-
PDF
- Split View
-
Views
-
Cite
Cite
Kechao Huang, Yongmei Liao, Tingfa Dong, Yanxia Yang, Jundong He, Huihui Huan, Qibing Zhang, Xiao Xu, Sex-specific responses of tree-ring growth to climate in the dioecious tree Populus cathayana, Journal of Plant Ecology, Volume 11, Issue 5, October 2018, Pages 771–779, https://doi.org/10.1093/jpe/rtx048
- Share Icon Share
Abstract
Radial growth in response to climate has been reported in many trees, but the sex-specific responses of tree-ring growth associated with altitude in dioecious trees are still poorly known. This study aims to examine whether (i) there are sex-related responses of tree-ring growth to climate in dioecious trees; (ii) these responses could be changed with altitude elevation.
The tree-ring width and basal area increment (BAI) were measured over the past 30 years (1982–2011), and the sexual differences in relationship between BAI and time span and correlations between ring width and climatic factors were investigated in Populus cathayana trees at two altitude sites (1,450 m and 1,750 m a.s.l.) in Xiaowutai Mountain, Hebei, north China.
The BAI was increased over the past 30 years. Trees at high-altitude sites had significantly lower mean ring width and mean BAI than those trees at low-altitude sites (P < 0.001). In addition, sexual differences in tree-ring growth and its response to climate were more pronounced by altitude elevation. Male trees had a significantly larger mean ring width and mean BAI than did females at high-altitude sites, whereas no significant sexual differences in these traits were detected at low-altitude sites. Female trees were sensitive to previous October–November temperatures at high altitude but to current February and April precipitation at low altitude (P < 0.05), whereas male trees were sensitive to current June temperature at high altitude but to January precipitation at low altitude (P < 0.05). Our results indicated that the responses of tree-ring growth to climate are sex dependent and can be changed with altitude elevation.
INTRODUCTION
The responses of tree-ring growth to climate factors, such as temperature, precipitation and their interactions have attracted widespread attention (Bräuning et al. 2004; Briffa et al. 1998; Büntgen et al. 2011; Esper et al. 2002; Frank et al. 2005; Körner and Basler 2010). For example, ring width was reported to be positively correlated with temperature in Pinus sylvestris (Grace and Norton 1990) and was strongly increased by previous August rainfall in Abies alba in the internal Alps (Rolland 1993). In central Canada, ring width was promoted by a cooler and wetter climate in black spruce (Picea mariana), while it was enhanced by higher temperature and spring precipitation in jack pine (Pinus banksiana) (Brooks et al. 1998). Additionally, ring width was reported to be increased by warm autumn or winter in A. alba, Pinus nigra, P. sylvestris and P. uncinata in Mediterranean mountains (Lebourgeois et al. 2012). As another character of tree-ring growth, tree-ring density was also reported to be affected by summer temperature in Abies lasiocarpa in British Columbia (Splechtna et al. 2000) or in Picea brachytyla in the central Hengduan Mountains of southern China (Fan et al. 2009). Moreover, tree-ring maximum density was positively correlated with mean temperature of August and September in Balfour spruce (Picea likiangensis var. balfouriana) in eastern Tibet of China (Wang et al. 2010), whereas it was closely related with temperature during whole growing season in white spruce (Picea glauca) in the highlands of southeastern New York (D’Arrigo et al. 1992). Although above-mentioned studies have confirmed the close relationship between tree-ring growth and climate, knowledge about sexual differences in tree-ring growth in response to climatic factors is still limited.
As an important component of terrestrial ecosystems, dioecious plants account for nearly 6% of all angiosperm species (Renner and Ricklefs 1995). Since attention has been paid to these plants in recent years, sexual differences in adaptive capacity have been widely studied in plants Populus cathayana, P. tremuloides, P. yunnanensis, Salix arctica, S. myrsinifolia, Silene latifolia and so on (e.g. Chen et al. 2014; Dong et al. 2017; Jones et al. 1999; Nybakken and Julkunen-Tiitto 2013; Randriamanana et al. 2015; Wang and Curtis 2001; Wang and Griffin 2003; Xu et al. 2008, 2010). In general, male trees were reported to possess higher adaptive capacity than females under drought, elevated CO2, enhanced UV-B, higher temperatures, or combinations because of few reproductive inputs (Chen et al. 2016; Xu et al. 2008, 2010; Zhao et al. 2012). However, little attention has been paid to sex-specific tree-ring growth (except for Ariel and Alejandro 2016; Gao et al. 2010; Olano et al. 2015; Rozas et al. 2009).
