-
PDF
- Split View
-
Views
-
Cite
Cite
M Noelia Barrios-Garcia, Mariano A Rodriguez-Cabal, Jennifer A Rudgers, Gregory M Crutsinger, Soil fertilization does not alter plant architectural effects on arthropod communities, Journal of Plant Ecology, Volume 10, Issue 5, October 2017, Pages 800–807, https://doi.org/10.1093/jpe/rtw087
- Share Icon Share
Abstract
While a growing number of studies have demonstrated the importance of intraspecific differences within plant species on associated arthropod communities, little is known regarding the relative strength of these effects compared to environmental factors. In this study, we examined whether intraspecific plant differences and nutrient fertilization interact to shape the arthropod community of a dominant coastal shrub, Baccharis pilularis (coyote bush).
We overlaid a fertilization treatment on a 12-year-old common garden experiment planted with erect and prostrate architectural morphs of Baccharis in California, USA. To collect the associated arthropod community, we vacuum sampled the crown of each Baccharis and identified individuals to species or morphospecies.
We found that arthropod richness and abundance were 2- to 3-fold greater on prostrate Baccharis than on erect morphs, but observed no main effects of fertilizer addition on the overall arthropod communities. Predators responded as strongly as herbivores to plant morph, and both were unaffected by nutrient additions. Only the specialist stem galler, Gnorimoschema baccharisella, showed an interactive response to plant morph and fertilization. Nitrogen, phosphorous and potassium addition had opposite effects on the two morphs, increasing stem gall abundance by 50% on prostrate morphs, but reducing galling by 20% on erect morphs. The architectural complexity of prostrate morphs could be the driving mechanism of differences in arthropod assemblages. Overall, our results demonstrate that community-level consequences of intraspecific differences in plants are strong, rather than being context dependent, and are generally maintained under different resource environments. The growing number of studies showing strong genotype than nutrient effects on associated arthropod communities suggests that this might be a generalized pattern.
INTRODUCTION
Over the past decade, empirical studies from a range of study systems have revealed an important role for intraspecific genetic variation in structuring plant-associated communities (Barbour et al. 2009; Crutsinger et al. 2014; Fritz 1990; Johnson and Agrawal 2005; Maddox and Root 1987; Schweitzer et al. 2008; Williams and Avakian 2015). While examples span diverse taxonomic groups (e.g. epiphytic and terrestrial plants, terrestrial invertebrates, foliar endophytes, mycorrhizal fungi and soil microbes), arthropods exhibit particularly strong responses to genetic differences among their host plants (Whitham et al. 2012). For example, host plant susceptibility or resistance traits can influence herbivore preference and performance, resulting in many-fold differences among plant individuals in the total herbivore abundance they support (Karban 1987; Maddox and Root 1987; Wimp et al. 2007). In turn, differences in the abundance of herbivorous prey can influence predation at higher trophic levels, including other arthropods (Johnson and Agrawal 2005; Schädler et al. 2010) and vertebrates (Bailey et al. 2004). Ultimately, differences among host plant genotypes can result in considerable shifts in overall arthropod diversity and composition (Johnson et al. 2006; Whitham et al. 2012; Wimp et al. 2005).
Although the ecological consequences of genetic differences can be considerable (Bolnick et al. 2011), there are numerous other drivers of trait variation within plant species that have the potential to impact associated arthropods (Barbour et al. 2015). Because studies have tended to use common garden experiments that control for environmental differences (Maddox and Root 1987; Wimp et al. 2005), prior work has not compared the magnitude of intraspecific genotypic differences to the effects of abiotic drivers of trait variation. Consequently, there are significant gaps in current understanding of how genetically based trait variation interacts with environmental conditions (a G × E interaction) to shape plant-associated communities (Hersch-Green et al. 2011; Hughes et al. 2008).
