-
PDF
- Split View
-
Views
-
Cite
Cite
Hajime Ishinaga, Yoshinaga Okugawa, Bo Hou, Feng He, Chengzeng Yin, Mariko Murata, Yuji Toiyama, Kazuhiko Takeuchi, The role of miR-21 as a predictive biomarker and a potential target to improve the effects of chemoradiotherapy against head and neck squamous cell carcinoma, Journal of Radiation Research, Volume 64, Issue 4, July 2023, Pages 668–676, https://doi.org/10.1093/jrr/rrad043
- Share Icon Share
Abstract
This study aimed to clarify whether circulating miR-21 represents a predictive biomarker in patients with head and neck squamous cell carcinoma (HNSCC) undergoing chemoradiotherapy, and to investigate the effect of miR-21 inhibitor for chemoradiation in human SCC cells. Plasma samples were obtained from 22 patients with HNSCC and 25 non-cancer volunteers. Plasma miR-21 expression was measured using real-time quantitative reverse transcription polymerase chain reaction. The effects of miR-21 inhibitor in human SCC cells were investigated by performing 3-(4,5-dimethylthiazol-2-yl)-2,5-diphenyltetrazolium bromide assay, flow cytometry and western blot analysis. As a result, plasma miR-21 expression was higher in HNSCC patients than in control patients (P < 0.001). Seven patients with recurrence showed significantly higher plasma miR-21 than the 15 patients without recurrence. And high miR-21 expression group showed poor overall survival. Moreover, miR-21 inhibition significantly enhanced cisplatin- or radiation-induced apoptosis. Western blot analysis suggested the programmed cell death 4 protein as a potential target of miR-21 in relation to apoptosis. In conclusion, this study provides new insights into the role of miR-21 as a predictive biomarker for HNSCC treated with chemoradiotherapy and suggests a potential target to improve the effects of chemoradiotherapy against HNSCC.
BACKGROUND
Head and neck squamous cell carcinoma (HNSCC) is the seventh most common cancer in the world, with an annual incidence of >19.3 million new cases and around 10.0 million cancer deaths each year [1, 2]. Concurrent chemoradiotherapy is the standard treatment for locally advanced (stages III–IV) HNSCC [3], and cisplatin is most widely used in combination with radiotherapy for this pathology [3]. According to the Longitudinal Oncology Registry of Head and Neck Carcinoma report for the years 2005–2010, 70% of patients with HNSCC in the USA received combination therapy with a cisplatin-based regimen and radiotherapy [4]. Nevertheless, 5-year overall survival (OS) rates for locally advanced HNSCC remain unacceptable, at under 40% [5]. Poor outcomes are associated with chemoradioresistance, which can lead to local, locoregional or distant failure, and are rarely amenable to further treatment. Investigation of the molecular mechanisms underlying treatment failure and identification of predictive biomarkers are thus urgently required.
MicroRNAs (miRNAs) are small endogenous, non-coding RNAs of 18–25 nucleotides, acting as post-transcriptional gene expression regulators by destabilizing or inhibiting translation of mRNAs [6]. These miRNAs not only regulate gene expression, but also involve in carcinogenesis, such as cell proliferation, apoptosis and angiogenesis [7]. One of the most well-known miRNAs is miR-21, an oncomiR (i.e. a miRNA associated with cancer) associated with carcinomas such as HNSCC, esophageal squamous cell carcinoma (SCC), gastric carcinoma, lung carcinoma, colorectal carcinoma, breast cancer and pancreatic carcinoma [8–13]. Increased expression of miR-21 has been deeply involved with poor prognosis of various cancers due to its oncogenic roles in processes such as cell proliferation, migration and invasion [9]. The potential utility of miR-21 as a predictive biomarker for oncological treatment was recently elucidated, with Atrantes et al. [14] revealing that miR-21 obtained from tissue samples was able to predict the response to an organ-preservation protocol based on chemoradiation in HNSCC. However, whether miR-21 circulating in blood can predict chemoradioresistance in patients with HNSCC remains unclear, despite its potential as a strong and useful biomarker in clinical situations.
In addition, emerging evidence has demonstrated that miR-21 modulates the chemosensitivity of cancer cells primarily by targeting phosphatase and tensin homolog (PTEN) or programmed cell death 4 (PDCD4) [14–17]. With regard to the chemoresistance induced by miR-21 in HNSCC, a few reports have shown that miR-21 induced chemoresistance in oral SCC cells [18], but not in pharyngeal or laryngeal cell carcinomas, even though chemoradiotherapy is the standard therapy for advanced oropharyngeal, hypopharyngeal and laryngeal carcinomas. Whether radioresistance is induced by miR-21 in HNSCC remains unclear.
