-
PDF
- Split View
-
Views
-
Cite
Cite
Martin Duchoslav, Martina Fialová, Michaela Jandová, The ecological performance of tetra-, penta- and hexaploid geophyte Allium oleraceum in reciprocal transplant experiment may explain the occurrence of multiple-cytotype populations, Journal of Plant Ecology, Volume 10, Issue 3, 1 June 2017, Pages 569–580, https://doi.org/10.1093/jpe/rtw039
- Share Icon Share
Abstract
Many polyploids are geographically separated from their lower-ploidy progenitors at a variety of spatial scales. Diverse adaptive and non-adaptive mechanisms have been suggested to contribute to these spatial patterns. A long-standing hypothesis holds that polyploids are established and persist due to specialization to different ecological conditions compared to their progenitors. However, experimental approaches testing this hypothesis remain rare. Empirical evidence for niche differences among ploidy levels has been found previously for the European polyploid geophyte Allium oleraceum. Here, we conducted a 5-year reciprocal transplant experiment with tetraploid, pentaploid and hexaploid A. oleraceum to test for adaptation at the ploidy and population levels.
In 2006, we sowed 2160 aerial bulbils among six sites (two per cytotype, central and western Moravia, the Czech Republic) and monitored their emergence in 2007, their survival until 2011 and their performance in 2011.
The emergence of bulbils was high at all target sites, and no evidence for niche differentiation among cytotypes was recorded in the first year. Survival in the following years was relatively high, but significantly better survival for plants of home ploidy than foreign ploidy was recorded. No plant reached the reproductive stage during our experiment. Adopting a strict definition of local adaptation, local plants only rarely performed better than foreign plants at both sites in pairwise comparisons within a cytotype. Together, our results suggest weak niche differentiation among cytotypes and provide limited support for the existence of local adaptation within cytotypes. Thus, all cytotypes are able to establish in habitats typically populated by other cytotypes via aerial bulbil spread, but will likely die out gradually in non-native habitats. This ability may explain the occurrence of some multiple-cytotype populations. The complex spatial pattern of A. oleraceum cytotypes is therefore the result of several interacting factors, including niche differentiation among cytotypes, the different evolutionary ages of cytotypes, the existence of gene flow between and within cytotypes, the dominance of clonality and stochastic events linked to anthropogenic dispersal and disturbance.
INTRODUCTION
Polyploidy is an important force driving the evolution and diversification of many plant lineages (Grant 1981; Lewis 1980; Soltis et al. 2014; Wendel 2000). It manifests in different characteristics at the ecological level, including the pattern of cytotype distribution (Soltis et al. 2010). Data reviewed by Grant (1981) shows that many polyploids are geographically separated from their diploid progenitors. Under what circumstances could such spatial separation between cytotypes originate? Polyploids must originate within populations of lower-ploidy progenitors (i.e. through sympatry), and in order to persist and form a viable population, must avoid reproductive disadvantages caused by a greater proportion of ineffectual pollinations from dominant lower-ploidy progenitors (Levin 1975). Polyploidization has profound consequences for gene expression (Adams and Wendel 2005) and the physiological and ecological behaviour of plants (Levin 2002; Lumaret 1988; Ramsey 2011; Soltis et al. 2003). Polyploids might be preadapted to a new environment and thus could escape competition with their parents. Alternatively, increased genetic buffering provided by possessing extra copies of the genome as well as changes in gene expression may affect the potential of polyploids for novel adaptation in response to selection (Bretagnole and Thompson 1996; Levin 2002; Otto and Whitton 2000; Soltis and Soltis 1999; Soltis et al. 2003; Wendel 2000). Numerous studies have demonstrated that polyploids have a broader niche and/or may differ in their niche optima from their diploid conspecifics (Ramsey and Ramsey 2014; Soltis and Soltis 1995, 2000; Thompson and Lumaret 1992). Hence, the spatial segregation of cytotypes at both the local and global scale might be the consequence of niche differentiation (Levin 2002).