Soil nutrient availability is a key aspect of the plant’s abiotic environment that can vary locally, influence plant quality and productivity (Pierik et al. 2011) and thereby potentially affect the composition of associated arthropods (Hurd and Wolf 1974; Kirchner 1977; Siemann 1998; Strauss 1987). For example, Orians and Fritz (1996) found that different genotypes of willows (Salix sericea) responded uniquely to nitrogen, phosphorous and potassium (NPK) fertilization to differentially affect the abundance of several herbivores, including leaf-folding sawflies. Far less is known about the importance of G × E interactions at the community level, as most studies have focused on pairwise interactions between host plants and individual herbivore species (Kittelson 2004; Osier and Lindroth 2001; Stiling and Bowdish 2000; Strauss 1990), rather than including the entire arthropod community (but see, Johnson and Agrawal 2005; Tack et al. 2010). Prior evidence suggests that the availability of nutrients can trump the effects of intraspecific differences within host plant species (Crutsinger et al. 2013; Johnson et al. 2008). However, in contrary examples, the effects of intraspecific differences remained strong across variable nutrient conditions (Burkle et al. 2013; Mutikainen et al. 2000; Orians and Fritz 1996; Osier and Lindroth 2001; Tomas et al. 2011). The lack of resolution from current data combined with the small total number of studies that test for interactions at the community level (N = 7) suggests that additional studies that expand the range of environments examined are needed to draw more general conclusions.
We examined intraspecific differences within Baccharis pilularis De Candolle (Asteraceae, coyote brush), a dominant shrub that occurs throughout California, USA. In the coastal dunes of California, B. pilularis grows as two distinct architectural forms: an erect morph (1- to 4-m tall, subspecies B. p. consanguinea) and a prostrate morph (0.1- to 0.2-m tall, formerly B. p. pilularis, Flora of North America Editorial Committee 1993+). The two architectural morphs of B. pilularis (Baccharis hereafter) co-occur and cover similar proportions of the dunes at our study site. The two morphs also differ in a variety of traits (leaf size, number of branches and branch length) and are known to vary in the composition of their foliar arthropods (Crutsinger et al. 2014; Rudgers and Whitney 2006), including dominant, specialist herbivore species, such as stem gallers (Gnorimoschema baccharisella) and leaf gallers (Rhopalomyia californica) (Rudgers and Whitney 2006). Architectural variation in Baccharis has a genetic basis (Rudgers and Whitney 2006; Thompson et al. 1995); however, since the genetic mechanisms of trait differences have not yet been described, we hereafter refer to the two forms as erect and prostrate ‘morphotypes’ and trait variations as ‘intraspecific differences’. Baccharis is a dominant species in coastal dunes, characterized by sandy, fast-draining and nutrient-poor soils (Barbour 1973; Maun 2009). Based on these features of the system, we expected nutrient availability to be an important abiotic factor affecting host plant quality and plant-associated arthropods. For example, studies in other systems have shown that fertilization benefits herbivorous insects by increasing the nutritional quality of the host plant (Coley et al. 1985; Herms 2002; La Pierre and Smith 2016). However, it is unclear whether and how fertilization effects might cascade to predatory arthropods. While some studies found that herbivores tend to be more responsive to plant nutrition than predators (Hartvigsen et al. 1995), others have shown proportional responses of predator numbers to herbivore densities (Denno et al. 2002; Forkner and Hunter 2000; Gruner 2004). Moreover, changes in the predator:herbivore ratios due to fertilization on the different morphotypes would let us identify the relative importance of bottom-up or top-down forces in the trophic structure of Baccharis (Denno et al. 2002; Forkner and Hunter 2000).
In this study, we fertilized erect and prostrate Baccharis shrubs growing in a long-term common garden experiment to assess the interactive effects of intraspecific differences and soil nutrient availability on arthropod communities. We addressed the following questions: (i) How do intraspecific differences in Baccharis architectural morph and nutrient fertilization treatment influence the richness, abundance and composition of foliar arthropods? (ii) Do herbivores and predators respond differently to plant architectural morph and nutrient fertilization? (iii) Are the community-level consequences of architectural differences maintained with nutrient fertilization?