Altitude is a geographic factor associated with temperature, precipitation, light and soil (He et al. 2017; Jin et al. 2016; Kienast et al. 1987; Körner 2007; Mai et al. 2017). Although most studies demonstrated the tree-ring growth and its responses to climatic factors could be affected by altitude (Liang et al. 2010; Lloyd et al. 2011; Splechtna et al. 2000; Wang et al. 2005; Zhang and Hebda 2004), few studies focused on the gender-related differences in responses of trees to climate change. Since males could display higher adaptive capacity than females under environmental stress (Li et al. 2014; Xu et al. 2008, 2010; Zhao et al. 2012), we hypothesized that sex-specific responses of tree-ring growth to climate would be changed with altitude elevation, and sexual differences in such responses are more pronounced at high-altitude sites because of a harsh environment (e.g. low temperature, strong UV-B radiation or poor soil). To test our hypothesis, Populus cathayana Rehd., a dioecious woody tree which is widely distributed in northern, central and southwestern China was chosen, and sexual differences in tree-ring width, basal area increment (BAI), scaling relationship between BAI and time (which is a index to test for a common slope or elevation among lines fitted to different groups), as well as the correlations between tree ring growth and climatic factors were investigated in the study.
MATERIALS AND METHODS
Study area and sampling
Study area was located in the Xijin Valley of the Xiaowutai Mountain Nature Reserve in Hebei, China (39°50′–40°07′N, 114°47′–115°30′E). This site is characterized by a warm-temperate continental monsoon climate, which has an average annual temperature of 5.5°C (−12.0°C in January and 19.5°C in July) and a mean annual precipitation of 628 mm (climate data acquired from http://www.meteoblue.com). Soil types are classified as alfisols, aridisols and inceptisols (Yu et al. 2002). The forest vegetation is dominated by species in the Acer, Tilia, Betula, Corylus, Quercus, Ulmus, Populus and Cerasus genera. P. cathayana is a dominant tree species in valleys and is widely distributed between 1,400–1,700 m a.s.l. (Yang et al. 2015).
Two sampling sites were established at 1,450 and 1,750 m a.s.l. along the Xijin River Valley in the middle of April (the beginning of the P. cathayana flowering season). Seventy mature trees (35 males and 35 females) with 30 to 45-cm diameter at breast height (~50 years old) were randomly selected for collecting tree-core samples at each site. The increment cores were taken at breast height (1.3 m) from each tree using an increment borer (length 600 mm, diameter 4.35 mm) (Increment Borer, Haglof, Langsele, Sweden). Poor-quality sample cores (such as pith missed, decay, erosion or broken pieces) were removed from further analyses. Descriptive statistics for tree-ring samples were listed in Table 1.
descriptive statistics for tree-ring samples of female and male Populuscathayana
Sex . | Low altitude . | High altitude . | ||
---|---|---|---|---|
Female . | Male . | Female . | Male . | |
No. of sample trees | 35 | 35 | 35 | 35 |
Sample size | 35 | 35 | 35 | 35 |
Chronology duration | 1965–2011 | 1963–2011 | 1960–2011 | 1959–2011 |
Time span (a) | 47 | 49 | 52 | 53 |
Common period | 1982–2011 | 1982–2011 | 1982–2011 | 1982–2011 |
No. of samples in common period | 25 | 21 | 35 | 35 |
Diameter (cm) (1.3 m above ground) | 37.5 ± 2.1b | 38.5 ± 1.7b | 37.5 ± 1.5b | 43.4 ± 1.5a |
Sex . | Low altitude . | High altitude . | ||
---|---|---|---|---|
Female . | Male . | Female . | Male . | |
No. of sample trees | 35 | 35 | 35 | 35 |
Sample size | 35 | 35 | 35 | 35 |
Chronology duration | 1965–2011 | 1963–2011 | 1960–2011 | 1959–2011 |
Time span (a) | 47 | 49 | 52 | 53 |
Common period | 1982–2011 | 1982–2011 | 1982–2011 | 1982–2011 |
No. of samples in common period | 25 | 21 | 35 | 35 |
Diameter (cm) (1.3 m above ground) | 37.5 ± 2.1b | 38.5 ± 1.7b | 37.5 ± 1.5b | 43.4 ± 1.5a |
Means with different letters are significantly different at the 0.05 level (Duncan’s multiple range test).
