-
PDF
- Split View
-
Views
-
Cite
Cite
Alexander S Plattner, Christine R Lockowitz, Rebekah Dumm, Ritu Banerjee, Jason G Newland, Rebecca G Same, Practice Versus Potential: The Impact of the BioFire FilmArray Pneumonia Panel on Antibiotic Use in Children, Journal of the Pediatric Infectious Diseases Society, Volume 13, Issue 3, March 2024, Pages 196–202, https://doi.org/10.1093/jpids/piae014
- Share Icon Share
Abstract
The BioFire FilmArray Pneumonia Panel (BFPP), a multiplex PCR panel for the diagnosis of lower respiratory tract infections, has been proposed as a tool for antimicrobial stewardship. Few studies evaluate real-world implementation of the BFPP and no studies focus exclusively on children. Our institution implemented BFPP testing without restrictions.
We conducted a retrospective cohort study in children hospitalized at St. Louis Children’s Hospital to (1) characterize the use of the BFPP in pediatric patients and (2) assess how results impacted antibiotic use. We included all BFPP tests obtained during the first year after the introduction of the test, September 2021 through August 2022. The primary outcome was change in antibiotic therapy within 24 hours of results, which was compared to the potential change in antibiotic therapy determined by two infectious diseases clinicians.
One hundred sixty-nine tests from 126 patients were included. Nine patients were immunocompromised and 19 had chronic tracheostomy. The majority of tests were sent from tracheal aspirate specimens (92%) and from patients in an intensive care unit (94%). Only 51% of tests were obtained due to respiratory failure or suspected pneumonia. For 80% of test results, there was potential to change antibiotics, but change occurred in only 46% of tests in practice. Antibiotic escalation was more common (26%) than de-escalation (15%) or discontinuation (4.1%).
In a cohort of pediatric patients tested with the BFPP, the majority of tests were sent from tracheal aspirates and less than half of tests were associated with a change in antibiotics.
INTRODUCTION
Community-acquired pneumonia (CAP) is one of the leading causes of hospitalization among children in the United States, with an estimated annual incidence of 15.7 cases per 10 000 children, and leads to more antibiotic use than any other condition [1, 2]. Among hospitalized children with pneumonia, 21% require intensive care and 7% require mechanical ventilation. While a bacterial pathogen is identified in only 15% of cases, antibiotics are given in up to 88% of children hospitalized for CAP [1].
The BioFire FilmArray Pneumonia Panel (BFPP) received FDA clearance in November 2018 for diagnosis of lower respiratory tract infections (ie, pneumonia). The panel is a multiplex PCR performed on either bronchoalveolar lavage (BAL) or sputum (including tracheal aspirate) specimens and includes targets for 18 bacteria and 8 viruses that cause pneumonia, as well as 7 antibiotic resistance genes. Fifteen of the bacterial targets are reported in a semi-quantitative manner on a logarithmic scale between 104 and 107 copies/mL. Notably, the BFPP does not include targets to detect SARS-CoV-2 [3].
In preliminary studies, the BFPP results correlated well with standard of care (SOC) testing for both viruses and bacteria [4]. The BFPP is highly sensitive with semi-quantitative reporting values higher than corresponding reference cultures [5, 6]. The test can deliver results in as little as 1 h, compared to days needed for standard culture techniques. Due to the high concordance with SOC and rapid availability of results, the BFPP has been proposed as a tool for antimicrobial stewardship efforts. Retrospective studies suggest that 64–80% of test results could lead to a change in antibiotic therapy, with 48–70% of test results potentially leading to a discontinuation or de-escalation of antibiotic therapy [7–10]. However, early impact studies in adult intensive care unit (ICU) patients with COVID and concern for bacterial secondary infection have shown a potential for antibiotic overuse. In one study, antibiotics were started in 81% of patients with a positive BFPP, but were only withheld in 56% and stopped in 8% of patients with negative BFPPs [11].
To date, studies evaluating the real-world implementation of the BFPP in adults have yielded mixed results, and no studies have assessed the use of the BFPP in pediatric populations [8, 10–20]. We sought to characterize the use of the BFPP among hospitalized children and identify factors that influence the utility of testing in this population. Additionally, we assessed how BFPP results impact antibiotic use in practice, compared to the potential impact, for hospitalized children.