METHODS
In 1998, Rudgers and Whitney (2006) established a common garden experiment at the University of California–Davis Bodega Marine Reserve (BMR) in Bodega Bay, CA, USA (38°19′N, 123°04′W). The common garden measured 45×60 m, originally consisting of 250 plants (125 erect, 125 prostrate) in 1 m2 plots spaced 3 m apart and arranged along fifteen 60-m transects. Plants were grown from cuttings of locally growing adult Baccharis plants of the two morphs from randomly chosen locations throughout the 147-ha BMR reserve. Cuttings were started in the greenhouse prior to planting the garden, and each individual was assigned at random to plot locations within the common garden (for further details on the common garden, see Rudgers and Whitney 2006). At the initiation of this study, 134 shrubs (74 prostrate, 60 erect) had survived with identifiable tags.
Nutrient additions
In 2010 and 2011, we applied one of three nutrient treatments on individuals of erect and prostrate morphs: NPK, carbon and control. Nutrient addition treatments consisted of (i) additions of a slow-release NPK fertilizer (14:14:14 Apex Nursery Fertilizer, JR Simplot Company, Lathrop, CA, USA) at 20g/m2 (or ~3 µg N, P, K per g soil), (ii) additions of carbon (C) applied as sucrose at a rate of 150g C/m2 beginning in March and repeated every 60 days or (iii) an unamended control. Application of sucrose, which is 46% C in a molecular form readily available to microbes, results in immobilization of plant available N in the soil solution. Addition rates were consistent with other studies investigating controls of N and C on plant population and community dynamics (McLendon and Redente 1992; Sanders et al. 2007). NPK additions were reapplied in 2011, but we ceased sucrose additions at the end of the first year due to logistical constraints (sucrose must be regularly applied) and to focus on primarily on NPK fertilization effects. Preliminary soil analysis showed no initial differences in soil nutrient availability between erect and prostrate morphs (Crutsinger et al. 2013). We used 69 shrubs total, including 28 controls (14 prostrate, 14 erect), 21 NPK additions (12 prostrate, 9 erect) and 20 sucrose additions (10 prostrate, 10 erect). The nutrient treatments were effective at reducing nutrient limitation, and the sample size was appropriate to detect differences, as evidenced on a previous study showing an increase in cover and biomass of the dominant understory plant species with NPK addition (Crutsinger et al. 2013).
Arthropod community sampling
The arthropod community was sampled once a year (2010 and 2011) in the middle of the growing season, when the community diversity is at its peak (Keith et al. 2010). We vacuum sampled the entire crown of each Baccharis shrub using a modified leaf blower/vacuum (Craftsman 25cc 2-cycle, Illinois, USA) with a fine insect net attached. We also visually surveyed each plant for sedentary arthropods, including leaf and stem galls. Vacuum samples were transferred to plastic storage bags and stored on ice in a cooler. In the laboratory, we sorted arthropods and identified them to species, family or morphospecies and assigned them to a trophic level using reference literature and feeding morphology. Here, we focused on the responses of herbivores and predators, as they were the most abundant trophic levels in our samples. Omnivores were included in the total richness and composition analyses, but were not examined for individual trophic responses because they made up a smaller proportion of the community.