Our aim in this study was to clarify whether circulating miR-21 offers a possible predictive biomarker for patients with locally advanced HNSCC undergoing platinum-based chemoradiotherapy, and to investigate the effects of miR-21 inhibitor together with chemoradiotherapy in human HNSCC cells.
METHODS
Patient recruitment and sample collection
A total of 22 patients with advanced HNSCC (21 men, 1 woman; mean age, 60.7 years) and 25 volunteers with no cancer (20 men, 5 women; mean age, 62.3 years) were investigated in this study (Table 1). Control patients had no history of cancers in the preceding 3 years. Peripheral blood samples were collected from patients with HNSCC who were receiving platinum-based chemoradiotherapy in the Department of Otorhinolaryngology – Head & Neck Surgery in our hospital, from January 2015 until December 2016. Blood samples were collected before initiating chemoradiotherapy. Within the patient group, we also compared plasma levels of miR-21 between the 15 patients with no recurrence and the 7 patients with recurrence. Clinical characteristics of the 22 patients are listed in Table 2. Written informed consent was obtained from each patient before enrolment in this study.
. | . | . | Control . | . | . | HNSCC . |
---|---|---|---|---|---|---|
Patient’s number | 25 | 22 | ||||
Age (average) | 62.3 | 60.7 | ||||
Sex | ||||||
Male | 20 | 21 | ||||
Female | 5 | 1 | ||||
Serum miR-21 (average) | 0.95 | 1.41 | ||||
Tumor origin | ||||||
Hypopharynx | 10 | |||||
Oropharynx | 8 | |||||
Epipharynx | 2 | |||||
Maxillary sinus | 2 | |||||
T stage | ||||||
T1–2 | 11 | |||||
T3–4 | 11 | |||||
N stage | ||||||
N0–1 | 8 | |||||
N2–3 | 14 | |||||
Stage | ||||||
III | 8 | |||||
IV | 14 | |||||
Radiation dose | ||||||
70.2Gy | 19 | |||||
<70.2Gy | 3 | |||||
Regimen | ||||||
CDDP | 1 | |||||
CDDP+5-FU | 17 | |||||
CBDCA+5-FU | 4 |
. | . | . | Control . | . | . | HNSCC . |
---|---|---|---|---|---|---|
Patient’s number | 25 | 22 | ||||
Age (average) | 62.3 | 60.7 | ||||
Sex | ||||||
Male | 20 | 21 | ||||
Female | 5 | 1 | ||||
Serum miR-21 (average) | 0.95 | 1.41 | ||||
Tumor origin | ||||||
Hypopharynx | 10 | |||||
Oropharynx | 8 | |||||
Epipharynx | 2 | |||||
Maxillary sinus | 2 | |||||
T stage | ||||||
T1–2 | 11 | |||||
T3–4 | 11 | |||||
N stage | ||||||
N0–1 | 8 | |||||
N2–3 | 14 | |||||
Stage | ||||||
III | 8 | |||||
IV | 14 | |||||
Radiation dose | ||||||
70.2Gy | 19 | |||||
<70.2Gy | 3 | |||||
Regimen | ||||||
CDDP | 1 | |||||
CDDP+5-FU | 17 | |||||
CBDCA+5-FU | 4 |
. | . | . | Control . | . | . | HNSCC . |
---|---|---|---|---|---|---|
Patient’s number | 25 | 22 | ||||
Age (average) | 62.3 | 60.7 | ||||
Sex | ||||||
Male | 20 | 21 | ||||
Female | 5 | 1 | ||||
Serum miR-21 (average) | 0.95 | 1.41 | ||||
Tumor origin | ||||||
Hypopharynx | 10 | |||||
Oropharynx | 8 | |||||
Epipharynx | 2 | |||||
Maxillary sinus | 2 | |||||
T stage | ||||||
T1–2 | 11 | |||||
T3–4 | 11 | |||||
N stage | ||||||
N0–1 | 8 | |||||
N2–3 | 14 | |||||
Stage | ||||||
III | 8 | |||||
IV | 14 | |||||
Radiation dose | ||||||
70.2Gy | 19 | |||||
<70.2Gy | 3 | |||||
Regimen | ||||||
CDDP | 1 | |||||
CDDP+5-FU | 17 | |||||
CBDCA+5-FU | 4 |
. | . | . | Control . | . | . | HNSCC . |
---|---|---|---|---|---|---|
Patient’s number | 25 | 22 | ||||
Age (average) | 62.3 | 60.7 | ||||
Sex | ||||||
Male | 20 | 21 | ||||
Female | 5 | 1 | ||||
Serum miR-21 (average) | 0.95 | 1.41 | ||||
Tumor origin | ||||||
Hypopharynx | 10 | |||||
Oropharynx | 8 | |||||
Epipharynx | 2 | |||||