descriptive statistics for tree-ring samples of female and male Populuscathayana
Sex . | Low altitude . | High altitude . | ||
---|---|---|---|---|
Female . | Male . | Female . | Male . | |
No. of sample trees | 35 | 35 | 35 | 35 |
Sample size | 35 | 35 | 35 | 35 |
Chronology duration | 1965–2011 | 1963–2011 | 1960–2011 | 1959–2011 |
Time span (a) | 47 | 49 | 52 | 53 |
Common period | 1982–2011 | 1982–2011 | 1982–2011 | 1982–2011 |
No. of samples in common period | 25 | 21 | 35 | 35 |
Diameter (cm) (1.3 m above ground) | 37.5 ± 2.1b | 38.5 ± 1.7b | 37.5 ± 1.5b | 43.4 ± 1.5a |
Sex . | Low altitude . | High altitude . | ||
---|---|---|---|---|
Female . | Male . | Female . | Male . | |
No. of sample trees | 35 | 35 | 35 | 35 |
Sample size | 35 | 35 | 35 | 35 |
Chronology duration | 1965–2011 | 1963–2011 | 1960–2011 | 1959–2011 |
Time span (a) | 47 | 49 | 52 | 53 |
Common period | 1982–2011 | 1982–2011 | 1982–2011 | 1982–2011 |
No. of samples in common period | 25 | 21 | 35 | 35 |
Diameter (cm) (1.3 m above ground) | 37.5 ± 2.1b | 38.5 ± 1.7b | 37.5 ± 1.5b | 43.4 ± 1.5a |
Means with different letters are significantly different at the 0.05 level (Duncan’s multiple range test).
Tree-ring width measurement
Following the method described by Cook and Kairiukstis (1990), 140 core samples were dried for 1 week at room temperature, and then glued onto wooden core holders and polished with a sanding machine until the cellular structure of the xylem was clearly visible. A Lintab 5 measuring system (Rinntech, Heidelberg, Germany) and COFECHA software (Holmes 1983) were used to measure tree-ring width (to the nearest 0.001 mm) and control the cross-matching quality of the different series, respectively. Additionally, BAI was calculated according to the formula ARAt = π (Rt2 – Rt−12) described by Biondi and Qeadan (2008), where Rt is the stem radius at the end of the annual increment, and Rt−1 is the stem radius at the beginning of the annual increment.
Chronology construction
The residual chronology was applied to the climate-growth response analysis. To remove non-climatic factors (e.g. age), each series was fitted to a spline function with a 50 % frequency response of 30 years using the ARSTAN programme (Cook and Holmes 1996). Chronologies for the common period of 1982–2011 were compared and the inter-series correlation was calculated using a 20-year moving window with a 10-year overlap. The standard deviation (SD), mean sensitivity (MS), mean correlations among cores (R), signal-to-noise ratio (SNR), expressed population signal (EPS) and explained variance of the first eigenvector (PCI) were subsequently calculated. Moreover, monthly mean temperature and total precipitation data for the period 1982–2011 were collected from the local meteorological station which is located ~20 km northwest of the sampling sites. These data were used for calculating correlation coefficients between climatic factors and tree-ring chronology because of the dramatic climate changes in this period in China (Zhang et al. 2013).
Data analysis
Dendroclimatic analysis was performed with the DendroClim2002 software package and 1000 bootstrapped samples were used to compute response and correlation coefficients (Biondi and Waikul 2004; Zhang et al. 2003). These correlation analyses were conducted from September of the previous year to October of the current year, and significance was set at the P < 0.05 level.
Scaling relationships between BAI and time span were analyzed by using the SMATR version 2.0 (Falster et al. 2006). Confidence intervals for individual regression slopes were calculated according to Pitman (1939), and heterogeneity of regression slopes (used to test whether the slopes are significantly different) between the sexes and common slopes (used to test whether the fitted slopes share a common elevation) were calculated followed Warton and Weber (2002).