Statistical analyses
To examine arthropod community responses, we used separate repeated-measures analysis of variance (ANOVA) with the fixed effects of architectural morph (erect vs prostrate) and nutrient treatment. To control for differences in number of individuals among plants, we calculated individual-based rarefaction in PRIMER V.6 (Clarke and Gorley 2006), using the sample with the fewest numbers of individuals for the whole community. We did not calculate herbivore and predator richness rarefied because we found no effect on total rarefied richness. The community-level response variables were as follows: total abundance and richness, rarefied total richness, Shannon diversity, herbivore abundance and richness, predator abundance and richness, predator:herbivore abundance and richness, and stem and leaf gall abundance. The response variables, total abundance, herbivore abundance, predator abundance and predator:herbivore abundance, were log10 (X + 1) transformed to meet normality and reduce heteroscedasticity of variances. When data transformation did not meet the assumptions of normality (rarefied total richness, Shannon diversity, predator richness and the abundance of stem and leaf galls), we used generalized linear models with a Poisson distribution and log-link function on the 2011 data for integer values, and quasi-Poisson for non-integer values. For clarity, we show the untransformed values in all figures. All the analyses were performed in JMP Pro 10 statistical software (SAS Institute, Pacific Grove, CA, USA, 2001) and RStudio version 0.98.1062 (RStudio Team, Boston, MA, USA, 2009). Next, we determined how arthropod community composition varied among the genotypes and nutrient treatments, using permutational multivariate ANOVA (Anderson 2001) with the fixed effects of architectural morph, nutrient treatment and year. We used a Bray–Curtis dissimilarity matrix on log-transformed raw abundance data of each arthropod species and ran 9999 permutations in PRIMER v6 (Clarke and Gorley 2006). The results were qualitatively the same when we used proportional data. To visualize the results, we performed ordinations using non-metric multidimensional scaling in PRIMER v6 using Bray–Curtis similarity (Clarke and Gorley 2006).
RESULTS
In total, we identified 80 species and collected 2281 individuals in 2010, of which 47% were characterized as predators and 42% as herbivores, and 3821 individuals in 2011, of which 33% were characterized as predators and 51% as herbivores. On average, there were four species of herbivores and five species of predators per sample. The most common herbivores were a leafhopper (Cicadellidae), a psyllid (Psyllidae), and a gall midge (Cecidomyiidae, together representing ~18.5% of all the individuals), while the most abundant predators were three parasitoid wasps (representing 12% of all the individuals).
We found that erect and prostrate architectural morphs of Baccharis differed considerably in the associated arthropod community (Fig. 1). Specifically, 3-fold more individuals (F1,60 = 32.24, P < 0.001) and 2-fold more species (F1,60 = 29.07, P < 0.001) were associated with prostrate morphs than with erect morphs of the same plant age (Fig. 1). Shannon diversity was 1.3-fold greater on prostrate morphs than on erect plants ( = −3.42, P = 0.001). Within trophic levels, herbivore abundance was 3-fold higher on prostrate than erect morphs (F1,60 = 32.66, P < 0.001), while herbivore richness was 1.4-fold higher (F1,60 = 18.57, P < 0.001, Fig. 1). Similarly, predator richness ( = 46.29, P < 0.001) and abundance (F1,60 = 28.84, P < 0.001) were 2- and 3-fold higher, respectively, on prostrate morphs (Fig. 1). Predator:herbivore abundance ratio remained similar for both morphs (F1,50 = 3.27, P = 0.076, Fig. 1), while predator:herbivore richness ratio was 1.2-fold greater in prostrate than on erect morphs (F1,54 = 11.32, P = 0.001). When looking at the dominant galling herbivores, we found that the abundance of R. californica leaf galls was twice as great on prostrate than erect morphs ( = 5.50, P = 0.019, Fig. 2). There was no difference in total rarefied richness among morphs, suggesting that diversity responses were driven by the overall higher abundances of arthropods on prostrate morphs (supplementary Table S1).
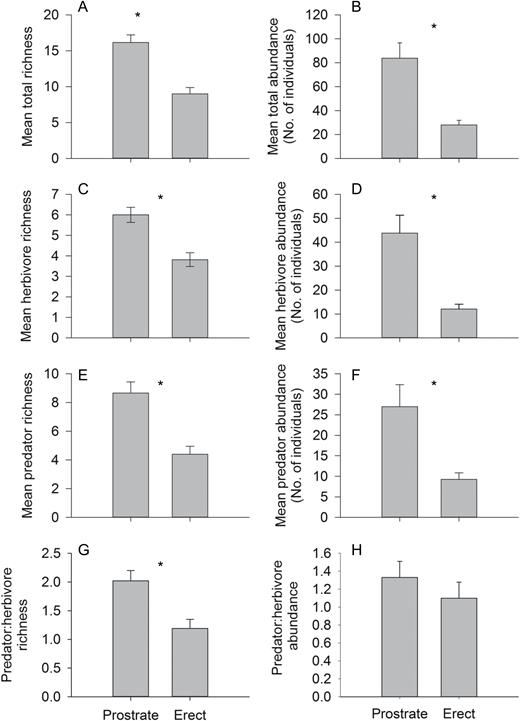
arthropod community responses to Baccharis pilularis architectural morphs (erect vs prostrate): (A) total richness, (B) total abundance, (C) herbivore richness, (D) herbivore abundance, (E) predator richness, (F) predator abundance, (G) predator:herbivore richness and (H) predator:herbivore abundance. Bars represent means ± SE, asterisks denote statistically significant differences (P < 0.05).