At the local scale, polyploid cytotypes that are dispersed into a new environment may respond to natural selection to become locally adapted (Buggs and Pannell 2007) under conditions of local environmental stability, sufficient time for selection and limited gene flow (Slatkin 1987). Because it is common in natural populations for polyploids to have multiple origins (Soltis and Soltis 1993, 1999), recurrent formations would introduce genetic variation into the polyploid population and could influence ecological attributes (Soltis et al. 2010). The ability of plants to adapt at a local scale to physical stressors in the environment has been described (Linhart and Grant 1996) but remains controversial. A meta-analysis of 35 reciprocal transplant studies showed that fewer than half provided evidence for local adaptation (Leimu and Fischer 2008).
However, spatial segregation of cytotypes on a global and/or local scale may be explained by the involvement of several alternative mechanisms, even without differences in niche (i.e. due to non-adaptive processes). Spatial segregation of cytotypes may simply reflect the position of past cytotype refuges relative to the sites available for colonization by single cytotypes (Godsoe et al. 2013; Mandáková and Münzbergová 2006; van Dijk et al. 1992). Alternatively, spatial segregation on a local scale may be maintained by limited dispersal (Baack 2005) or by frequency-dependent mating (Felber 1991; Rodriguez 1996).
The importance of niche differentiation to the spatial differentiation of cytotypes has been determined by the analysis of local environmental conditions at the sites occupied by each cytotype (e.g. Johnson et al. 2003; Lumaret et al. 1987; Sonnleitner et al. 2010) or from correlations between climatic data and cytotype occurrence (Chung et al. 2015; Glennon et al. 2014; Laport et al. 2013; McIntyre 2012; Thompson et al. 2014). However, such approaches may confound niche differentiation by competitive displacement (Baack and Stanton 2005) and cannot replace the knowledge obtained through experimentation (Glennon et al. 2014). Indeed, a recently published analysis of large data sets collected in 20 polyploid complexes did not find evidence for climatic niche shifts between conspecific ploidy levels. Instead, the results indicated that alternative explanations, including competitive exclusion, dispersal capabilities, drift or local selection could explain polyploid persistence better (Glennon et al. 2014).
Surprisingly, studies adopting experimental approaches comparing the performance of cytotypes in a common garden or using reciprocal transplants to directly test whether ecological differentiation exists between cytotypes are rare. Common garden experiments are inferior to reciprocal transplant experiments because they minimize environmental variation or include a specific environmental treatment. Hence they cannot incorporate all of the relevant conditions of the native habitats of the compared cytotypes (Baack and Stanton 2005). Until now, only six studies have investigated the ecological performance of cytotypes using reciprocal transplantation (Baack and Stanton 2005; Buggs and Pannell 2007; Flegrová and Krahulec 1999; Martin and Husband 2013; Raabová et al. 2008; Ramsey 2011). These experiments primarily compared higher ploidy levels (2n = 4× or 6×) with the diploid cytotype, and resulted in various findings spanning from evidence for local adaptation through the superiority of one cytotype to a lack of difference in adaptation between cytotypes. Flegrová and Krahulec (1999) performed reciprocal transplantations between a population of diploid Anthoxanthum alpinum occupying higher altitudes and a population of tetraploid Anthoxanthum odoratum occupying lower altitudes. They reported a higher fitness for A. odoratum in both environments and provided little clear evidence for differential local adaptation. Baack and Stanton (2005) did not observe ecological differentiation between cytotypes or local adaptation within cytotypes of Ranunculus adoneus, despite strong allopatry between diploids and tetraploids. Buggs and Pannell (2007) detected ecological differences between diploid and hexaploid Mercurialis annua but did not observe local adaptation; rather, the diploids had a higher fitness than the polyploids across both diploid- and polyploid-occupied regions. Raabová et al. (2008) reported weak niche differentiation between and local adaptation within diploid and hexaploid cytotypes of Aster amellus that may contribute to the maintenance of uniform cytotype populations within the contact zone of the cytotypes. In Achillea borealis, Ramsey (2011) recorded a greater fitness advantage of newly generated hexaploids over tetraploids in parental tetraploid habitats, achieved via genome duplication. However, reciprocal fitness was not examined. Martin and Husband (2013) found adaptation of diploid and tetraploid Chamerion angustifolium to their native elevation but not to local plots within the same elevation. It is clear that more data are necessary to address the relative importance of adaptive versus non-adaptive processes facilitating polyploid evolution (Ramsey and Ramsey 2014; Soltis et al. 2010; Weiss-Schneeweiss et al. 2013).