Maxillary sinus | 2 | |||||
T stage | ||||||
T1–2 | 11 | |||||
T3–4 | 11 | |||||
N stage | ||||||
N0–1 | 8 | |||||
N2–3 | 14 | |||||
Stage | ||||||
III | 8 | |||||
IV | 14 | |||||
Radiation dose | ||||||
70.2Gy | 19 | |||||
<70.2Gy | 3 | |||||
Regimen | ||||||
CDDP | 1 | |||||
CDDP+5-FU | 17 | |||||
CBDCA+5-FU | 4 |
. | . | . | Relapse free . | Recurrent . | . | P value . | |
---|---|---|---|---|---|---|---|
. | . | . | n = 15 . | . | n = 7 . | . | . |
Mean age | 61.7 | 58.7 | 0.832 | ||||
Sex | |||||||
Male | 14 | 7 | |||||
Female | 1 | 0 | 0.259 | ||||
Plasma miR-21 | |||||||
(Mean) | 1.27 | 1.7 | 0.04 | ||||
Stage | |||||||
III | 6 | 2 | |||||
IV | 9 | 5 | 0.49 | ||||
Subsite | |||||||
Oro-hypopharynx | 13 | 5 | |||||
Others | 2 | 2 | 0.378 | ||||
HPV status positive | 3 | 1 | |||||
Negative | 12 | 5 | 0.105 | ||||
Brinkman index | |||||||
≦400 | 4 | 1 | |||||
>400 | 11 | 5 | 0.651 | ||||
Sake index (mean) | 47.1 | 73.5 | 0.805 | ||||
CRP (mean) | 0.413 | 2.059 | 0.49 | ||||
NLR (mean) | 2.75 | 2.49 | 0.49 | ||||
SCC antigen (mean) | 1.66 | 2.33 | 0.112 |
. | . | . | Relapse free . | Recurrent . | . | P value . | |
---|---|---|---|---|---|---|---|
. | . | . | n = 15 . | . | n = 7 . | . | . |
Mean age | 61.7 | 58.7 | 0.832 | ||||
Sex | |||||||
Male | 14 | 7 | |||||
Female | 1 | 0 | 0.259 | ||||
Plasma miR-21 | |||||||
(Mean) | 1.27 | 1.7 | 0.04 | ||||
Stage | |||||||
III | 6 | 2 | |||||
IV | 9 | 5 | 0.49 | ||||
Subsite | |||||||
Oro-hypopharynx | 13 | 5 | |||||
Others | 2 | 2 | 0.378 | ||||
HPV status positive | 3 | 1 | |||||
Negative | 12 | 5 | 0.105 | ||||
Brinkman index | |||||||
≦400 | 4 | 1 | |||||
>400 | 11 | 5 | 0.651 | ||||
Sake index (mean) | 47.1 | 73.5 | 0.805 | ||||
CRP (mean) | 0.413 | 2.059 | 0.49 | ||||
NLR (mean) | 2.75 | 2.49 | 0.49 | ||||
SCC antigen (mean) | 1.66 | 2.33 | 0.112 |
NLR: neutrophil–lymphocyte ration
. | . | . | Relapse free . | Recurrent . | . | P value . | |
---|---|---|---|---|---|---|---|
. | . | . | n = 15 . | . | n = 7 . | . | . |
Mean age | 61.7 | 58.7 | 0.832 | ||||
Sex | |||||||
Male | 14 | 7 | |||||
Female | 1 | 0 | 0.259 | ||||
Plasma miR-21 | |||||||
(Mean) | 1.27 | 1.7 | 0.04 | ||||
Stage | |||||||
III | 6 | 2 | |||||
IV | 9 | 5 | 0.49 | ||||
Subsite | |||||||
Oro-hypopharynx | 13 | 5 | |||||
Others | 2 | 2 | 0.378 | ||||
HPV status positive | 3 | 1 | |||||
Negative | 12 | 5 | 0.105 | ||||
Brinkman index | |||||||
≦400 | 4 | 1 | |||||
>400 | 11 | 5 | 0.651 | ||||
Sake index (mean) | 47.1 | 73.5 | 0.805 | ||||
CRP (mean) | 0.413 | 2.059 | 0.49 | ||||
NLR (mean) | 2.75 | 2.49 | 0.49 | ||||
SCC antigen (mean) | 1.66 | 2.33 | 0.112 |
. | . | . | Relapse free . | Recurrent . | . | P value . | |
---|---|---|---|---|---|---|---|
. | . | . | n = 15 . | . | n = 7 . | . | . |
Mean age | 61.7 | 58.7 | 0.832 | ||||
Sex | |||||||
Male | 14 | 7 | |||||
Female | 1 | 0 | 0.259 | ||||
Plasma miR-21 | |||||||
(Mean) | 1.27 | 1.7 | 0.04 | ||||
Stage | |||||||
III | 6 | 2 | |||||
IV | 9 | 5 | 0.49 | ||||
Subsite | |||||||
Oro-hypopharynx | 13 | 5 | |||||
Others | 2 | 2 | 0.378 | ||||
HPV status positive | 3 | 1 | |||||
Negative | 12 | 5 | 0.105 | ||||
Brinkman index | |||||||
≦400 | 4 | 1 | |||||
>400 | 11 | 5 | 0.651 | ||||
Sake index (mean) | 47.1 | 73.5 | 0.805 | ||||
CRP (mean) | 0.413 | 2.059 | 0.49 | ||||
NLR (mean) | 2.75 | 2.49 | 0.49 | ||||
SCC antigen (mean) | 1.66 | 2.33 | 0.112 |
NLR: neutrophil–lymphocyte ration
Cell culture