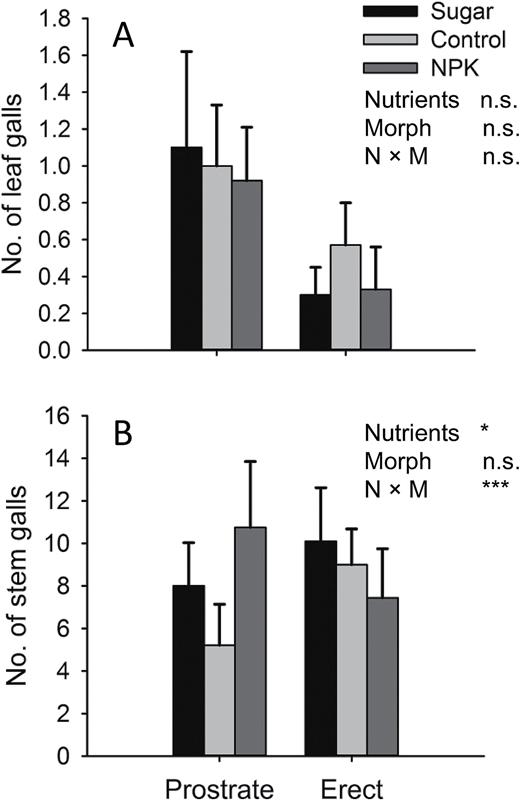
relationship between the number of Rhopalomyia californica leaf galls (A) and Gnorimoschema baccharisella stem galls (B) associated with Baccharis pilularis architectural morphs (erect vs prostrate) and nutrient treatments (sugar, control and NPK). Bars represent means ± SE, asterisks denote statistically significant differences (P < 0.05).
In contrast to their large response to architectural morph, we observed no response of any aspects of the arthropod community we measured to nutrient fertilization, nor were there any interactions between morphs and nutrients, with the exception of the dominant stem-galling moth, Gnorimoschema (supplementary Table S1 and Figure S1; Fig. 2). Overall, we found 1.3-fold greater abundance of stem galls on sugar and NPK treatments than in controls ( = 9.06, P = 0.0108), but importantly, there was a significant interaction between morph and nutrients ( = 20.10, P < 0.0001). Specifically, NPK addition had opposite effects on the two morphs, increasing 50% on prostrate morphs, but reducing galling by 20% on erect morphs relative to unfertilized controls (Fig. 2).
In addition to the differences in arthropod richness and abundance, overall arthropod composition varied with erect and prostrate morphs (pseudo-F = 8.80, P = 0.0001), but not with the nutrient treatments (pseudo-F = 0.99, P = 0.4680) or interaction (pseudo-F = 1.05, P = 0.3842), having an average Bray–Curtis dissimilarity of 74.8% between architectural morphs and 74.08% between years (Fig. 3).
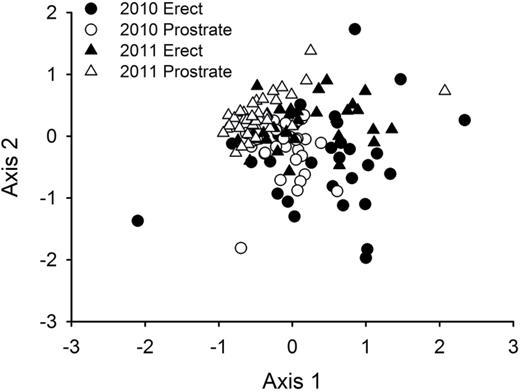
a non-metric multidimensional scaling ordination of community composition based on Bray–Curtis dissimilarity showing differences due to architectural morphs (erect vs prostrate) and sampling year (2010 and 2011). There were no significant effects of nutrient addition or interactions with nutrient addition.