Allium oleraceum L. (Amaryllidaceae) is a polyploid geophyte with common asexual (aerial bulbils, daughter bulbs) but rare sexual reproduction (Fialová and Duchoslav 2014; Fialová et al. 2014), represented by three common cytotypes (2n = 4×, 5×, 6×) in Central Europe that frequently forms mixed cytotype populations (Šafářová and Duchoslav 2010; Šafářová et al. 2011). Duchoslav and Staňková (2015) found that the studied cytotypes showed low within-population genetic variation, whereas genetic differentiation was high among populations. In a detailed cytogeographical study, Duchoslav et al. (2010) found a complex distribution pattern of the aforementioned cytotypes, with pentaploids most frequently occurring in sympatry with other cytotypes. The distribution of the less frequent tetra- and hexaploids was sympatric in the eastern part of the Czech Republic, but parapatric in western regions. All cytotypes inhabited a wide and overlapping spectrum of habitats but were ecologically differentiated (i.e. the ruderal character of cytotypes increased from tetra- to hexaploids). Duchoslav et al. (2010) have provided evidence for niche differences among ploidy levels. However, this explanation is based on the description of patterns and must be experimentally tested (Soltis et al. 2010). The experimental approach is particularly important in situations when at least 25% of the analyzed A. oleraceum populations possess multiple cytotypes (Duchoslav et al. 2010) and when cytotypes co-occur with or without significant spatial segregation within multiple-cytotype sites found in both heterogeneous and homogeneous environments (Šafářová and Duchoslav 2010). Such patterns suggest that niche differentiation alone is probably insufficient to limit the co-occurrence of A. oleraceum cytotypes and also question the existence of local adaptation within cytotypes due to the observed low within-population genetic diversity and dominance of asexuality.
Here, we carried out a complete reciprocal transplant experiment in the field using aerial bulbils of three A. oleraceum cytotypes (tetra-, penta- and hexaploids) transplanted among six sites (two per cytotype). We asked the following questions: (i) Is there any evidence of superior performance of plants of local ploidy level compared to foreign ploidy plants in a local ploidy level habitat (i.e. niche differentiation among the cytotypes)? (ii) Do plants at their home site outperform plants originating from a foreign (= non-native) site of the same ploidy level, suggesting the presence of local adaptation?
MATERIALS AND METHODS
Study sites, plant material and experimental design
A complete reciprocal transplant experiment was carried out in the Moravia region of the Czech Republic at plots within six sites that harboured existing populations of A. oleraceum (tetraploids: Ludmírov, Slatinice; pentaploids: Bošovice, Smrk; hexaploids: Lísky, Potštát; see online supplementary Table S1). We selected study sites from the stock of known localities (Duchoslav et al. 2010) as follows: (i) habitat conditions of site were typical of the cytotype requirements according to Duchoslav et al. (2010), and (ii) the native population contained >500 reproductive individuals—a sufficient number of available fruiting individuals at the site for the collection of propagules. Habitat conditions of the sites where fruiting plants freely occur were considered optimal for each cytotype.
All A. oleraceum cytotypes produce viable seeds, but their numbers are low (Fialová et al. 2014). We therefore used aerial bulbils rather than seeds for this experiment. Mature aerial bulbils were collected from 40 randomly selected individuals (from 10 to 30 bulbils per plant) at each of the six sites in August 2006. Bulbils originating from individual plants sampled within the site were mixed together before sowing. In late September 2006 just after natural bulbil dispersal, bulbils from each sampled population were sowed into each site (i.e. we used all six sites where bulbils were collected for the transplant experiment) in three 90×90cm plots (= blocks) consisting of nine grid cells of 30×30cm. Each plot was created within the area of the population at each site in patches without the presence of native Allium plants to reduce possible mixing of home and alien bulbils. The transplant plots did not differ in vegetation composition from the surrounding habitat. Before sowing, the litter cover within each plot was slightly disturbed to ensure contact of sowed bulbils with soil. In each plot, we placed 20 bulbils from each population on the ground in the centres of randomly selected cells. Bulbils were placed slightly apart to decrease possible competitive interactions. This resulted in the sowing of 2160 bulbils (6 target sites × 3 plots × 6 populations of origin × 20 bulbils). The three remaining cells within each plot served as controls for natural propagule rain. We did not record any germinated propagules within the control cells during the course of the experiment.