HNSCC FaDu and SAS cell lines (derived from human hypopharyngeal cell carcinoma and human tongue SCC, respectively) were obtained from RIKEN BRC Cell Bank (Tsukuba, Japan). These HNSCC cell lines were maintained in modified Eagle’s medium (MEM) or RPMI-1640 medium with 10% fetal bovine serum (FBS) and antibiotics (1% penicillin, streptomycin), respectively. All human cell lines have been authenticated using short tandem repeat profiling in 2021.
RNA extraction from plasma
RNA was extracted from 200 μl of plasma by using an miRNeasy Serum/Plasma kit (QIAGEN, Hiden, Germany) in the automated QIAcube (QIAGEN) as described previously [10], according to the manufacturer’s instructions. A synthetic Caenorhabditis elegans miR-39 miRNA mimic (QIAGEN) and carrier RNA (0.94 μg, MS2 bacteriophage total RNA; Roche Applied Sciences, Indianapolis, IN, USA) were spiked-in before RNA extraction. Isolated RNA was eluted in 15 μl of RNase-free water.
Reverse transcription quantitative polymerase chain reaction for plasma miR-21
cDNA was synthesized from 5 μl of eluted RNA (containing miRNAs) in HiFlex buffer (QIAGEN), by using an miScript II RT kit (QIAGEN), as described previously [10]. Next, quantitative reverse transcription-polymerase chain reaction (RT-PCR) was performed using the miScript SYBR Green PCR kit (QIAGEN) and miScript Primer Assays. MiRNA were normalized against the average of four reference miRNAs (miR-423-5p, miR-103a-3p, miR-191-5p and miR-93), which were used as internal controls in plasma samples. In addition, syn-cel-miR-39 was used to confirm the extraction efficacy of RNA. All amplifications were carried out in an ABI Step One Plus Real-time PCR System (Applied Biosystems, Singapore, Singapore). Amplification curves were analyzed using SDS software version 2.2.2 (Applied Biosystems). Expression levels of mRNA were determined by using the 2−ΔΔC method.
Transfection experiments using miR-21 inhibitor
To transiently inhibit miR-21 expression, hsa-miR-21 mirVana™ miRNA inhibitor (Applied Biosystems) or hsa-miR-21 mirVana® mimics (Applied Biosystems) were used to transfect HNSCC cells, as described previously [19]. Verification of transfection efficiency was conducted using the mirVana™ miRNA Mimic Negative Control (Applied Biosystems) and mirVana™ miRNA Inhibitor Negative Control (Applied Biosystems), respectively. Forward transfections were conducted by mixing miRNA mimic or inhibitor (70 nM) with Lipofectamine RNAiMAX (Invitrogen, Carlsbad, CA, USA) and Opti-MEM I (Invitrogen) and applying the mixture to cells at 24 h after plating. A series of in vitro assays was conducted after 48–72 h of incubation.
Cell viability assay
A 3-(4,5-dimethylthiazol-2-yl)-2,5-diphenyltetrazolium bromide (MTT) assay was performed to assess the anti-proliferative effects of miR-21 inhibitor and proliferative effects of the miR-21 mimic on HNSCC cells, as previously described [20]. Cells were plated at a density of 4 × 103 cells/well into a 96-well plate, transfected with either miR-21 mimic or inhibitor, and treated with either 48 h administration of cisplatin at various concentrations (0, 0.2, 1 or 5 μg/ml) or three exposures to radiation at various doses (0, 2 or 4 Gy). The cells were then incubated for a total of 72 h. Next, 10 μl of 5 mg/ml MTT (Sigma-Aldrich, St Louis, MO, USA) in 100 μl of medium was added to each well, followed by incubation for 4 h at 37°C. The medium was removed and replaced with 100 μl DMSO, and absorbance values were measured at 570 nm on a Bio-Rad model 680 microplate reader (Bio-Rad Laboratories, Hercules, CA, USA).