METHODS
Design, Setting, and Participants
This was a retrospective cohort study of children hospitalized at St. Louis Children’s Hospital, a 402-bed tertiary pediatric academic hospital in St. Louis, MO, during the first year following the introduction of the BFPP, from September 2021 through August 2022. The affiliated Barnes Jewish Hospital microbiology laboratory introduced the BFPP on September 1, 2021 with testing offered 24 h a day and 7 days a week. Per standard laboratory protocols for testing of respiratory specimens, a Gram stain is performed to ensure specimen quality. If >10 squamous epithelial cells are visualized per low power field, the specimen is rejected for PCR due to likely oral contamination. Whenever the BFPP test is ordered, a routine bacterial culture is performed in parallel to correlate with PCR results, identify off-panel bacterial pathogens, and perform susceptibility testing. Our institution implemented BFPP testing on lower respiratory tract specimens without restrictions or pre-approval. The antimicrobial stewardship program (ASP) developed a guidance document outlining the general approaches to using and interpreting the BFPP test for clinicians to reference. Additionally, during handshake stewardship rounds, the ASP team provided feedback and education intermittently, often after the test was ordered. There was no formal ASP audit and feedback of all BFPP results. All tests collected at St. Louis Children’s Hospital within the first year were eligible for inclusion in this study. Tests were excluded if the panel was not performed due to inadequate specimen quality or if patients died prior to results being reported, which would preclude antibiotic changes based on the results.
Data Collection
Individual tests were identified through a query within the electronic medical record, Epic. Manual retrospective chart review was then performed to collect demographic data, preexisting medical conditions and comorbidities (eg, presence of tracheostomy and immunocompromised status), antibiotics received, results of BFPP and concurrent respiratory cultures, and timing of testing relative to admission and other key clinical information (eg, intubation, ICU admission, infectious diseases [ID] consultation). Race and ethnicity data were recorded per US Census and institutional tracking methods. Information was stored within a secure REDCap database. The Washington University in St. Louis School of Medicine Institutional Review Board approved this study, with a waiver of informed consent.
Immunocompromised status was defined as receipt of chemotherapy, hematopoietic stem cell transplant, or solid organ transplant within the last 6 months, or current neutropenia with absolute neutrophil count less than 500 cells/mm3. ID consultation was defined by the presence of an ID note within 24 h prior to or following BFPP collection. Repeat testing was defined as multiple BFPP tests occurring within the same admission.
Exposures and Outcomes
Antibiotics were assessed at the time of BFPP PCR result and evaluated for change over the next 24 h. Start and stop times were determined based on order signature times. Inhaled antibiotics and antifungals were excluded. A “new intubation” was defined as intubation on the same calendar day or one day prior to BFPP results. Time from intubation to BFPP collection was calculated based on time from initial intubation and not from last ETT exchange. However, if a patient was extubated for more than 24 h prior to re-intubation, the date of new intubation was used.
The primary outcome was change in antibiotic therapy within 24 hours of BFPP results. These changes were characterized using the Antibiotic Spectrum Index (ASI), a metric that represents the range of antibiotic activity as a single number, with higher numbers representing “broader” coverage [21]. The total ASI of combined therapy at time of BFPP result was compared to the ASI following any changes to antibiotic therapy in the first 24 h, if any. Total ASI was calculated as the sum of the ASI of all antibiotics a patient was receiving at each time point. Antibiotic changes were classified as (1) “Stop” if ASI went from greater than zero to zero, (2) “De-escalation” if ASI decreased, (3) “Escalation” if ASI increased, or (4) “No change” if the ASI remained the same. To align with methods from previously published literature regarding the potential for changes in antibiotic regimen, we determined potential changes by independent review of pre- and post-BFPP antibiotic regimens along with PCR results with the assumption that patients were being treated for pneumonia [7–10]. Potential antibiotic changes were determined independently by two pediatric ID physicians (A. S. P. and R. G. S.) using local antibiogram data and institutional ASP empiric treatment guidelines, prioritizing the use of the narrowest effect agent that would treat organisms identified by PCR. For example, one patient was receiving ceftriaxone and vancomycin (ASI 10) at the time of a PCR result for Haemophilus influenzae and MSSA. The potential antibiotic change was “de-escalation” to ceftriaxone alone (ASI 5). Any discordance between the two parallel reviews was settled by a third pediatric ID physician (J. G. N.).
Statistical Analyses
Basic statistical analysis was performed using Microsoft Excel for Mac version 16.71. Welch’s T-test was used for comparison of test results from patients with chronic tracheostomy versus newly intubated patients, due to unequal sample size and variance between the two groups.