In early May 2007, we returned to each plot and recorded the number of emerged juveniles as well as their positions within each cell. From 2008 to 2011, we visited each site twice a year. During early May, we recorded the number of surviving marked plants within each cell, their height and the number of leaves and number of underground daughter bulbs produced by the marked plant. Plants were checked for flowering during the second half of July. At the end of the experimental period (July 2011), we dug up all surviving plants and retested their ploidy by estimating the relative amount of DNA via flow cytometric analysis (see Duchoslav et al. 2010 for methodology) to determine whether the results were influenced by the admixture of bulbils from the local population. Retesting verified that no admixture of alien cytotypes into the experimental plots occurred.
In this paper, we present data for the first (2007) and the last year (2011) of the experiment. Unfortunately, during the first 2 years, one of three plots at each site was partly or completely destroyed by human activity (ploughing, log stacks by the roadside, afforestation) or by animal (pigs, voles) digging. Therefore, only two plots per site were available for the analysis of survival and performance.
The composition of vegetation at each site was recorded in order to characterize the habitats. We recorded vegetation relevés using the Braun-Blanquet (1964) scale at the 2×2 m area around the each plot at each site. For each relevé, a mean Ellenberg indicator value (Ellenberg et al. 1992) for several environmental factors (i.e. nutrients, light, temperature, moisture and soil reaction) was calculated without species weighting. Ellenberg indicator values are frequently used by European vegetation scientists as surrogates for measured environmental variables and reflect the ecological behaviour of species (Schaffers and Sýkora 2000).
Data analysis
Vegetation composition of the sites was analyzed by Detrended correspondence analysis (DCA), with cover data transformed by log transformation. Differences in vegetation composition among groups of relevés recorded at the sites were tested by Canonical correspondence analysis (CCA) using a Monte Carlo permutation test with 999 permutations and a split-plot design (sites freely exchangeable; relevés within each site were not permuted). Analyses were performed in CANOCO 4.5 package software (ter Braak and Šmilauer 2002).
To analyze plant survival and performance in the transplant experiment, we used linear or generalized linear mixed models ((G)LMMs; Bolker et al. 2009) using the Laplace approximation with an identity link function and normal distribution function for plant height and number of leaves, a log link function and Poisson distribution function for the number of daughter bulbs and a logit link function and binomial distribution function for emergence and survival. To distinguish between experimental sites and sources of propagules, we adopted the terminology used by Kawecki and Ebert (2004): ‘target’ identifies where the bulbils were sowed and ‘origin’ identifies where the bulbils were from; and ‘local’ versus ‘foreign’ indicates whether the target is the same versus is not the same as its origin, respectively. The transplanted bulbils were of three ploidy levels (tetraploid, pentaploid, hexaploid; ploidy level of origin) sampled within two populations per ploidy (population of origin). Within each target ploidy level habitat, there were two target sites and two blocks (= plots) at each target site. For each test, we adopted a specific structure of random factors using nested design. The block (= plot) was nested within the target site nested within the target ploidy level habitat and the population of origin was nested within the ploidy level of origin. In particular, we constructed two separate (G)LMMs for each fitness surrogate so as to investigate (i) ‘ploidy-level’ adaptation, and (ii) ‘population-level’ local adaptation using two local versus foreign contrasts: local ploidy level and local population. A positive effect of local ploidy level indicates niche differentiation between cytotypes (i.e. in its own habitat, the local ploidy is expected to show higher fitness than foreign cytotypes from other habitats). A positive effect of the local population indicates local adaptation (i.e. at each site, the local [= native] population is expected to show higher fitness than the population of the same ploidy from the other site). These hypotheses were addressed using separate (G)LMMs in which the contrast of local ploidy/population versus foreign ploidy/population for each site was represented as fixed effect predictor. Random factors included plot nested within target site nested within target ploidy level habitat and population of origin nested within ploidy level of origin. Additionally, we adopted a stricter approach to local adaptation (sensu Kawecki and Ebert 2004) and determined whether local plants perform better than foreign plants at both transplant sites for each pair of sites within each ploidy level using separate (G)LMMs in which the effect of local population versus foreign population for each site was represented as a fixed effect predictor and plot was a random factor.