Cell apoptosis assay
Quantitative analysis of apoptotic and dead cells was performed by using An Annexin V and Dead Cell Assay kit (Millipore, Burlington, MA, USA) with a flow cytometer (Muse™ Cell Analyzer; Millipore), as described previously [21], according to the instructions from the manufacturer. After incubating with cisplatin (0, 4 or 6 μg/ml) for 48 h or exposure to radiation (0, 4 or 12 Gy), all cells were harvested and diluted to a concentration of 5 × 105 cells/ml in MEM medium with 2% FBS. One hundred microliters of Annexin V and Dead Reagent and 100 μl of single cell suspension were then mixed in a microtube and analyzed by using the Muse™ Cell Analyzer (Millipore) after 30-min incubation in the dark at room temperature. All experiments were performed in quadruplicate.
Western blot analysis
After transfection with miR-21 mimic or inhibitor for 48 h, cells were harvested and lysed using RIPA buffer (Cell Signaling Technology, Dancers, MA, USA) supplemented with phenylmethylsulphonyl fluoride (Nacalai Tesque, Kyoto, Japan). Equal amounts of protein were run on sodium dodecyl sulfate polyacrylamide gel electrophoresis and transferred to polyvinylidene fluoride membranes (0.45 μm; Millipore). Membranes were washed with Tris-buffered saline (TBST) containing 0.1% Tween-20 (Nacalai Tesque) and 5% bovine serum albumin (Sigma-Aldrich, St Louis, MO, USA), and incubated overnight at 4°C with primary antibodies (PDCD4 antibody from Cell Signaling Technology, Danvers, MA, USA; β-actin antibody from MP Biomedicals, LLC, Irvine, CA, USA). After washing with TBST, membranes were further incubated with horseradish peroxidase-conjugated secondary antibody (1:10 000; Santa Cruz Biotechnology, Dallas, TX, USA) for 1 h at room temperature and developed by using an electrochemiluminescence system in the end (GE Healthcare, Little Chalfont, UK). Protein bands were detected by an LAS4000mini imaging system (Fujifilm, Tokyo, Japan), and band intensities of western blots were quantitatively measured by calculating integrated grayscale densities in consistently sized windows incorporating each band using ImageJ version 1.48 software, as described previously [22] .
Statistical analysis
All statistical analyses were performed with IBM SPSS version 23 statistical software (IBM, Chicago, IL, USA). Two-group comparisons of ΔCt values between plasma samples from controls and HNSCC patients, as well as relapse-free and recurrent patients, were performed by using the Mann–Whitney U-test. The Kaplan–Meier method with log-rank comparison was selected to calculate OS, measured from the date of chemoradiotherapy initiation until the date of death from any cause (i.e. cancer-unrelated deaths were not censored) or the last known follow-up for patients who were still alive. Statistical differences between groups were calculated by χ2 text, unpaired t-test, Mann–Whitney test and one-way ANOVA with post hoc multiple comparisons. Values of P < 0.05 were considered to be a statistical significance.
RESULTS
Plasma miR-21 level and OS rate in HNSCC patients treated with chemoradiotherapy
First, peripheral blood samples were collected from 22 patients with advanced HNSCC treated using platinum-based chemoradiotherapy and 25 healthy subjects without HNSCC, and levels of plasma miR-21 expression were detected by quantitative PCR (qPCR). As expected, relative levels of miR-21 were significantly higher in HNSCC patients than in control subjects (Fig. 1A; P < 0.001). Next, we sought to determine whether expression levels of plasma miR-21 were associated with tumor recurrence after chemoradiation. Our results demonstrated a significant difference in plasma miR-21 levels between the tumor-free and recurrent groups (Fig. 1B; P < 0.05). Factors including age, sex, stage, subsite, HPV status, Brinkman index, Sake index, CRP, neutrophil–lymphocyte ratio and SCC antigen were compared between the tumor-free and recurrent groups (Table 2). In addition, we assessed the correlation between plasma miR-21 expression and OS. The results demonstrated a significant difference of OS between high miR-21 group and low miR-21 group, as shown in Fig. 1C (P < 0.05).