Statistical analyses were performed using the SPSS 19.0 for Windows statistical software package (IBM Corp., New York, USA). Data were checked for normality and homogeneity of variances, and log-transformed to correct deviations from these assumptions when needed. Stepwise linear regressions were used to characterize trends in annual mean temperature and annual precipitation over the 1982–2011 period. One-way analyses of variance (ANOVAs) were used to determine differences in tree-ring width between the sexes or altitude sites. Duncan’s multiple range tests were used to detect differences among means. Two-way ANOVAs were used to assess the effects of sex, altitude and their interactive effects. Differences were considered significant at P <0.05 level.
RESULTS
Trends in annual mean temperature and annual precipitation
Annual precipitation was fluctuating during the period of 1982–2011, and no significant change was found (R2 = 0.057, P = 0.217) (Fig. 1). However, annual mean air temperature significantly increased from 6.5°C to 8.3°C over the past 30 years (R2 = 0.560, P < 0.001), indicating a warming trend in this region (Fig. 1).
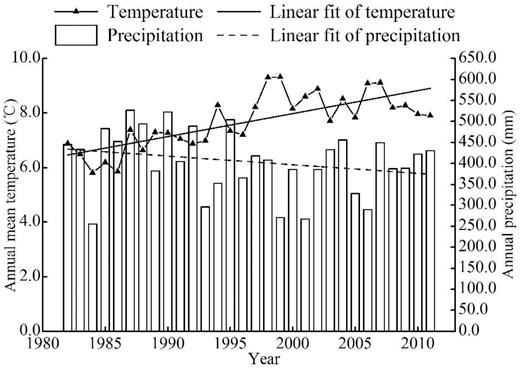
distribution of annual precipitation (bars) and mean air temperature (line with triangles) and fit line of annual precipitation (dotted line) and fit line of annual air temperature (solid line) during the period of 1982–2011 at the Yuxian Meteorological Station, China.
Sexual difference in relationships between BAI and time span at high- and low-altitude sites
During the period of 1982–2011, BAI was observed to be inconsistent with an upward fluctuation, and this trend was more pronounced at low-altitude site (Fig. 2a). Additionally, male trees had greater BAI than females at high-altitude site (Fig. 2a). On the other hand, for two sexes, BAI was positively correlated with time span at both high- and low-altitude sites. Females have common slopes of 0.746 and 0.629 with males at high- and low-altitude sites, respectively (Table 2; Fig. 2b). Moreover, a significant shift in the y-intercept was found between the sexes (P = 0.001) and male trees exhibited larger value than females at high-altitude sites (Table 2; Fig. 2b), suggesting that the males had larger BAI per unit time span than the females. However, similar result was not found at low-altitude sites (Table 2; Fig. 2b).
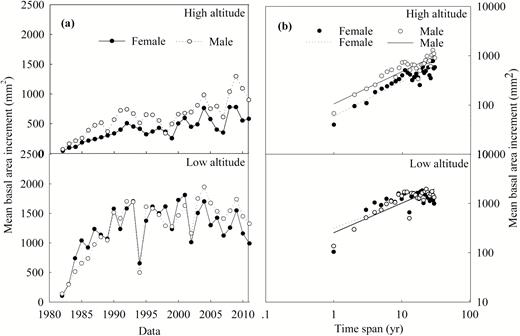
(a) The basal area increment (BAI) series between the sexes during the period of 1982–2011 and (b) scaling relationships between BAI and time span of male and female Populus cathayana at high- and low-altitude sites in the Xiaowutai Mountains.