All calculations were performed using R 3.1.2 (R Core Team 2014). For (G)LMMs, the ‘lme4’ (Bates et al. 2016) package was used. To evaluate the significance of fixed and random effects, alternative models without the variable of interest were compared to the full model using likelihood ratio tests. If an alternative model had a significantly better fit, this model was subsequently compared against further reduced models (Zuur et al. 2009). Model validity was checked by visual examination of the residual plots. Parameter (i.e. mean, SE, 95% confidence interval [CI]) estimates were calculated using the ‘lsmeans’ package (Lenth and Hervé 2016). We also calculated marginal R2 (proportion of variance explained by the fixed effects only) and conditional R2 (proportion of variance explained by both the fixed and random factors) as a measure of goodness-of-fit of each model (Nakagawa and Schielzeth 2013) using the ‘MuMIn’ package (Bartoń 2016). Multiple comparison Tukey tests were performed using the ‘multcomp’ package with P-values adjusted with the single-step method (Hothorn et al. 2016).
RESULTS
Vegetation composition of sites
Groups of sites classified according to ploidy level and sites within ploidy significantly differed in vegetation composition (CCA; ploidy: F = 1.2, P = 0.002; sites within ploidy: F = 2.7, P = 0.008). Tetraploid sites tended to be shadier, moister and better supplied with nutrients (e.g. forest mantel) in contrast to pentaploid sites, which were drier and more open, with nutrient poor soils (e.g. dry grasslands), as indicated by Ellenberg indicator values (Fig. 1). Hexaploid sites differed from sites of other ploidies by more acidic soils and a higher proportion of ruderal plants indicating frequent disturbances (i.e. road verge, ruderalized border of dry grassland).
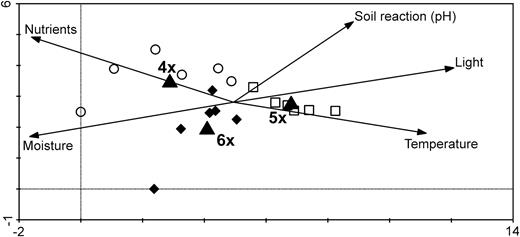
First two axes of DCA of vegetation relevés of the studied plots. Empty circles denote tetraploid sites, squares denote pentaploid sites and filled diamonds denote hexaploid sites. Centroids of respective target ploidy levels are denoted by filled triangles. Ellenberg indicator values are represented by vectors within the ordination diagram. The first two axes explain 25.2% of the total variation in species composition.
Bulbil emergence and plant survival
The establishment of juveniles from bulbils varied largely among cells (range 15–100%) and plots within sites (Table 1a) but generally reached high values (mean 66%). Pentaploids showed a better emergence rate compared to tetraploids in the target pentaploid habitat, while a worse performance of local cytotype or no differences among cytotypes were found in emergence rate in target tetraploid and hexaploid habitats, respectively (online supplementary Fig. S1). This resulted in a significant interaction of target ploidy level habitat × ploidy level of origin but non-significant contrast between local and foreign ploidy levels (Table 1a and b; Fig. 2a). A contrast between local and foreign populations was detected in the emergence rate (Table 1b; Fig. 2b). Separate analyses of each target site indicated a significantly higher emergence rate of the local population than of the foreign population of the same cytotype at four out of six sites; however, only at one pair of sites did the local plants display significantly better emergence than foreign plants (online supplementary Table S2a).
