patients was carried out manually. After a 3D-couch shift, the first EE-BH CBCT scan on each treatment day was acquired at a rotation angle of 200° according to our institutional BH protocol. Acquisition of the EE-BH CBCT scan is typically interrupted once or twice depending on the patient’s BH ability, because the scanning lasts for ~40 s. An iterative reconstruction algorithm was used to reconstruct the CBCT images.
A radiation therapist (RTT) performed the soft-tissue matching using EE-BH CBCT. One of two protocols for soft-tissue matching was selected in each case according to the resectability classification. For patients with PR cancer, the RTTs performed soft-tissue matching manually based on the GTV; however, the GTV visibility was often poor because CBCT was inferior to pCT in terms of the low-contrast resolution. In these cases, the RTT performed matching by referring to the boundaries with surrounding soft tissues, such as the stomach, duodenum and abdominal fat. Since the matching in these cases mostly focused on the GTV, this process was defined as GTV matching. The target localization error in the GTV matching was represented by the anterior–posterior (AP), superior–inferior (SI) and left–right (LR) directions and was defined as the difference in the localization of the GTV between the pCT and CBCT scans. The protocol for soft-tissue matching in patients with BR and UR cancer differed from that in those with PR cancer in that the matching predominantly focused on OARs and the surrounding normal tissues, such as major vessels and abdominal fat; this process was defined as PTV matching. In such cases, as a result, the GTV was contained within the PTV, and it is important to note that the matching does not necessarily need to perfectly match the GTV. The target localization error in the PTV matching represented the amount of couch shift required for structures within the PTV to be mostly similar, visually, between the pCT and CBCT imaging after bone matching with the ExacTrac system. The daily target localization error was determined by consensus between two RTTs. The schemas for the two protocols for soft-tissue matching are shown in Fig. 1.
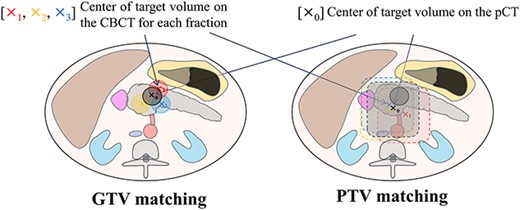
Schema for the two soft-tissue matching protocols. The x0 indicate the center of the target volume on pCT. X1, X2 and X3 mark the center of the target volume on the CBCT scan for each fraction, respectively. GTV matching is the local area matching based on the GTV and/or the boundaries with surrounding soft tissues such as the stomach, duodenum and abdominal fat. PTV matching is the matching predominantly focused on OARs and the normal tissues, such as major vessels and abdominal fat.
The tolerance of the target localization error at our institution, regardless of the protocol used for soft-tissue matching, was defined as a difference of 5 mm between the pCT and CBCT imaging in any direction after bone matching, which is equivalent to the PTV margins. The treatment beam was delivered without couch shifts after confirming that the target localization error on the first CBCT scan did not exceed the tolerance in any direction. If the target localization error exceeded the tolerance in any direction, the couch was shifted. Subsequently, the second CBCT scan under the EE-BH condition was acquired for verification, and soft-tissue matching was performed again. When the target localization error exceeded the tolerance on the second CBCT scan, the couch was shifted again, and a third CBCT scan was acquired. Couch correction and acquisition of the CBCT scans were repeated until the target localization error was within the tolerance. Finally, the CBCT scan that was within the tolerance of the target localization error just before treatment was defined as the last CBCT scan. A detailed workflow of IGRT for patients with pancreatic cancer is shown in Fig. 2.