standardized major axis common slopes, 95% confidence intervals (CIs) (for the sexes) and SMA regression parameters between basal area increment (BAI) and time span from female and male Populus cathayana trees
y–x . | Sex . | Slope . | r2 . | y-intercept . | P(Slope) . | Common slope . | CIs . | P(y-intercept) . |
---|---|---|---|---|---|---|---|---|
High altitude | Female | 0.766 | 0.849*** | 1.731 | 0.621 ns | 0.746 | 0.669–0.831 | 0.001*** |
Male | 0.723 | 0.824*** | 1.926 | |||||
Low altitude | Female | 0.680 | 0.559*** | 2.300 | 0.847 ns | 0.692 | 0.593–0.808 | 0.923 ns |
Male | 0.700 | 0.732*** | 2.304 |
y–x . | Sex . | Slope . | r2 . | y-intercept . | P(Slope) . | Common slope . | CIs . | P(y-intercept) . |
---|---|---|---|---|---|---|---|---|
High altitude | Female | 0.766 | 0.849*** | 1.731 | 0.621 ns | 0.746 | 0.669–0.831 | 0.001*** |
Male | 0.723 | 0.824*** | 1.926 | |||||
Low altitude | Female | 0.680 | 0.559*** | 2.300 | 0.847 ns | 0.692 | 0.593–0.808 | 0.923 ns |
Male | 0.700 | 0.732*** | 2.304 |
P(Slope), level of significance testing for slope; P(y-intercept), level of significance testing for y-intercept between genders; r2, correlation coefficients. The significance values of analyses are denoted as: ns, not significant; ***P < 0.001.
standardized major axis common slopes, 95% confidence intervals (CIs) (for the sexes) and SMA regression parameters between basal area increment (BAI) and time span from female and male Populus cathayana trees
y–x . | Sex . | Slope . | r2 . | y-intercept . | P(Slope) . | Common slope . | CIs . | P(y-intercept) . |
---|---|---|---|---|---|---|---|---|
High altitude | Female | 0.766 | 0.849*** | 1.731 | 0.621 ns | 0.746 | 0.669–0.831 | 0.001*** |
Male | 0.723 | 0.824*** | 1.926 | |||||
Low altitude | Female | 0.680 | 0.559*** | 2.300 | 0.847 ns | 0.692 | 0.593–0.808 | 0.923 ns |
Male | 0.700 | 0.732*** | 2.304 |
y–x . | Sex . | Slope . | r2 . | y-intercept . | P(Slope) . | Common slope . | CIs . | P(y-intercept) . |
---|---|---|---|---|---|---|---|---|
High altitude | Female | 0.766 | 0.849*** | 1.731 | 0.621 ns | 0.746 | 0.669–0.831 | 0.001*** |
Male | 0.723 | 0.824*** | 1.926 | |||||
Low altitude | Female | 0.680 | 0.559*** | 2.300 | 0.847 ns | 0.692 | 0.593–0.808 | 0.923 ns |
Male | 0.700 | 0.732*** | 2.304 |
P(Slope), level of significance testing for slope; P(y-intercept), level of significance testing for y-intercept between genders; r2, correlation coefficients. The significance values of analyses are denoted as: ns, not significant; ***P < 0.001.
Sexual difference in mean ring width and mean BAI at low- and high-altitude sites
The tree-ring growth was significantly affected by altitude. Compared with low-altitude trees, high-altitude trees had significantly smaller mean ring width and mean BAI in both males and females (P < 0.001) (Fig. 3). Furthermore, sexual difference in tree-ring growth was induced by altitude elevation, and male trees exhibited significant larger mean ring width and mean BAI than females at high-altitude sites (P = 0.032 and 0.043, respectively), whereas no significant sexual differences in these traits were detected at low-altitude sites (Fig. 3)
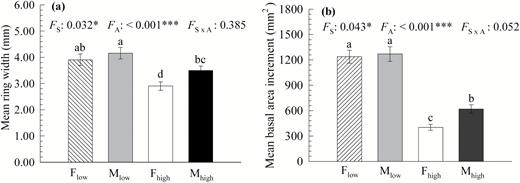
(a) Mean ring width and (b) mean basal area increment (BAI) of male and female Populus cathayana trees at low- and high-altitude sites in the Xiaowutai Mountains. The values are expressed as the means ± SE (n = 35). Different letters above the bars denote statistically significant differences between sexes and altitudes at P < 0.05 based on Duncan’s multiple range test. Abbreviations: FA, altitude effect; FS, sex effect; FS×A, the interactive effect of sex and altitude. Fhigh or Mhigh, female or male at high-altitude sites; Flow or Mlow, female or male at low-altitude sites.