(a) chi-square values and significance from a (generalized) linear mixed models analysis for the emergence of juveniles in 2007, survival of plants from bulbils sowed in 2006 at the final survey in 2011, survival of adult plants emerged in 2007 at the final survey in 2011, height and number of leaves of plants at the final survey in 2011 and cumulative number of daughter bulbs produced by survived plants from 2007 to 2011 in the reciprocal transplant experiment with bulbils of Allium oleraceum; (b) local ploidy level contrast and local population contrast in emergence, survival and performance traits. χ2 and P-values are based on maximum likelihood ratio tests. Significant effects (at P ≤ 0.05) are in bold
Source of variation . | df . | Emergence (2006–07) . | Survival (2006–11) . | Survival of established plants (2007–11) . | Height (2011) . | Number of leaves (2011) . | Number of daughter bulbs (2011) . | ||||||
---|---|---|---|---|---|---|---|---|---|---|---|---|---|
(a) . | χ2 . | P . | χ2 . | P . | χ2 . | P . | χ2 . | P . | χ2 . | P . | χ2 . | P . | |
Target ploidy level habitat | 2 | 0.35 | 0.841 | 3.89 | 0.143 | 8.42 | 0.015 | 3.18 | 0.204 | 0.92 | 0.629 | 0.44 | 0.802 |
Target site | 1 | 1.21 | 0.271 | 0.01 | 0.920 | 0.02 | 0.887 | 0.48 | 0.489 | 5.01 | 0.025 | 4.03 | 0.045 |
Plot | 1 | 39.00 | <0.001 | 34.50 | <0.001 | 25.70 | <0.001 | 89.97 | <0.001 | 1.22 | 0.269 | 10.71 | 0.001 |
Ploidy level of origin | 2 | 1.06 | 0.588 | 0.57 | 0.754 | 0.43 | 0.806 | 2.93 | 0.231 | 2.15 | 0.341 | 37.74 | <0.001 |
Population of origin | 1 | 3.35 | 0.067 | 4.30 | 0.038 | 4.10 | 0.043 | 0.01 | 0.911 | 0.15 | 0.701 | 0.94 | 0.332 |
Target ploidy level habitat × Ploidy level of origin | 4 | 16.46 | 0.003 | 8.53 | 0.074 | 12.12 | 0.016 | 8.09 | 0.089 | 15.81 | 0.003 | 27.64 | <0.001 |
Marginal R2; conditional R2 | 0.04; 0.18 | 0.06; 0.14 | 0.12; 0.20 | 0.10;0.66 | 0.05; 0.27 | 0.15; 0.38 | |||||||
(b) | |||||||||||||
Local ploidy level | 1 | 0.05 | 0.827 | 4.38 | 0.037 | 6.54 | 0.011 | 1.92 | 0.166 | 1.58 | 0.209 | 6.97 | <0.001 |
Local population | 1 | 30.39 | <0.001 | 6.18 | 0.013 | 0.18 | 0.672 | 3.08 | 0.079 | 19.9 | <0.001 | 3.06 | 0.080 |
Source of variation . | df . | Emergence (2006–07) . | Survival (2006–11) . | Survival of established plants (2007–11) . | Height (2011) . | Number of leaves (2011) . | Number of daughter bulbs (2011) . | ||||||
---|---|---|---|---|---|---|---|---|---|---|---|---|---|
(a) . | χ2 . | P . | χ2 . | P . | χ2 . | P . | χ2 . | P . | χ2 . | P . | χ2 . | P . | |