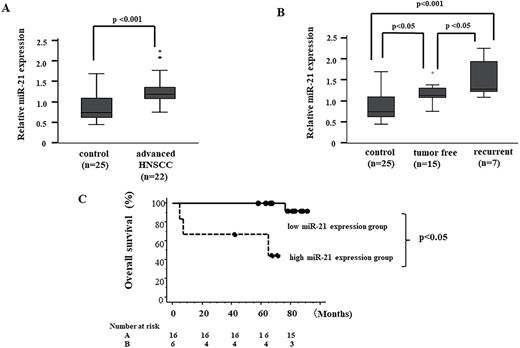
Plasma miR-21 levels in HNSCC patients. (A) Levels of miR-21 in plasma samples are measured using RT-qPCR methods. Expression level of miR-21 is calibrated to obtain fold change. (B) Levels of miR-21 in plasma samples are measured by RT-qPCR methods. Significant differences are determined using one-way ANOVA with post hoc correction. (C) OS according to plasma miR-21 expression level by Kaplan–Meier methods. A significant difference is seen between high miR-21 expression groups and low by log-rank testing. Patients >1.8 (mean miR-21 in all patients in this study) in miR-21 expression level were classified as high miR-21 expression group.
Increased sensitivity to radiotherapy in HNSCC cells by miR-21 inhibitor
Next, we hypothesized that miR-21 would play an important role in chemoradioresistance to platinum-based chemoradiotherapy for HNSCC. First, we confirmed expression levels of miR-21 by RT-qPCR analysis in human HNSCC cells (FaDu cells derived from hypopharyngeal SCC) with transfection of miR-21 inhibitor. As expected, we could confirm that miR-21 inhibitor suppressed miR-21 expression (Fig. 2A). Cell proliferation was significantly suppressed by the miR-21 inhibitor compared with radiation alone (Fig. 2B and C). Similar results were also observed in the SAS cell line, a human tongue SCC cells (Fig. 2F).
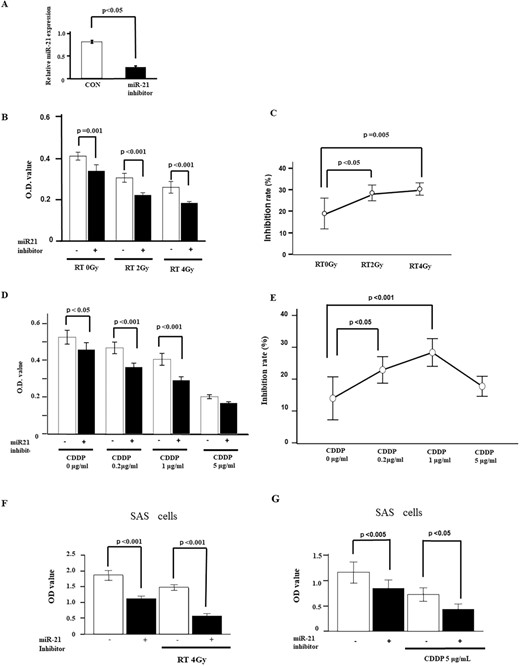
Effects of miR-21 inhibitor on radiosensitivity and chemosensitivity. (A) MiR-21 inhibitor in FaDu cells showed that ~70% knockdown of miR-21 expression is obtained by 48 h after transfection of 70 nM of miR-21 inhibitor (n = 3). (B) Antiproliferative effect of radiation with miR-21 inhibitor on MTT assay. FaDu cells transfected with miR-21 inhibitor are treated with various dose of radiation (0, 2 or 4 Gy) for three times (n = 6). (C) Inhibition rate for radiation with miR-21 inhibitor is assessed by MTT assay. Inhibition rate is enhanced by 2-Gy radiation with miR-21 inhibitor. (D) Antiproliferative effect of cisplatin with miR-21 inhibitor on MTT assay. FaDu cells transfected with miR-21 inhibitor are treated with various concentration of cisplatin (0, 0.2, 1 or 5 μg/ml) for 48 h (n = 6). (E) Inhibition rate for cisplatin with miR-21 inhibitor is assessed by MTT assay. Inhibition rate is enhanced by 1 μg/ml of cisplatin with miR-21 inhibitor. (F, G) Effects of miR-21 inhibitor are also confirmed in SAS cells. Antiproliferative effects from co-treatment with radiation and miR-21 inhibitor are demonstrated in the left panel. Effects from co-treatment with cisplatin and miR-21 inhibitor are shown in the right panel. Values are presented as mean ± standard error. Data are representative of three independent experiments.
Increased sensitivity to chemotherapy in HNSCC cells by miR-21 inhibitor
We also investigated whether miR-21 is involved in the chemosensitivity of HNSCC treated with miR-21 inhibitor using the MTT assay. We selected CDDP concentration at 0.2, 1 and 5 μg/ml for MTT assay. Cell proliferation was significantly decreased by cisplatin treatment in a concentration-dependent manner, and miR-21 inhibitor enhanced the effects of cisplatin treatment in a similar manner to that with radiation (Fig. 2D and E). Similar results were also observed in SAS cells (Fig. 2G).