RESULTS
A total of 189 BFPP tests were sent during the 1-year study period. We excluded 19 tests because the panel was not performed due to poor specimen quality and 1 test that was obtained from a patient who died prior to results returning. We included 169 BFPP tests from 126 unique patients. Patient demographics and test characteristics are outlined in Tables 1 and 2. Seven percent of patients were immunocompromised and 15% had a chronic tracheostomy. The majority of tests were obtained from tracheal aspirate specimens (92%) and were sent from patients admitted to an ICU (94%).
Patient Demographics . | N (%) . |
---|---|
Sex | |
Female | 52 (41) |
Male | 73 (58) |
Other | 1 (0.8) |
Race | |
White | 85 (66) |
Black | 34 (27) |
Asian | 2 (1.6) |
American Indian or Alaska Native | 1 (0.8) |
Native Hawaiian or Pacific Islander | 1 (0.8) |
Unknown | 3 (2.4) |
Ethnicity | |
Hispanic | 8 (6.3) |
Non-Hispanic | 116 (92) |
Unknown | 2 (1.6) |
Immunocompromised | |
Yes | 9 (7.1) |
No | 117 (93) |
Chronic tracheostomy | |
Yes | 19 (15) |
No | 107 (85) |
Age (years) | |
Range | 0.0–24.5 |
IQR | 0.5–15.2 |
Median | 4.3 |
Patient Demographics . | N (%) . |
---|---|
Sex | |
Female | 52 (41) |
Male | 73 (58) |
Other | 1 (0.8) |
Race | |
White | 85 (66) |
Black | 34 (27) |
Asian | 2 (1.6) |
American Indian or Alaska Native | 1 (0.8) |
Native Hawaiian or Pacific Islander | 1 (0.8) |
Unknown | 3 (2.4) |
Ethnicity | |
Hispanic | 8 (6.3) |
Non-Hispanic | 116 (92) |
Unknown | 2 (1.6) |
Immunocompromised | |
Yes | 9 (7.1) |
No | 117 (93) |
Chronic tracheostomy | |
Yes | 19 (15) |
No | 107 (85) |
Age (years) | |
Range | 0.0–24.5 |
IQR | 0.5–15.2 |
Median | 4.3 |
Patient Demographics . | N (%) . |
---|---|
Sex | |
Female | 52 (41) |
Male | 73 (58) |
Other | 1 (0.8) |
Race | |
White | 85 (66) |
Black | 34 (27) |
Asian | 2 (1.6) |
American Indian or Alaska Native | 1 (0.8) |
Native Hawaiian or Pacific Islander | 1 (0.8) |
Unknown | 3 (2.4) |
Ethnicity | |
Hispanic | 8 (6.3) |
Non-Hispanic | 116 (92) |
Unknown | 2 (1.6) |
Immunocompromised | |
Yes | 9 (7.1) |
No | 117 (93) |
Chronic tracheostomy | |
Yes | 19 (15) |
No | 107 (85) |
Age (years) | |
Range | 0.0–24.5 |
IQR | 0.5–15.2 |
Median | 4.3 |
Patient Demographics . | N (%) . |
---|---|
Sex | |
Female | 52 (41) |
Male | 73 (58) |
Other | 1 (0.8) |
Race | |
White | 85 (66) |
Black | 34 (27) |
Asian | 2 (1.6) |
American Indian or Alaska Native | 1 (0.8) |
Native Hawaiian or Pacific Islander | 1 (0.8) |
Unknown | 3 (2.4) |
Ethnicity | |
Hispanic | 8 (6.3) |
Non-Hispanic | 116 (92) |
Unknown | 2 (1.6) |
Immunocompromised | |
Yes | 9 (7.1) |
No | 117 (93) |
Chronic tracheostomy | |
Yes | 19 (15) |
No | 107 (85) |
Age (years) | |
Range | 0.0–24.5 |
IQR | 0.5–15.2 |
Median | 4.3 |
Test Characteristics . | N (%) . |
---|---|
Specimen source | |
BAL | 8 (4.7) |
Sputum | 6 (3.5) |
Tracheal aspirate | 155 (92) |
Originating unit | |
Pediatric ICU | 119 (70) |
Cardiac ICU | 26 (15) |
Neonatal ICU | 15 (8.9) |
Pulmonology floor | 4 (2.3) |
Other floor | 4 (2.3) |
Outpatient | 1 (0.6) |
ID consult | |