DISCUSSION
Our results suggest that intraspecific differences in a dominant plant is more important than plant nutrient fertilization in structuring an arthropod assemblage in the coastal dunes of California. While our common garden experiment showed consistently strong effects of erect versus prostrate Baccharis morphotypes on arthropod communities, there were minimal effects of nutrient fertilization during this 2-year study. Prostrate shrubs supported 2- to 3-fold more arthropod species and individuals, and these effects were consistent across herbivore and predator trophic levels. From prior work in this experiment, Crutsinger et al. (2014) showed that architectural morphs of Baccharis supported unique assemblages of aboveground community of foliage arthropods and litter microarthropods. A suite of plant traits (leaf size, number of branches and branch length) and abiotic conditions, as part of the general phenotypic differences between the morphs, likely account for these arthropod responses. For example, prostrate shrubs provide a greater biomass, a deeper litter layer and a more humid environment (Crutsinger et al. 2010, 2014; Rudgers and Whitney 2006). These differences in the abiotic microenvironment combined with the greater architectural complexity of prostrate morphs may be the driving mechanism of differences in arthropod assemblages (Langellotto and Denno 2004).
Our study also provides further evidence for community specificity to intraspecific differences among plants (reviewed by Whitham et al. 2012). For example, one of the earliest studies to find that genetic differences scale to the community level was Maddox and Root (1987) who reported that 18 clones of goldenrod (Solidago altissima) differed in resistance to 15 herbivorous insects. Since then, a growing number of studies using trees, shrubs, forbs and grasses have all observed that plant genotype shapes the plant-associated arthropod community (Faeth and Shochat 2010; Johnson and Agrawal 2005; Rudgers and Whitney 2006; Wimp et al. 2005). The differences among plant genotypes in arthropod diversity can rival those among plant species (Crutsinger et al. 2006). Our results thus strengthen the evidence in support of the importance of intraspecific genetic variation in structuring plant-associated communities.
Interestingly, we observed minimal effects of nutrient fertilization on arthropods. In a past study, we showed that the cover and biomass of the dominant understory plant species increased with NPK addition to Baccharis shrubs, indicating that our nutrient treatments were effective at reducing nutrient limitation in the dunes (Crutsinger et al. 2013). Baccharis shrubs grow slowly, and we did not detect growth rate differences (crown circumference did not vary between treatments or years, P > 0.05). Yet, we found that the abundance of a dominant herbivore in this system, Gnorimoschema, responded to both Baccharis morphotype and fertilization interactively, increasing with NPK addition on prostrate morphs but decreasing with fertilization on erect morphs (Fig. 2). The branching architecture, number of buds per branch length or plant secondary chemistry could be underlying drivers of the alternative responses of different morphs, suggesting different resource dynamics or defensive chemistry between the two morphs (Larson and Whitham 1991). Galling insects are typically attracted to vigorously growing, high-nutrient plants (Moran and Goolsby 2014), which would support the positive correlation on prostrate morphs. In addition, fertilizer additions may have increased the number of buds inside the plant canopy and hence the number of opportunities for galls to form. The decrease with fertilization on erect morphs is more puzzling to explain. One hypothesis is that nutrient additions elevate defensive secondary chemistry in the erect morphs, which naturally receive higher loads of the stem galls than prostrate plants (Rudgers and Whitney 2006). Thus, a decline in gall abundance with fertilization could occur if plants shunt added nutrients toward increased production of defensive compounds. However, we currently lack information on differences in secondary chemistry between the morphs that would allow a test of this hypothesis.