Apoptotic effects of miR-21 inhibitor in HNSCC cells
We further analyzed the effects of miR-21 inhibitor on apoptosis from treatment with either cisplatin or radiation by flow cytometry after annexin V and 7-amino-actinomycin D staining. The results demonstrated that FaDu cells transfected with miR-21 inhibitor showed synergistic enhancement of apoptosis compared with either cisplatin alone or radiation alone (Fig. 3A–D).
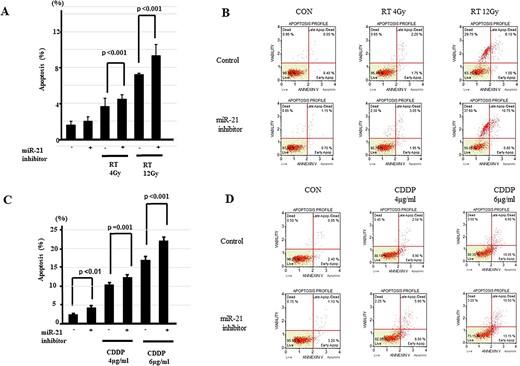
Apoptotic effects of miR-21 inhibitor in HNSCC. (A) Apoptosis assay is performed to measure the population of apoptotic FaDu cells after exposure to radiation with miR-21 inhibitor. Rate of apoptotic cells is significantly enhanced by miR-21 knockdown with radiation (n = 6/group) as shown in the left panel. (B) Representative graphs of flow cytometry for miR-21 knockdown with radiation. Upper left: no treatment; middle left: 4-Gy radiation alone; lower left: 12-Gy radiation alone; upper right: miR-21 inhibitor alone; middle right: miR-21 inhibitor +4-Gy radiation; lower right: miR-21 inhibitor +12-Gy radiation. (C) Apoptotic effects of cisplatin are enhanced by co-treatment with miR-21 inhibitor. Rate of apoptotic cells is significantly enhanced by miR-21 knockdown with cisplatin (n = 6/group). (D) Representative graphs of flow cytometry for miR-21 knockdown with cisplatin. Values are presented as mean ± standard error. Data are representative of three independent experiments.
PDCD4 as a possible target of miR-21
PDCD4 has been reported as a target molecule related to drug sensitivity in various cancers [23, 24]. Therefore, we investigated the protein levels of PDCD4 following transfection of the miR-21 inhibitor or mimic in FaDu cells using western blot analysis (Fig. 4A–D). In addition, we provided Supplementary Figs. 1 and 2 as full-length data for the western blot analysis, as shown in Figs. 4A and C. As expected, we could confirm that miR-21 inhibitor upregulated PDCD expression, while miR-21 mimic decreased PDCD4 expression.
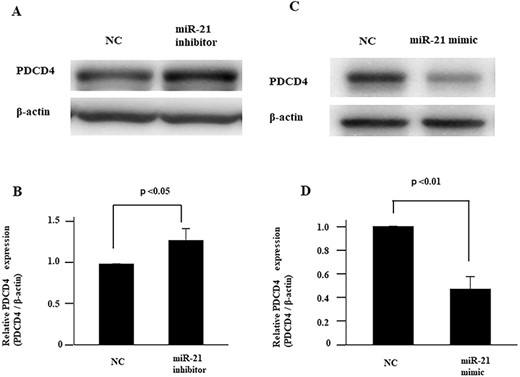
Effects of miR-21 on expression of PDCD4. Administration of miR-21 inhibitor increases the expression of PDCD4 in FaDu cells, as assessed by western blot analysis. Full length western blot data of Fig. 5A are provided as Supplementary Data 1 (Suppl. 1). (B) PDCD4 protein expression level after transfection of miR-21 inhibitor is standardized against the level of GAPDH and presented as the relative intensity. (C) Overexpression of miR-21 suppresses expression of PDCD4 in FaDu cells, as assessed by western blot analysis. Full length western blot data of Fig. 5C are provided as Supplementary Data 2 (Suppl. 2). (D) PDCD4 protein expression level with overexpression of miR-21 is standardized against the level of GAPDH and presented as the relative intensity. Values are presented as mean ± standard error. Data are representative of three independent experiments. Data are representative of three independent experiments (n = 3).
DISCUSSION
To improve the prognosis of HNSCC, it is crucial to identify useful biomarkers for estimating chemoradiosensitivity in individual HNSCC patients. A new finding in this study was the direct evidence that the expression levels of plasma miR-21, not tissue samples, were significantly higher in HNSCC patients with recurrence compared with those without recurrence. As expected, the high miR-21 expression group showed poorer OS (Fig. 1C). Our results suggest that plasma miR-21 at the pretreatment time-point may serve as a potential predictor of HNSCC susceptibility to chemoradiotherapy. Several studies have described circulating miRNAs as predictors of the efficacy of chemoradiotherapy in various cancers, including rectal cancer [25, 26], cervical SCC [27], nasopharyngeal carcinoma [28], HNSCC [29] and esophageal carcinoma [30]. In addition, in the field of HNSCC, circulating miR-1290 [29] has been reported as a useful prognostic biomarker for oral SCC, and circulating miR-744 has been reported as useful for nasopharyngeal carcinoma [31]. However, no reports have identified circulating miR-21 as predictive of chemoradiosensitivity in HNSCC patients. Our study may thus bring new insights into the novel roles of plasma miR-21 in predicting chemoradioresistance for HNSCC patients.