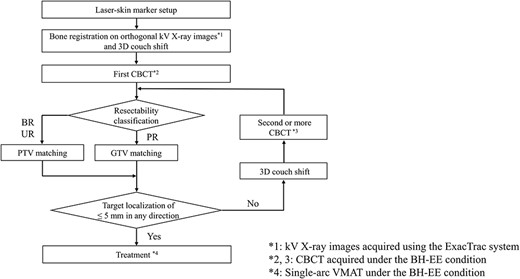
Daily workflow of IGRT for patients with pancreatic cancer. The kV X-ray images were acquired using the ExacTrac system. CBCT was acquired under the BH-EE condition. Soft-tissue matching. Treatment was performed via single-arc VMAT under the BH-EE condition.
Preliminary experiment; verification of differences in the IGRT experience of RTTs for the different protocols for soft-tissue matching
Our institution has frequently employed many RTT trainees. Thus, to evaluate observer variations in the two different protocols for soft-tissue matching, a preliminary experiment that included 11 RTTs was conducted. There were 5 coaches with a median of 10 years of IGRT experience (range, 6–15 years) and 6 trainees with a median of 3.5 months of IGRT experience (range, 3–5 months). The accreditation criteria for coaches require individuals to demonstrate aptitude in 90% of the institution-specific skills from a checklist, which consists of 30 items that assess clinical skills. Every trainee was always paired with a coach in clinical situations.
Among the 54 patients, the datasets from the first CBCT scans of 10 patients (5 with PR cancer and 5 with BR cancer) who enrolled early in the observation periods were used for the analysis of the preliminary experiment. The first CBCT scans in 3 of the 15 fractions were randomly selected for each patient. The target localization error between the pCT and first CBCT scan obtained from the matching of a board-certified radiation oncologist (R.A.) was determined as a criterion. Subsequently, RTTs retrospectively performed soft-tissue matching using ARIA Offline Review (Varian Medical Systems, Palo Alto, CA, USA), following the clinical procedures, without any special training. After >3 months, the same six trainees conducted a second series of soft-tissue matching for the same cases. The difference in the target localization error between the reference and that of each RTT was calculated, and the difference in the 3D observer variations between the coaches and trainees was compared for the GTV and PTV matching protocols. The 3D observer variations were calculated from the root sum squares in each direction. The Steel–Dwass multiple comparison test was used for statistical analysis. The level of significance was set at 0.05.
Inter- and intrafraction positional variations
The interfraction positional variation for each patient was calculated from the difference in target localization between the pCT and last CBCT scan on each treatment day. The mean and standard deviation (SD) of the interfraction positional variation were evaluated. The intrafraction positional variation in this study was defined as the difference in target localization between the (i + 1)th and ith CBCT in cases involving the acquisition of more than two CBCT scans on each treatment day. Moreover, the population systematic error (∑) and random error (σ) in each protocol for soft-tissue matching were calculated for each direction.
The systematic error of the interfraction positional variation (∑inter) was defined as the SD of the individual mean interfraction positional variation. The random error of the interfraction positional variation (σinter) was determined by the root mean square of the SD of each patient’s interfraction positional variation.
The systematic error of the intrafraction positional variation (∑intra) was defined as the mean intrafraction positional variation among all patients as acquired from the second or later CBCT scans. The random error of the intrafraction positional variation (σintra) was determined by the SD of the intrafraction positional variation among all patients as acquired from the second or later CBCT scans.
The total systematic error (∑tot) was determined by the root sum square of ∑inter and ∑intra, and the total random error (σtot) was determined by the root sum square of σintra and σintra.
The optimal margin size according to each soft-tissue matching protocol was calculated from the systematic and random errors of the interfraction and intrafraction positional variations using the van Herk formula [22].