Yes | 76 (45) |
No | 93 (55) |
Timing | Median (IQR) |
Admission to collection (days) | 6 (2, 19) |
Intubation to collection (days) | 3 (1, 10) |
Collection to result (hours) | 4.9 (4, 6.2) |
Test Characteristics . | N (%) . |
---|---|
Specimen source | |
BAL | 8 (4.7) |
Sputum | 6 (3.5) |
Tracheal aspirate | 155 (92) |
Originating unit | |
Pediatric ICU | 119 (70) |
Cardiac ICU | 26 (15) |
Neonatal ICU | 15 (8.9) |
Pulmonology floor | 4 (2.3) |
Other floor | 4 (2.3) |
Outpatient | 1 (0.6) |
ID consult | |
Yes | 76 (45) |
No | 93 (55) |
Timing | Median (IQR) |
Admission to collection (days) | 6 (2, 19) |
Intubation to collection (days) | 3 (1, 10) |
Collection to result (hours) | 4.9 (4, 6.2) |
Test Characteristics . | N (%) . |
---|---|
Specimen source | |
BAL | 8 (4.7) |
Sputum | 6 (3.5) |
Tracheal aspirate | 155 (92) |
Originating unit | |
Pediatric ICU | 119 (70) |
Cardiac ICU | 26 (15) |
Neonatal ICU | 15 (8.9) |
Pulmonology floor | 4 (2.3) |
Other floor | 4 (2.3) |
Outpatient | 1 (0.6) |
ID consult | |
Yes | 76 (45) |
No | 93 (55) |
Timing | Median (IQR) |
Admission to collection (days) | 6 (2, 19) |
Intubation to collection (days) | 3 (1, 10) |
Collection to result (hours) | 4.9 (4, 6.2) |
Test Characteristics . | N (%) . |
---|---|
Specimen source | |
BAL | 8 (4.7) |
Sputum | 6 (3.5) |
Tracheal aspirate | 155 (92) |
Originating unit | |
Pediatric ICU | 119 (70) |
Cardiac ICU | 26 (15) |
Neonatal ICU | 15 (8.9) |
Pulmonology floor | 4 (2.3) |
Other floor | 4 (2.3) |
Outpatient | 1 (0.6) |
ID consult | |
Yes | 76 (45) |
No | 93 (55) |
Timing | Median (IQR) |
Admission to collection (days) | 6 (2, 19) |
Intubation to collection (days) | 3 (1, 10) |
Collection to result (hours) | 4.9 (4, 6.2) |
The most common bacterial targets identified by the BFPP were Staphylococcus aureus (35%), Haemophilus influenzae (15%), and Pseudomonas aeruginosa (14%) (Table 3). MRSA was identified in 18/59 (31%) Staphylococcus aureus results. Rhinovirus/Enterovirus was the most common viral target identified (21%). No targets were identified on 24% of tests and no subsequent culture growth was seen for 64% of tests. In comparing culture growth to semi-quantitative reporting of BFPP results, only 2% of tests with bacterial targets identified at 104 copies/mL and 3% of tests with bacterial targets at 105 copies/mL had growth of the same organism identified by culture. However, when semi-quantitative values increased to 106 copies/mL and 107 copies/mL, the same organism was identified by culture in 29% and 65% of tests, respectively. The overall concordance between BFPP testing and pathogens identified by culture was 27%. Results were then stratified by days of antibiotic exposure prior to test collection: none, less than 24 h, or greater than 24 h. There was higher concordance for tests without prior exposure to antibiotics (43%, N = 26) compared to less than 24 h (31%, N = 51) or greater than 24 h (19%, N = 92) of antibiotic exposure. The overall trend of higher concordance when semi-quantitative results were 106 or 107 persisted across all groups.