Target ploidy level habitat | 2 | 0.35 | 0.841 | 3.89 | 0.143 | 8.42 | 0.015 | 3.18 | 0.204 | 0.92 | 0.629 | 0.44 | 0.802 |
Target site | 1 | 1.21 | 0.271 | 0.01 | 0.920 | 0.02 | 0.887 | 0.48 | 0.489 | 5.01 | 0.025 | 4.03 | 0.045 |
Plot | 1 | 39.00 | <0.001 | 34.50 | <0.001 | 25.70 | <0.001 | 89.97 | <0.001 | 1.22 | 0.269 | 10.71 | 0.001 |
Ploidy level of origin | 2 | 1.06 | 0.588 | 0.57 | 0.754 | 0.43 | 0.806 | 2.93 | 0.231 | 2.15 | 0.341 | 37.74 | <0.001 |
Population of origin | 1 | 3.35 | 0.067 | 4.30 | 0.038 | 4.10 | 0.043 | 0.01 | 0.911 | 0.15 | 0.701 | 0.94 | 0.332 |
Target ploidy level habitat × Ploidy level of origin | 4 | 16.46 | 0.003 | 8.53 | 0.074 | 12.12 | 0.016 | 8.09 | 0.089 | 15.81 | 0.003 | 27.64 | <0.001 |
Marginal R2; conditional R2 | 0.04; 0.18 | 0.06; 0.14 | 0.12; 0.20 | 0.10;0.66 | 0.05; 0.27 | 0.15; 0.38 | |||||||
(b) | |||||||||||||
Local ploidy level | 1 | 0.05 | 0.827 | 4.38 | 0.037 | 6.54 | 0.011 | 1.92 | 0.166 | 1.58 | 0.209 | 6.97 | <0.001 |
Local population | 1 | 30.39 | <0.001 | 6.18 | 0.013 | 0.18 | 0.672 | 3.08 | 0.079 | 19.9 | <0.001 | 3.06 | 0.080 |
(a) chi-square values and significance from a (generalized) linear mixed models analysis for the emergence of juveniles in 2007, survival of plants from bulbils sowed in 2006 at the final survey in 2011, survival of adult plants emerged in 2007 at the final survey in 2011, height and number of leaves of plants at the final survey in 2011 and cumulative number of daughter bulbs produced by survived plants from 2007 to 2011 in the reciprocal transplant experiment with bulbils of Allium oleraceum; (b) local ploidy level contrast and local population contrast in emergence, survival and performance traits. χ2 and P-values are based on maximum likelihood ratio tests. Significant effects (at P ≤ 0.05) are in bold
Source of variation . | df . | Emergence (2006–07) . | Survival (2006–11) . | Survival of established plants (2007–11) . | Height (2011) . | Number of leaves (2011) . | Number of daughter bulbs (2011) . | ||||||
---|---|---|---|---|---|---|---|---|---|---|---|---|---|
(a) . | χ2 . | P . | χ2 . | P . | χ2 . | P . | χ2 . | P . | χ2 . | P . | χ2 . | P . | |
Target ploidy level habitat | 2 | 0.35 | 0.841 | 3.89 | 0.143 | 8.42 | 0.015 | 3.18 | 0.204 | 0.92 | 0.629 | 0.44 | 0.802 |
Target site | 1 | 1.21 | 0.271 | 0.01 | 0.920 | 0.02 | 0.887 | 0.48 | 0.489 | 5.01 | 0.025 | 4.03 | 0.045 |
Plot | 1 | 39.00 | <0.001 | 34.50 | <0.001 | 25.70 | <0.001 | 89.97 | <0.001 | 1.22 | 0.269 | 10.71 | 0.001 |
Ploidy level of origin | 2 | 1.06 | 0.588 | 0.57 | 0.754 | 0.43 | 0.806 | 2.93 | 0.231 | 2.15 | 0.341 | 37.74 | <0.001 |