To date, there has been little research on the interactive effects of intraspecific differences and other drivers at the whole-community level. Previous work has primarily explored genotype and fertilization effects on insect performance rather than abundance or composition (Mutikainen et al. 2000; Osier and Lindroth 2001; Tomas et al. 2011). For example, Osier and Lindroth (2001) found that gypsy moth (Lymantria dispar) larvae grew faster feeding on foliage under fertilizer addition, and this effect depended on plant genotype. Orians and Fritz (1996) found that herbivore abundances on S. sericea interacted with genotype and NPK fertilization treatments. Specifically, they found that several herbivores were more abundant on fertilized plants, one was less abundant, and the abundance of others did not change. Fertilization decreases woody plant resistance to insects by enhancing the nutritional quality of the plant and/or decreasing secondary metabolite concentrations (Herms 2002). However, genotypes may differ in their rates of nitrogen incorporation; thus, nutrient addition may trigger genotype-specific responses (Tomas et al. 2011) in which some genotypes are more resistant in one environment, but more susceptible in another environment (Orians and Fritz 1996).
One of the goals of this study was to assess whether herbivores and predators respond differently to morphotype or soil nutrient fertilization. Predators responded as strongly as herbivores to plant morph, and both were unaffected by nutrient additions. While, to our knowledge, other studies have not explored the interactive effects of genotype and fertilization at different trophic levels, our results complement previous community genetics studies, in which plant genotypes have been shown to influence the abundance and richness of higher trophic levels. This result indicates that genotypic effects of plant host on herbivorous arthropods can cascade through the food web (Dickson and Whitham 1996; Johnson 2008; Wimp et al. 2005). Larger and complex habitat in prostrate shrubs provides a range of favorable conditions (e.g. microhabitat, food resources) than those in erect shrubs thus supporting more foliar arthropods (Crutsinger et al. 2014), which in turn attracts natural enemies and explains the greater predator abundance, richness and predator:herbivore richness (Langellotto and Denno 2004).
Similarly, at the community level, arthropod composition varied with genotype and time, but not with nutrients. The large variation in arthropod composition (74%) among morphs was maintained through time and also much stronger than temporal variability within a morph. This result further highlights the prevailing role of genetic differences on associated communities (Whitham et al. 2012). Moreover, there was larger temporal variability in arthropod community composition on erect plants compared to prostrate plants. We hypothesize that erect morphs are less protected from winds and dispersal immigration/emigration than are prostrate morphs; this difference in exposure could make them more sensitive to interannual variability in the environment, and therefore more variable in arthropod species composition.
Taken together, our results demonstrated that intraspecific plant differences in Baccharis prevailed over nutrient fertilization in structuring a plant-associated arthropod community. While some studies have shown that environmental variations such as the location of common garden experiments may swamp the effect of host plant genotype (Kittelson 2004; Stiling and Rossi 1995; Tack et al. 2010; Tack and Roslin 2011), our results confirm a strong role of intraspecific differences in structuring dependent communities (Whitham et al. 2012). The architectural traits of prostrate morphs may be the driving mechanism of differences in arthropod assemblages in Baccharis. The growing number of studies showing strong genotype than nutrient effects on associated arthropod communities suggests that this might be a generalized pattern (Burkle et al. 2013; Mutikainen et al. 2000; Orians and Fritz 1996; Osier and Lindroth 2001; Tomas et al. 2011). A comprehensive understanding of the role of G × E interactions will require careful, detailed study in a range of different systems.
SUPPLEMENTARY MATERIAL
Supplementary material is available at Journal of Plant Ecology online.
FUNDING
EEB summer grants from the Department of Ecology and Evolutionary Biology, University of Tennessee (to M.N.B.-G. and M.A.R.-C.); the Miller Institute for Basic Research in Science (to G.M.C.).
ACKNOWLEDGEMENTS
We thank J. Sones at BMR for research assistance. We gratefully acknowledge two anonymous reviewers who provided constructive comments on a previous version of this manuscript.
Conflict of interest statement. None declared.
REFERENCES