. | N (%) . |
---|---|
Bacterial targets . | . |
Staphylococcus aureus | 59 (35) |
Haemophilus influenzae | 25 (15) |
Pseudomonas aeruginosa | 23 (14) |
Serratia marcescens | 18 (11) |
Moraxella catarrhalis | 16 (9.5) |
Streptococcus pneumoniae | 15 (8.9) |
Klebsiella pneumonia | 11 (6.5) |
Acinetobacter calcoaceticus-baumannii complex | 8 (4.7) |
Enterobacter cloacae complex | 7 (4.1) |
Proteus spp | 7 (4.1) |
Klebsiella oxytoca | 5 (2.9) |
Escherichia coli | 5 (2.9) |
Streptococcus agalactiae | 3 (1.8) |
Streptococcus pyogenes | 3 (1.8) |
Mycoplasma pneumoniae | 1 (0.6) |
No bacterial targets | 53 (31) |
Viral targets | |
Rhinovirus/enterovirus | 36 (21) |
Respiratory syncytial virus | 9 (5.3) |
Metapneumovirus | 8 (4.7) |
Adenovirus | 5 (2.9) |
Coronavirus (non-COVID) | 4 (2.3) |
Influenza A | 1 (0.6) |
No viral targets | 113 (67) |
Resistance genes | |
MecA/C + MREJ | 18 (11) |
CTX-M | 3 (1.8) |
. | N (%) . |
---|---|
Bacterial targets . | . |
Staphylococcus aureus | 59 (35) |
Haemophilus influenzae | 25 (15) |
Pseudomonas aeruginosa | 23 (14) |
Serratia marcescens | 18 (11) |
Moraxella catarrhalis | 16 (9.5) |
Streptococcus pneumoniae | 15 (8.9) |
Klebsiella pneumonia | 11 (6.5) |
Acinetobacter calcoaceticus-baumannii complex | 8 (4.7) |
Enterobacter cloacae complex | 7 (4.1) |
Proteus spp | 7 (4.1) |
Klebsiella oxytoca | 5 (2.9) |
Escherichia coli | 5 (2.9) |
Streptococcus agalactiae | 3 (1.8) |
Streptococcus pyogenes | 3 (1.8) |
Mycoplasma pneumoniae | 1 (0.6) |
No bacterial targets | 53 (31) |
Viral targets | |
Rhinovirus/enterovirus | 36 (21) |
Respiratory syncytial virus | 9 (5.3) |
Metapneumovirus | 8 (4.7) |
Adenovirus | 5 (2.9) |
Coronavirus (non-COVID) | 4 (2.3) |
Influenza A | 1 (0.6) |
No viral targets | 113 (67) |
Resistance genes | |
MecA/C + MREJ | 18 (11) |
CTX-M | 3 (1.8) |
. | N (%) . |
---|---|
Bacterial targets . | . |
Staphylococcus aureus | 59 (35) |
Haemophilus influenzae | 25 (15) |
Pseudomonas aeruginosa | 23 (14) |
Serratia marcescens | 18 (11) |
Moraxella catarrhalis | 16 (9.5) |
Streptococcus pneumoniae | 15 (8.9) |
Klebsiella pneumonia | 11 (6.5) |
Acinetobacter calcoaceticus-baumannii complex | 8 (4.7) |
Enterobacter cloacae complex | 7 (4.1) |
Proteus spp | 7 (4.1) |
Klebsiella oxytoca | 5 (2.9) |
Escherichia coli | 5 (2.9) |
Streptococcus agalactiae | 3 (1.8) |
Streptococcus pyogenes | 3 (1.8) |
Mycoplasma pneumoniae | 1 (0.6) |
No bacterial targets | 53 (31) |
Viral targets | |
Rhinovirus/enterovirus | 36 (21) |
Respiratory syncytial virus | 9 (5.3) |
Metapneumovirus | 8 (4.7) |
Adenovirus | 5 (2.9) |
Coronavirus (non-COVID) | 4 (2.3) |
Influenza A | 1 (0.6) |
No viral targets | 113 (67) |
Resistance genes | |
MecA/C + MREJ | 18 (11) |
CTX-M | 3 (1.8) |
. | N (%) . |
---|---|
Bacterial targets . | . |
Staphylococcus aureus | 59 (35) |
Haemophilus influenzae | 25 (15) |
Pseudomonas aeruginosa | 23 (14) |
Serratia marcescens | 18 (11) |
Moraxella catarrhalis | 16 (9.5) |
Streptococcus pneumoniae | 15 (8.9) |
Klebsiella pneumonia | 11 (6.5) |
Acinetobacter calcoaceticus-baumannii complex | 8 (4.7) |
Enterobacter cloacae complex | 7 (4.1) |
Proteus spp | 7 (4.1) |
Klebsiella oxytoca | 5 (2.9) |
Escherichia coli | 5 (2.9) |
Streptococcus agalactiae | 3 (1.8) |
Streptococcus pyogenes | 3 (1.8) |
Mycoplasma pneumoniae | 1 (0.6) |
No bacterial targets | 53 (31) |
Viral targets | |
Rhinovirus/enterovirus | 36 (21) |
Respiratory syncytial virus | 9 (5.3) |
Metapneumovirus | 8 (4.7) |
Adenovirus | 5 (2.9) |
Coronavirus (non-COVID) | 4 (2.3) |
Influenza A | 1 (0.6) |
No viral targets | 113 (67) |
Resistance genes | |
MecA/C + MREJ | 18 (11) |
CTX-M | 3 (1.8) |
Bacterial detection rates were compared among tracheal aspirate specimens for patients who had a chronic tracheostomy (N = 25) versus those who were newly intubated during the admission (N = 130). On average, tests obtained from patients with chronic tracheostomy identified more bacterial targets per test (2.48 vs 1.02, p < .001), more isolates grown on culture (1.88 vs 1.14, p = .003), and a higher rate of growth of off-panel bacteria on culture (44% vs 4%, p = .004).