Sexual differences in chronological characteristics at high- and low-altitude sites
Compared with chronologies of low-altitude trees, high-altitude trees exhibited relatively lower SD and MSs but higher SNR and EPS. On the other hand, male trees had lower SD but higher MS, SNR and EPS than females at high-altitude sites, whereas the opposite results were observed at low-altitude sites (Table 3). However, the mean correlations among all cores (R) and the variance in the first eigenvector (%) were always greater in male trees regardless of altitude (Table 3).
statistical characteristics of the residual chronology of female and male Populus cathayana trees (1982–2011)
Statistical item . | Low altitude . | High altitude . | ||
---|---|---|---|---|
Female . | Male . | Female . | Male . | |
Standard deviation (SD) | 0.208 | 0.169 | 0.191 | 0.186 |
Mean sensitivity (MS) | 0.257 | 0.212 | 0.207 | 0.210 |
Mean correlations among all cores (R) | 0.264 | 0.277 | 0.238 | 0.350 |
Signal-to-noise ratio (SNR) | 8.960 | 8.028 | 10.928 | 18.890 |
Expressed population signal (EPS) | 0.900 | 0.889 | 0.916 | 0.950 |
Variance in the first eigenvector (%) (PCI) | 31.5 | 32.4 | 29.6 | 39.9 |
Statistical item . | Low altitude . | High altitude . | ||
---|---|---|---|---|
Female . | Male . | Female . | Male . | |
Standard deviation (SD) | 0.208 | 0.169 | 0.191 | 0.186 |
Mean sensitivity (MS) | 0.257 | 0.212 | 0.207 | 0.210 |
Mean correlations among all cores (R) | 0.264 | 0.277 | 0.238 | 0.350 |
Signal-to-noise ratio (SNR) | 8.960 | 8.028 | 10.928 | 18.890 |
Expressed population signal (EPS) | 0.900 | 0.889 | 0.916 | 0.950 |
Variance in the first eigenvector (%) (PCI) | 31.5 | 32.4 | 29.6 | 39.9 |
statistical characteristics of the residual chronology of female and male Populus cathayana trees (1982–2011)
Statistical item . | Low altitude . | High altitude . | ||
---|---|---|---|---|
Female . | Male . | Female . | Male . | |
Standard deviation (SD) | 0.208 | 0.169 | 0.191 | 0.186 |
Mean sensitivity (MS) | 0.257 | 0.212 | 0.207 | 0.210 |
Mean correlations among all cores (R) | 0.264 | 0.277 | 0.238 | 0.350 |
Signal-to-noise ratio (SNR) | 8.960 | 8.028 | 10.928 | 18.890 |
Expressed population signal (EPS) | 0.900 | 0.889 | 0.916 | 0.950 |
Variance in the first eigenvector (%) (PCI) | 31.5 | 32.4 | 29.6 | 39.9 |
Statistical item . | Low altitude . | High altitude . | ||
---|---|---|---|---|
Female . | Male . | Female . | Male . | |
Standard deviation (SD) | 0.208 | 0.169 | 0.191 | 0.186 |
Mean sensitivity (MS) | 0.257 | 0.212 | 0.207 | 0.210 |
Mean correlations among all cores (R) | 0.264 | 0.277 | 0.238 | 0.350 |
Signal-to-noise ratio (SNR) | 8.960 | 8.028 | 10.928 | 18.890 |
Expressed population signal (EPS) | 0.900 | 0.889 | 0.916 | 0.950 |
Variance in the first eigenvector (%) (PCI) | 31.5 | 32.4 | 29.6 | 39.9 |
Sexual differences in climate responses at high- and low-altitude sites
Ring width was negatively correlated with previous October–November temperatures in females but with current June temperature in males at high-altitude sites (P < 0.05), whereas it was negatively correlated with current January and August temperatures in female, but only with current January temperature in males at low-altitude sites (Fig. 4a).