RESULTS
Preliminary experiment; verification of differences in the IGRT experience of RTTs for the different protocols for soft-tissue matching
Figure 3a shows the difference in 3D observer variations according to the amount of IGRT experience of the RTTs. Trainees with a median of just 3.5 months of IGRT experience showed a larger degree of observer variation than coaches in both GTV and PTV matching protocols; in particular, a significant difference was observed in PTV matching (P < 0.05). However, no significant difference in the observer variation was observed between coaches and trainees with a median of over 6 months of IGRT experience in either matching protocol. Figure 3b shows a case of PTV matching with the largest observer variation. The image quality around the PTV was degraded by artifacts from the biliary stent and intestinal gas. Although the time to complete soft-tissue matching decreased by an average of 80 s with an increase of 3 months of IGRT experience among trainees, even trainees with 6 months of IGRT experience spent more time on soft-tissue matching than coaches in both matching protocols (P < 0.05), as shown in Fig. 3c.
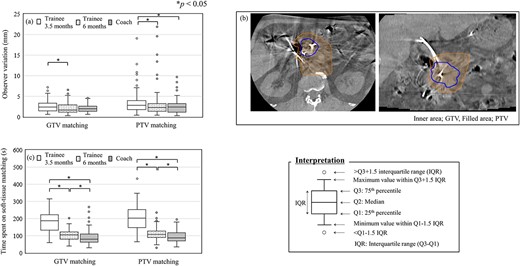
Observer variations in the IGRT experience of 11 RTTs (5 coaches and 6 trainees) for the soft-tissue matching. ‘Trainee 3.5 months’ indicates the population of trainees who had a median of 3.5 months of IGRT experience. ‘Trainee 6 months’ indicates the population of the same trainees with an additional 3 months of IGRT experience. 3D observer variations (a). CBCT images of a PTV matching case with the largest interobserver variation (b). The inner area bounded by line shows the GTV, and the filled area shows the PTV. Time spent on soft-tissue matching (c).
Inter- and intrafraction positional variations
Figure 4 shows the distribution of the target localization error in 810 of the first CBCT scans (15 fractions for 54 patients) and the cumulative frequency for each direction. The percentage of target localization error exceeding 5 mm between pCT and the first CBCT scan after bone matching with the ExacTrac system in the AP, SI and LR directions was 2.7, 10.1 and 1.9%, respectively. The percentage of target localization error exceeding 5 mm in the PTV matching was 4.8%, even in the SI direction. By contrast, in the GTV matching, the percentage of target localization error exceeding 5 mm between pCT and the first CBCT scan was 12.6%.
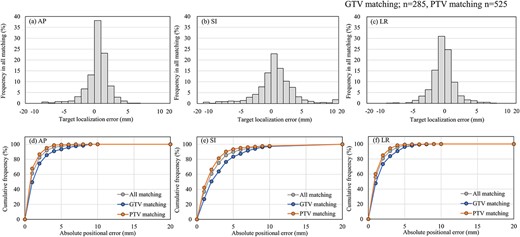
Target localization error on the first CBCT scan for all matching. Matching was performed using a total of 810 scans (GTV matching; n = 285, PTV matching; n = 525). Histograms for the AP (a), SI (b) and LR (c) directions. Cumulative frequency for the AP (d), SI (e) and LR (f) directions.
Figure 5 shows the distribution of the interfraction positional variation in the different soft-tissue matching protocols and the cumulative frequency for each direction. Among the 810 first-CBCT scans, 285 involved GTV matching and 525 involved PTV matching. The interfraction positional variation of PTV matching was smaller than that of GTV matching.
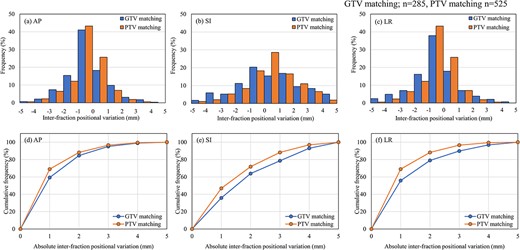
Interfraction positional variation for GTV matching (n = 285) and PTV matching (n = 525). Histograms of the AP (a), SI (b) and LR (c) directions. Cumulative frequency of the AP (d), SI (e) and LR (f) directions.