Indications for BFPP testing were determined by review of documentation by the primary team and/or ID on the date of test collection (Table 4). The most common indications for test ordering were respiratory failure (36%), suspected pneumonia (15%), and sepsis evaluation without respiratory change (12%). There were 37 repeat BFPP tests in 29 patients, defined as a subsequent test obtained from the same patient during the same admission. The median time between tests was 8.6 days (IQR: 4.9, 13.5). Among repeat tests, 67% identified either the same or fewer targets as a previous test during the same admission.
Indication . | N (%) . |
---|---|
Respiratory failure | 61 (36) |
Pneumonia evaluation | 25 (15) |
Sepsis evaluation without respiratory failure | 21 (12) |
Fever (alone) | 15 (8.9) |
Increased secretions (alone) | 9 (5.3) |
Fever and increased secretions | 6 (3.6) |
Desaturations (alone) | 5 (2.9) |
Desaturations and increased secretions | 5 (2.9) |
Difficulty weaning ventilator | 4 (2.3) |
Unknown indication | 4 (2.3) |
Cardiac arrest | 2 (1.2) |
Seizures | 2 (1.2) |
Tachycardia (alone) | 2 (1.2) |
Dystonia | 1 (0.6) |
Pulmonary edema | 1 (0.6) |
Assess bacterial clearance | 1 (0.6) |
Indication . | N (%) . |
---|---|
Respiratory failure | 61 (36) |
Pneumonia evaluation | 25 (15) |
Sepsis evaluation without respiratory failure | 21 (12) |
Fever (alone) | 15 (8.9) |
Increased secretions (alone) | 9 (5.3) |
Fever and increased secretions | 6 (3.6) |
Desaturations (alone) | 5 (2.9) |
Desaturations and increased secretions | 5 (2.9) |
Difficulty weaning ventilator | 4 (2.3) |
Unknown indication | 4 (2.3) |
Cardiac arrest | 2 (1.2) |
Seizures | 2 (1.2) |
Tachycardia (alone) | 2 (1.2) |
Dystonia | 1 (0.6) |
Pulmonary edema | 1 (0.6) |
Assess bacterial clearance | 1 (0.6) |
Indication . | N (%) . |
---|---|
Respiratory failure | 61 (36) |
Pneumonia evaluation | 25 (15) |
Sepsis evaluation without respiratory failure | 21 (12) |
Fever (alone) | 15 (8.9) |
Increased secretions (alone) | 9 (5.3) |
Fever and increased secretions | 6 (3.6) |
Desaturations (alone) | 5 (2.9) |
Desaturations and increased secretions | 5 (2.9) |
Difficulty weaning ventilator | 4 (2.3) |
Unknown indication | 4 (2.3) |
Cardiac arrest | 2 (1.2) |
Seizures | 2 (1.2) |
Tachycardia (alone) | 2 (1.2) |
Dystonia | 1 (0.6) |
Pulmonary edema | 1 (0.6) |
Assess bacterial clearance | 1 (0.6) |
Indication . | N (%) . |
---|---|
Respiratory failure | 61 (36) |
Pneumonia evaluation | 25 (15) |
Sepsis evaluation without respiratory failure | 21 (12) |
Fever (alone) | 15 (8.9) |
Increased secretions (alone) | 9 (5.3) |
Fever and increased secretions | 6 (3.6) |
Desaturations (alone) | 5 (2.9) |
Desaturations and increased secretions | 5 (2.9) |
Difficulty weaning ventilator | 4 (2.3) |
Unknown indication | 4 (2.3) |
Cardiac arrest | 2 (1.2) |
Seizures | 2 (1.2) |
Tachycardia (alone) | 2 (1.2) |
Dystonia | 1 (0.6) |
Pulmonary edema | 1 (0.6) |
Assess bacterial clearance | 1 (0.6) |
Antibiotic use at the time of BFPP results reflected common use for empiric coverage of pneumonia and sepsis rule outs, with cefepime, vancomycin, and ceftriaxone being the most commonly used agents. Similarly, these agents were the most likely to be started or stopped following BFPP results. The mean time to start a new antibiotic was 7.1 h from time of BFPP result, compared to a mean of 11.0 h to discontinue an antibiotic.