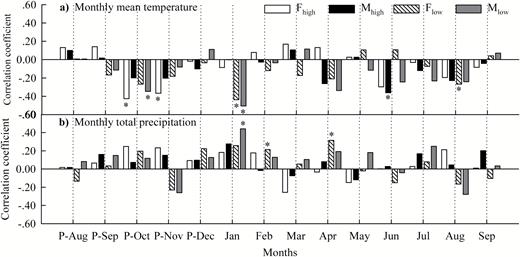
correlation coefficients between residual chronologies and (a) monthly mean temperatures and (b) monthly total precipitation during the period of 1982–2011. In the figure, P-August−September refers to the previous August to the current September, and the asterisk indicates a 0.05 significance level. Fhigh or Mhigh: female or male at high-altitude sites; Flow or Mlow: female or male at low-altitude sites.
Furthermore, ring width was positively correlated with current February and April precipitation in females but with current January precipitation in males at low-altitude sites (P < 0.05). However, no significant correlations were found between ring width and precipitation for either males or females at high-altitude sites (Fig. 4b).
DISCUSSION
In this study, we determined that annual mean temperature in the Xiaowutai Mountains had increased by an average of 1.8°C over the past 30 years (Fig. 1). The warming trend is consistent with the prediction of Intergovernmental Panel on Climate Change (IPCC) that annual mean temperatures are likely to increase by 1.5–4.0°C in continental areas of the Northern Hemisphere by the end of the 21st century (Stocker 2014). As a result, the growth of trees would be promoted by warming because of the enhanced enzyme activity and the extended duration of favourable temperatures (Chmura et al. 2011; Luong et al. 2013). As we predicted, BAI of P. cathayana trees significantly increased during the period of 1982–2011 (Fig. 2). Similar results that warming accelerates tree growth were also reported in tree species Abies fargesii, A. chensiensis, Cryptomeria japonica, Larix chinensis, Pinus densiflora, P. ponderosa, P. tabulaeformis, Pseudotsuga menziesii, Quercus alba and Tsuga canadensis in North American, Northwest China and South Korea (Black et al. 2008; Liu et al. 2013; Luong et al. 2013).
In addition to warming, altitude is another factor to affect tree-ring growth (He et al. 2013; Lv and Zhang 2012; Splechtna et al. 2000; Zhang and Wilmking 2010). A change in altitude is associated with a change in temperature, precipitation, soil, light, humid as well as UV-B radiation. The precipitation generally increases with altitude elevation, whereas temperature decreases with elevation. In our study, P. cathayana trees displayed a significantly smaller mean ring width and mean BAI at high-altitude sites (P < 0.001; Fig. 3). In general, trees at high-altitude sites are usually subjected to a colder, humid and strong UV-B radiation environment, and have a lower growth rate and shorter growth season (Peng et al. 2008). Therefore, tree-ring growth is negative correlated with altitude elevation. Similar results were reported for trees in the Qilian, Anyemaqen, Emei and Sygera mountain ranges, as well as for trees in the southern part of the Qinghai-Tibet Plateau in China (e.g. Fang et al. 2010; Gou et al. 2005; Liang et al. 2010; Liu et al. 2013; Peng et al. 2008). Moreover, we found that radial growth was significantly negatively correlated with mean January temperature at low altitude, but it was not found at high altitude (Fig. 4a). It seems that there exist different growth sensitivities to temperature between high- and low-altitude trees. Since most deciduous trees require a specific minimum winter temperature to break dormancy (Faust et al. 1997), warmer winter temperature would be detrimental to dormancy and early-spring sprouting of trees, especially at low-altitude sites in the Northern Hemisphere (D’Arrigo et al. 2001; Wang et al. 2005). Simultaneously, results of residual chronology analysis also showed that ring width chronologies from low-altitude trees had relatively higher MS and lower SNR than chronologies from high-altitude trees (Table 3).