The histograms of the intrafraction positional variation in each direction are shown in Fig. 6a–c. Among the 54 patients, 26 underwent two or more CBCT scans. A total of 88 scans were conducted in those who received a CBCT scan a second time, including 51 involving GTV matching and 37 involving PTV matching. Two patients who received GTV matching required a third CBCT scan due to poor reproducibility of the BHs. Among these 26 patients, 20 (76.9%) underwent a second CBCT scan for a maximum of 3 treatment days during treatment. However, three patients underwent a second CBCT scan for a minimum of 10 treatment days during treatment, as shown in Fig. 6d. Tolerance was achieved, as noted, by the third CBCT scan on each treatment day in all patients.
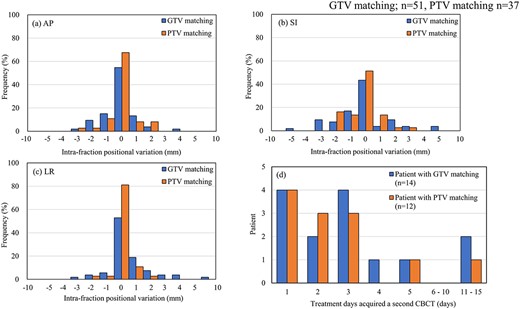
Intrafraction positional variation for GTV matching (n = 51) and PTV matching (n = 37). Histograms of the AP (a), SI (b) and LR (c) directions. Relationship between the number of patients and treatment days that required a second CBCT scan (d).
Table 2 shows the ∑tot, σtot and optimal margin size in each protocol for soft-tissue matching in each direction. The optimal margin size based on the van Herk formula was larger in the GTV matching than in the PTV matching. The increase in the optimal margin for GTV matching was largely affected by σtot.
Population systematic error (∑tot), random error (σtot) and optimal margin size in each direction
. | AP (mm) . | SI (mm) . | LR (mm) . | |
---|---|---|---|---|
GTV matching (PR patient) | ∑tot | 0.9 | 1.8 | 1.0 |
σtot | 1.7 | 2.8 | 2.2 | |
Margina | 3.7 | 5.4 | 3.9 | |
PTV matching (BR and UR patient) | ∑tot | 0.7 | 1.1 | 0.9 |
σtot | 1.4 | 1.9 | 1.2 | |
Margina | 2.7 | 4.1 | 3.0 | |
All matching (PR, BR and UR patient) | ∑tot | 0.8 | 1.2 | 0.9 |
σtot | 1.5 | 2.4 | 1.8 | |
Margina | 3.0 | 4.6 | 3.5 |
. | AP (mm) . | SI (mm) . | LR (mm) . | |
---|---|---|---|---|
GTV matching (PR patient) | ∑tot | 0.9 | 1.8 | 1.0 |
σtot | 1.7 | 2.8 | 2.2 | |
Margina | 3.7 | 5.4 | 3.9 | |
PTV matching (BR and UR patient) | ∑tot | 0.7 | 1.1 | 0.9 |
σtot | 1.4 | 1.9 | 1.2 | |
Margina | 2.7 | 4.1 | 3.0 | |
All matching (PR, BR and UR patient) | ∑tot | 0.8 | 1.2 | 0.9 |
σtot | 1.5 | 2.4 | 1.8 | |
Margina | 3.0 | 4.6 | 3.5 |
aThe bold values were the calculated margins using the van Herk formula, as indicated by 2.5 ∑ + 0.7 σ.