Eighty percent of test results could have led to a change in antibiotic spectrum as adjudicated by two infectious diseases physicians (Figure 1). Results could have led to antibiotic discontinuation in 27%, de-escalation in 31%, and escalation in 21%. However, in practice, the majority of test results (54%) led to no change in antibiotic therapy in the first 24 h. When a change did occur, it was more likely to be an escalation (26%) than a de-escalation (15%) or discontinuation (4.1%).
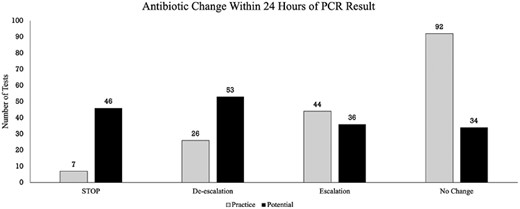
Practice versus potential changes in antimicrobial therapy. Potential changes (black) in antimicrobial therapy following BFPP results are graphed against actual changes that occurred in practice within the first 24 h following results (grey). The four possible actions were defined by a change in the ASI for the total antibiotic regimen pre- and post-BFPP results.
There were 39 cases where a patient was receiving vancomycin and MRSA was not identified on the BFPP, however vancomycin was discontinued in only 13 (33%) patients. Fifty-three BFPP tests did not detect a bacterial target. Among these, six patients were not receiving antibiotics prior to the result and remained off antibiotics. Of the remaining patients who were on antibiotics prior to negative testing for a bacterial target, antibiotics were discontinued in 3/47 (6.4%). Conversely, when patients were off antibiotics and a positive result was reported, antibiotics were started in 15/18 (83%) cases.
DISCUSSION
We conducted the first evaluation of the real-world use of the BFPP in children. We used similar criteria as prior studies to evaluate BFPP potential impact on antibiotic use and, consistent with prior studies, we found that most of the BFPP results could have led to antibiotic changes [7–10]. However, in practice at our institution, antibiotic changes occurred less than half of the time. We identified the potential to de-escalate or stop antibiotics following almost two-thirds of tests, which is similar to the rate reported in the literature [7, 8]. However, discontinuation or de-escalation rarely occurred in practice at our institution.
The discrepancies in this test’s potential and performance at our institution may be related to the patient population (ie, children) and the indications for which the test is being sent (eg, sepsis rule out). The BFPP may be a more invasive test in children, who do not reliably produce sputum and subsequently the majority of tests in our study were obtained from tracheal aspirate specimens. Similarly, although previous studies have shown higher rates of discontinuation or de-escalation of antibiotic therapy in response to BFPP results, these almost exclusively included adult patients with presumed pneumonia. Concern for pneumonia was documented in only 15% of cases in our cohort, compared to published studies that focus on evaluation of CAP, hospital-acquired pneumonia, and/or ventilator-associated pneumonia, or identifying bacterial co-infection in adults with COVID-19 [8–20, 22–25]. In this study, the BFPP was often used as part of a broader sepsis evaluation in children, even when there were no changes in respiratory status. However, even when tests were used for changes in respiratory status, the most likely action was for no change in antibiotic therapy within 24 h of results, which may reflect that the test is being used as part of a larger evaluation, or may indicate provider uncertainty in rapid diagnostic test results. Repeat testing and specimens from patients with chronic tracheostomies, which was not uncommon in our study, are also not reported in the literature. Repeat testing rarely provided new information, while tests from patients with chronic tracheostomy often identified multiple targets with a high likelihood of off-panel culture growth, making them difficult to interpret.