On the other hand, the result of Duncan’s multiple range tests showed that male trees had significantly wider mean ring width and more mean BAI than females at high-altitude sites(P = 0.032 and 0.043, respectively), whereas no significant differences between two sexes were detected at low-altitude sites (Fig. 3). It suggested that sexual differences in tree-ring growth were enlarged by increasing altitude. Previous studies reported that male and female P. cathayana individuals had different adaptive capability under environmental stress (Chen et al. 2010, 2016; Li et al. 2014); Xu et al. 2008, 2010; Zhang et al. 2011. For example, chilling-stressed males exhibited a better chloroplast structure and higher osmotic adjustment substance contents than females (Zhang et al. 2011). Drought-stressed males had higher photosynthetic ability than females (Xu et al. 2008). UV-B radiation-stressed males had a more efficient antioxidant system and higher anthocyanin content to alleviate UV-B penetration stress than females (Xu et al. 2010). Under nitrogen or phosphorus deficit environment, females suffered more negative effects on photosynthesis, organelle ultrastructures and nitrogen metabolic processes than did males (Zhang et al. 2014). According to Zhang et al. (2016), sexual differences in adaptive capability are the result of abundant sex-specifically expressed proteins, which involved with photosynthesis, carbohydrate metabolism, lipid metabolism, secondary metabolism, oxidation reduction, signal transduction, as well as gene expression regulation. Female plants usually express fewer proteins than males under environmental stress, which results in a weak adaptive capability (Zhang et al. 2016). Hence, with the increasing of altitude, habitat is gradually replaced by a stress environment with cold air, infertile soil and higher UV-B radiation. Male P. cathayana trees would be induced to express more functional proteins than females to adapt stress environment, and result in a wider ring width and more BAI at high-altitude sites.
The phenomenon that the sensitivity of tree-ring variability to climate is sex dependent has been reported in dioecious plants Araucaria araucana, Fraxinus mandschurica, Juniperus communis, J. thurifera, Populus tremuloides and Taxus baccata (Ariel and Alejandro 2016; Cedro et al. 2011; Gao et al. 2010; Grant and Mitton 1979; Iszkuło and Boratyński 2011; Iszkuło et al. 2011; Olano et al. 2015). For instance, female F. mandshurica and J. communis trees were sensitive to precipitation, whereas males were sensitive to previous November temperature (Gao et al. 2010; Iszkuło and Boratyński 2011). Female J. thurifera trees were more sensitive to summer water stress and displayed stronger ring-width responses than males (Olano et al. 2015; Rozas et al. 2009). Female A. araucana trees are more sensitive to air surface temperature but males appear to be more sensitive to precipitation (Ariel and Alejandro 2016). To our knowledge, dioecious plants may evolve alternative optimal strategies to maximize reproductive success when resources are limited (Charnov 1982) and females usually invest more resources into reproduction and less into growth than do males (Delph 1990; Cipollini and Whigham 1994), which would result in different strategies between two sexes for the acquiring and deploying resources (Sánchez Vilas and Pannell 2011), such as females substantial demand carbon and water for the production of flowers, seeds and fruits, while males particularly require nitrogen and phosphorus for production of pollen (Ashman 1994; Bloom et al. 1985; Obeso 2002). As a result, sexual dimorphism in resource acquirement and investment would lead to different growth strategies in response to climate factors between two sexes. In present study, we found that female trees were sensitive to previous October–November temperatures at high-altitude sites but to current February and April precipitation at low-altitude sites, whereas male trees were sensitive to current June temperature at high-altitude sites but to January precipitation at low-altitude sites (Fig. 4). So, our study on P. cathayana trees also confirmed the sensitivity of tree-ring variability to climate is sex and altitude dependent.
In conclusion, our results showed that the tree-ring growth of P. cathayana was increased during the period of 1982–2011. Mean ring width and mean BAI were both significantly higher in males than in females at high altitude, whereas no significant sexual differences were found at low altitude. Female trees were sensitive to previous October–November temperatures at high altitude but to current February and April precipitation at low altitude, whereas male trees were sensitive to current June temperature at high altitude but to January precipitation at low altitude. Our findings provide direct evidence that the response of tree-ring growth to climate is sex dependent, and could be changed with altitude elevation.
FUNDING
This research was supported by the National Natural Science Foundation of China (31170389 and 31370596) and the Innovative Team Foundation of the Sichuan Provincial Department of Education (14TD0015).
ACKNOWLEDGEMENTS
We thank Mr. Xiaofeng Li, Mr. Chengming Li, Dr. Hongyan Qiu, Dr. Jianping Duan, Dr. Lixin Lv, Dr. Pei Xing and Dr. Hanxue Liang for their help with both field and laboratory work. Thanks also to the administrators of Xiaowutai Mountain Nature Reserve for their support and to the Institute of Botany of the Chinese Academy of Sciences for supplying the Lintab 5 measuring system.
Conflict of interest statement. None declared.