Population systematic error (∑tot), random error (σtot) and optimal margin size in each direction
. | AP (mm) . | SI (mm) . | LR (mm) . | |
---|---|---|---|---|
GTV matching (PR patient) | ∑tot | 0.9 | 1.8 | 1.0 |
σtot | 1.7 | 2.8 | 2.2 | |
Margina | 3.7 | 5.4 | 3.9 | |
PTV matching (BR and UR patient) | ∑tot | 0.7 | 1.1 | 0.9 |
σtot | 1.4 | 1.9 | 1.2 | |
Margina | 2.7 | 4.1 | 3.0 | |
All matching (PR, BR and UR patient) | ∑tot | 0.8 | 1.2 | 0.9 |
σtot | 1.5 | 2.4 | 1.8 | |
Margina | 3.0 | 4.6 | 3.5 |
. | AP (mm) . | SI (mm) . | LR (mm) . | |
---|---|---|---|---|
GTV matching (PR patient) | ∑tot | 0.9 | 1.8 | 1.0 |
σtot | 1.7 | 2.8 | 2.2 | |
Margina | 3.7 | 5.4 | 3.9 | |
PTV matching (BR and UR patient) | ∑tot | 0.7 | 1.1 | 0.9 |
σtot | 1.4 | 1.9 | 1.2 | |
Margina | 2.7 | 4.1 | 3.0 | |
All matching (PR, BR and UR patient) | ∑tot | 0.8 | 1.2 | 0.9 |
σtot | 1.5 | 2.4 | 1.8 | |
Margina | 3.0 | 4.6 | 3.5 |
aThe bold values were the calculated margins using the van Herk formula, as indicated by 2.5 ∑ + 0.7 σ.
DISCUSSION
In this study, we focused on revealing the target localization accuracy of CBCT under the EE-BH condition for two different soft-tissue matching protocols according to the resectability classification in patients with pancreatic cancer.
We initially hypothesized that soft-tissue matching that focused on the local area containing the GTV would be more accurate than that focused on the outline of the PTV. However, PTV matching was less dependent on interfraction positional variation than GTV matching (Fig. 5). This low accuracy of GTV could be explained by the following two possibilities: (i) the pancreas could have been deformed in some cases, and so could the area around the GTV, depending on daily changes in the shape and position of tissues surrounding the pancreas, especially the stomach and intestine; and (ii) the presence of artifacts on CBCT images. Although the iterative reconstruction algorithm improves image quality when compared with the traditional CBCT using Feldkamp-based reconstruction [23], artifacts such as streak artifacts due to intestinal peristalsis and metal artifacts due to the presence of a biliary stent still persist. By contrast, PTV matching exhibited a small intrafraction positional variation because some structures within the PTV enabled soft-tissue matching, even though the area around the GTV was blurred by artifacts. Figure 3b shows an example of CBCT images with severe artifacts. The structures within the GTV were relatively obscured by the artifacts compared with those within the PTV. Similar to the interfractional position variation, the intrafraction positional variation of the PTV matching was smaller than that of the GTV matching (Fig. 6). Consequently, the GTV matching may have been overcorrected due to artifacts on the CBCT images. Therefore, the use of fiducial markers should be considered even in conventionally fractionated RT, if accurate matching in the local area is required.
In the preliminary experiment, the observer variation among trainees with a median of only 3.5 months of IGRT experience was larger than that among coaches, especially for the PTV matching. However, no significant difference in observer variation was found by gaining an additional 3 months of IGRT experience (6 months total). Moreover, the RTTs with limited IGRT experience spent significant time on soft-tissue matching, which could result in a drift in the baseline owing to muscle relaxation and patient motion [13, 24]. With experience, the time required for soft-tissue matching becomes shortened. To shorten the time required to complete soft-tissue matching, a rule that the verification of the image matching needs to be agreed upon by two RTTs has been implemented at our institution. However, it should be noted that the observer variation was included in soft-tissue matching, even though RTTs with enough IGRT experience are like coaches.