In addition, further interesting evidence was provided for the involvement of miR-21 in suppressing chemoradiosensitivity in HNSCC in this study. Even though miR-21 is well-known as an oncomiR for various types of carcinoma [10–13], whether elevated miR-21 levels are associated with chemoradioresistance and the influence of miR-21 on radioresistance in HNSCC have remained unclear. The present study provides first-hand evidence that miR-21 inhibitor enhanced the antiproliferative effects of radiation in HNSCCs, including FaDu cells and SAS cells, which represent hypopharyngeal SCC and tongue SCC cell lines, respectively, except for the nasopharyngeal carcinoma cell line. [32]. In addition, miR-21 inhibitor was also found to enhance the apoptotic effects of radiation, but had little effect by miR-21 inhibitor alone. These data confirmed that miR-21 caused radioresistance by increasing cell proliferation and decreasing apoptosis, identifying a potential therapeutic target for reducing radioresistance. Additional experimental significance of our findings was that miR-21 inhibitor also enhanced the antiproliferative effects of cisplatin in HNSCCs, including FaDu and SAS cell lines. A few reports have described miR-21 as modulating chemosensitivity to cisplatin for oral SCC [18, 33], but no reports are available for oropharyngeal or hypopharyngeal cell carcinoma. Since chemoradiotherapy is the standard therapy for advanced oropharyngeal or hypopharyngeal cell carcinomas but not oral SCC, the present study offers useful information for clinical strategies. Furthermore, miR-21 inhibitor also enhanced the apoptotic effects of radiation, but had little effect when administered alone. Collectively, these data demonstrated that miR-21 was deeply involved in the chemoradioresistance of HNSCC, suggesting miR-21 as a potential target to maximize the effects of chemoradiotherapy on HNSCC.
Finally, our data in this study implicated the involvement of PDCD4 as a target of miR-21 (Fig. 4A–D). Knockdown of miR-21 clearly elevated PDCD4 expression on western blotting. Logically, decreased levels of PDCD4 by miR-21 would suppress tumor cell apoptosis, contributing to chemoradioresistance [34–36]. However, miR-21 did not influence PTEN in FaDu cells in this study (data not shown). In addition to PDCD4, a number of targets of miR-21 have been already reported, including phosphatase and tensin homolog deleted on chromosome 10 (PTEN), which is a tumor suppressor gene encoding a phosphatase that regulates cell cycle, Akt and p53 activity. In addition, reversion-induced cystine-rich protein and tissue inhibitor of metalloproteinases 3 have been identified as suppressors of malignancy and inhibitors of matrix metalloproteinases [37–39].
We acknowledge there are several limitations of our study. Though plasma samples were obtained from 22 patients with HNSCC and 25 non-cancer volunteers, the sample size of plasma smiR-21 from patients was still small and mainly oro-hypopharyngeal cancers. Second, tissue miR-21 was not measured in HNSCC patients. In addition, this study is insufficient to provide a full understanding of the mechanisms by which miR-21 induces chemoradioresistance in HNSCC. This question needs to be addressed in the future investigations.
CONCLUSION
In conclusion, the present study suggested the importance of circulating miR-21 as a predictive biomarker in chemoradiotherapy for HNSCC. Our data provide new insights into novel roles of miR-21 for treating HNSCC.
CONFLICT OF INTEREST
The authors have no competing interest to declare.
FUNDING
This work was partly supported by EKIDEN for Life 2016, Bristol-Myers Squibb Company 2014, and Merck Serono 2015. Part of this work was supported by JSPS KAKENHI grant number 22K09683 (to H.I.), JP16H05255 (to M.M.), and by funds from Mie University Hospital (2015).
DATA AVAILABILITY
The datasets generated and/or analyzed during the current study are available from the corresponding author on reasonable request.
AUTHOR CONTRIBUTIONS
H.I., Y.O., Y.T. and M.M. designed the experiments. B.H. and F.H. collected samples and measured plasma miR-21. H.I., C.Y. and Y.O. performed the research. H.I. and K.T. wrote the manuscript.
ETHICAL STATEMENT
This study was approved by the ethics committee at Mie University Graduate School of Medicine (approval nos. 2445 and H2020-232).