The variability in indications for testing and the relative lack of impact of BFPP testing on antibiotic use may also reflect known challenges in implementation of rapid diagnostics. Previous studies evaluating rapid diagnostic testing for blood cultures have shown that implementation of a test alone may not decrease unnecessary antibiotic use. Rather, implementation of rapid diagnostics in conjunction with clinical feedback, such as real-time prospective audit and feedback by members of an ASP, can be highly effective [26–28]. Our hospital has a well-established ASP that provided general guidance to clinicians on optimal use of the BFPP via an institutional guideline and through handshake stewardship rounds. It was recommended to generally use the test primarily for children with severe pneumonia who were newly intubated (rather than in patients with chronic tracheostomy), and to use negative MRSA results to discontinue vancomycin. However, there were no restrictions on the use of the test and no approval required to order. The SLCH ASP team performs daily handshake stewardship rounds Monday through Friday and often provided feedback on testing during rounds, addressing both interpretation of results and appropriateness of ordering. However, there was no formal process to audit or provide guidance for every BFPP result and data was not collected on how frequently recommendations were provided or how often they were followed by the primary team. A recent study by Miller et. al. similarly showed no significant difference in time to de-escalation of anti-MRSA or anti-pseudomonal agents when comparing cohorts from pre- and post-BFPP implementation [20]. More intensive stewardship oversight may improve the utility of the BFPP results. This has been best illustrated by Esplund et al, who paired written communication with educational sessions for their ICU providers and pharmacists [10]. Additionally, all BFPP results were reviewed by critical care clinical pharmacists between 0700 and 2200, who provided real-time guidance in result interpretation and clinical decision making. They found that when the BFPP results suggested a potential antibiotic change, there were more de-escalations (41%) than escalations (12.5%) in antibiotic therapy. However, no change in therapy was still the most common outcome (46%).
There are also intrinsic challenges in microbiologic testing of upper or lower respiratory tract specimens, namely that these are not sterile spaces and it is difficult to distinguish oral contamination versus colonization versus infection, which may drive unnecessary antibiotic use. A multicenter review of endotracheal aspirate cultures sent from 31 pediatric hospitals found that culture rate was highly variable across institutions and that culture rate positively correlated with hospital antibiotic use (R = 0.46, p = .009) [29]. Each endotracheal aspirate culture increased the odds of additional days of antibiotics by 2.87 (95% CI, 2.74–3.01). Compared to culture alone, there is an increased sensitivity with PCR panels, as demonstrated by our correlation data. This increased sensitivity could lead to an even greater increase in antibiotic use, although additional studies are needed to appropriately assess a correlation. We were unable to clearly evaluate the relationship between use of the BFPP, use of traditional endotracheal aspirate cultures alone, and overall antibiotic use in this study. Our institution participated in a multicenter quality improvement initiative to reduce overall use of endotracheal aspirate cultures that coincided with introduction of the BFPP, making any changes in endotracheal aspirate culture rates or associated antibiotic use difficult to interpret.
We suggest that ASPs seeking to effectively implement the BFPP in children should focus on three areas. (1) Education for providers about indications for use, primarily recommending use in newly intubated patients with respiratory symptoms and avoidance of testing when infection is unlikely. (2) Active ASP or pharmacy intervention to communicate results and recommendations for antibiotic changes in real-time. (3) Incorporation of existing diagnostic stewardship practices around respiratory cultures, avoidance of testing patients with chronic tracheostomy, and consideration of lockout periods for repeat testing [30].
Our study has several limitations. This was a single-center review of the use of BFPP at a tertiary children’s hospital and results may not be generalizable to all settings. However, the breakdown of the BFPP test characteristics highlights important distinctions between the use of this test in children versus adults. Our methodology was intentionally chosen to replicate previous studies that have proposed the BFPP as an ASP tool, and thus focused solely on BFPP results and did not consider concurrent cultures, other rapid diagnostic tests, or other clinical factors in determining potential antibiotic changes. It is possible that provider decision making was dependent on information from cultures beyond the BFPP, which could explain the predominance of no change in antibiotic therapy within 24 hours of PCR results in our data. However, this suggests that the BFPP did not contribute significantly to clinical decision making in our cohort. Additionally, the review was focused on patients in whom the BFPP was collected, so there was no comparator group without BFPP testing. The study was also not designed for assessment of patient outcomes based on test results and antibiotic selections.
CONCLUSIONS
In conclusion, our study represents the first real-world evaluation of the BFPP in a pediatric hospital. Indications for testing varied greatly in this pediatric population and the test was not used exclusively to evaluate pneumonia. Less than half of BFPP results were associated with a change in antibiotics, despite the potential for antibiotic change following 80% of results. Combining implementation of the BFPP with real-time clinical guidance, decision support provided by ASP or pharmacists, and diagnostic stewardship efforts may be more effective than implementation of the test alone.
Financial support. This work was supported by a grant from the National Institutes of Health (T32 AI106688 to A. S. P.).
Potential conflicts of interest. R. B. and R. D. have both received partial support for previous research studies from Biomerieux, but were not related to the Pneumonia Panel reviewed in this study. J. G. N. has grant funding from Moderna, Pfizer, AHRQ, and the NIH, but nothing related to this work. A. S. P., C. R. L., and R. G. S. have no conflicts of interest to disclose.
Data availability. The datasets generated during and/or analyzed during the current study are available from the corresponding author on reasonable request.