The soft-tissue matching should be taken into account for the uncertainties, such as a delineation error, an observer variation and reproducibility of BH. For this reason, in our institution, the tolerance of the target localization error, regardless of the matching protocol, was set at ±5 mm in any direction, which was derived from PTV margins of 5 mm. According to this tolerance, the margin size calculated from the last CBCT scan for all patients was 4.6 mm in the SI direction, which turned out to be smaller than the planned PTV margin size. However, to improve the accuracy of target localization, a tighter tolerance should be set or all first CBCT scans should be corrected, and a second CBCT scan should be acquired for verification. In fact, 89.0% of all treatment fractions were within the tolerance after bone matching. In a previous study, the target matching for 11 patients with pancreatic cancer using EE-BH CBCT with visual feedback indicated an interfraction positional variation >5 mm in the bone matching position in 11.0% of all fractions [16]. Although visual feedback can aid in respiratory motion management [25–27], based on our clinical experience, patients occasionally complained about discomfort while using goggle-type visual feedback. Additionally, a limitation of this method is patient confusion regarding whether they can hold their breath at the determined position. As shown in Fig. 3, this study revealed that the target localization error after bone matching without visual feedback was comparable with that obtained with visual feedback. Therefore, target localization accuracy under the EE-BH condition could be ensured even without visual feedback. Effective respiratory motion management can be achieved by ensuring patients have a sufficient understanding of the BH procedure and by establishing a consensus on the BH policy among RTTs.
This study has two limitations. First, no patients with fiducial markers were enrolled in this study. Thus, the gold standard in soft-tissue matching could not be defined. However, all soft-tissue matching results were post-checked and approved by board-certified radiation oncologists. Thus, the localization accuracy obtained from soft-tissue matching using CBCT in a large sample of patients with pancreatic cancer would be reliable. Second, the intrafractional positional variation was often defined as the difference in the target position on CBCT scans obtained pre- and post-treatment to evaluate target motion during treatment [8]. By contrast, we defined it as the difference in the target localization between the (i + 1)th and ith CBCT scan in only 26 patients with a target localization error in any direction exceeding 5 mm on the first CBCT scan. Although the acquisition interval between two CBCTs in our definition was shorter than that in other literature, the intrafractional positional variation calculated from the second or more CBCT scan was comparable to the results published by Hill et al. [8]. Additionally, the motion of the target was quite small during BH, according to Sasaki et al. [18]. By contrast, BH reproducibility was the poorest in the SI direction, and it was reported that the mean ± SD was 1.9 ± 1.0 mm for inhalation and − 0.1 ± 2.2 mm for exhalation, respectively [18, 28]. Considering these facts, the intrafractional target position with the BH technique may be most affected by the reproducibility of BHs in the case of the short treatment time with the single arc VMAT. Therefore, our intrafractional positional variation should be comparable to the standard intrafractional positional variation obtained from pre- and post-treatment.
CONCLUSION
This study demonstrated the target localization accuracy for soft-tissue matching using CBCT in a large sample of patients with pancreatic cancer. Contrary to our expectations, GTV matching showed larger variations in target localization than PTV matching. An optimal margin size for PTV matching was within 5.0 mm in all directions, which was smaller than that of GTV matching. By determining the tolerance level of the target localization error, treatment can be successful within the planned margin size. However, matching should be performed cautiously when a high target localization accuracy in a localized area is required, as in GTV matching.
ACKNOWLEDGEMENTS
We sincerely appreciate all radiation therapists in the Division of Clinical Radiology Service, Kyoto University Hospital for their participation in the preliminary experiment on this study. We would also like to thank Editage (www.editage.com) for English language editing.
CONFLICT OF INTEREST
The authors declare that they have no competing interests.
FUNDING
This research was supported by the Japan Society for the Promotion of Science (JSPS) KAKENHI grant number 20K16757 and 22H03021. These sponsors had no involvement in the study design or the collection, analysis and interpretation of data.
ETHICAL CONSIDERATIONS
The study was conducted in accordance with the Declaration of Helsinki and approved by the Institutional Ethical Review Board (approval number R1446).
CONSENT FOR PUBLICATION
Consent was provided via our institutional consent form.
DATA AVAILABILITY
The data that support the findings of this study are available from the corresponding author upon reasonable request.