Population of origin | 1 | 3.35 | 0.067 | 4.30 | 0.038 | 4.10 | 0.043 | 0.01 | 0.911 | 0.15 | 0.701 | 0.94 | 0.332 |
Target ploidy level habitat × Ploidy level of origin | 4 | 16.46 | 0.003 | 8.53 | 0.074 | 12.12 | 0.016 | 8.09 | 0.089 | 15.81 | 0.003 | 27.64 | <0.001 |
Marginal R2; conditional R2 | 0.04; 0.18 | 0.06; 0.14 | 0.12; 0.20 | 0.10;0.66 | 0.05; 0.27 | 0.15; 0.38 | |||||||
(b) | |||||||||||||
Local ploidy level | 1 | 0.05 | 0.827 | 4.38 | 0.037 | 6.54 | 0.011 | 1.92 | 0.166 | 1.58 | 0.209 | 6.97 | <0.001 |
Local population | 1 | 30.39 | <0.001 | 6.18 | 0.013 | 0.18 | 0.672 | 3.08 | 0.079 | 19.9 | <0.001 | 3.06 | 0.080 |
Source of variation . | df . | Emergence (2006–07) . | Survival (2006–11) . | Survival of established plants (2007–11) . | Height (2011) . | Number of leaves (2011) . | Number of daughter bulbs (2011) . | ||||||
---|---|---|---|---|---|---|---|---|---|---|---|---|---|
(a) . | χ2 . | P . | χ2 . | P . | χ2 . | P . | χ2 . | P . | χ2 . | P . | χ2 . | P . | |
Target ploidy level habitat | 2 | 0.35 | 0.841 | 3.89 | 0.143 | 8.42 | 0.015 | 3.18 | 0.204 | 0.92 | 0.629 | 0.44 | 0.802 |
Target site | 1 | 1.21 | 0.271 | 0.01 | 0.920 | 0.02 | 0.887 | 0.48 | 0.489 | 5.01 | 0.025 | 4.03 | 0.045 |
Plot | 1 | 39.00 | <0.001 | 34.50 | <0.001 | 25.70 | <0.001 | 89.97 | <0.001 | 1.22 | 0.269 | 10.71 | 0.001 |
Ploidy level of origin | 2 | 1.06 | 0.588 | 0.57 | 0.754 | 0.43 | 0.806 | 2.93 | 0.231 | 2.15 | 0.341 | 37.74 | <0.001 |
Population of origin | 1 | 3.35 | 0.067 | 4.30 | 0.038 | 4.10 | 0.043 | 0.01 | 0.911 | 0.15 | 0.701 | 0.94 | 0.332 |
Target ploidy level habitat × Ploidy level of origin | 4 | 16.46 | 0.003 | 8.53 | 0.074 | 12.12 | 0.016 | 8.09 | 0.089 | 15.81 | 0.003 | 27.64 | <0.001 |
Marginal R2; conditional R2 | 0.04; 0.18 | 0.06; 0.14 | 0.12; 0.20 | 0.10;0.66 | 0.05; 0.27 | 0.15; 0.38 | |||||||
(b) | |||||||||||||
Local ploidy level | 1 | 0.05 | 0.827 | 4.38 | 0.037 | 6.54 | 0.011 | 1.92 | 0.166 | 1.58 | 0.209 | 6.97 | <0.001 |
Local population | 1 | 30.39 | <0.001 | 6.18 | 0.013 | 0.18 | 0.672 | 3.08 | 0.079 | 19.9 | <0.001 | 3.06 | 0.080 |
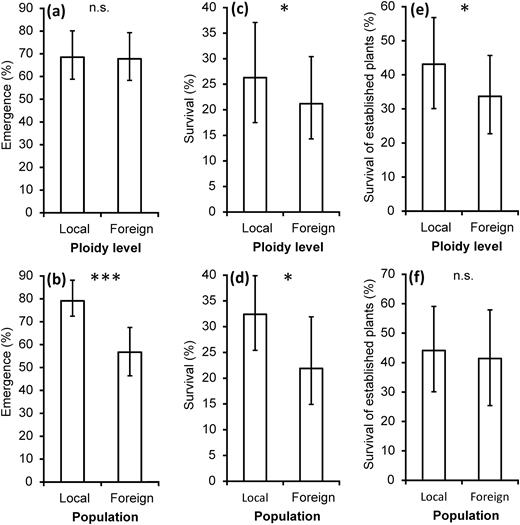
local versus foreign ploidy level contrast in (a) the emerged percentage of juveniles in 2007, (c) the survival of adult plants from sowed bulbils until 2011 and (e) the survival of plants until 2011 from established plants in 2007. Local versus foreign population contrast in (b) the emerged percentage of juveniles in 2007, (d) the survival of adult plants from sowed bulbils until 2011 and (f) the survival of adult plants until 2011 from established plants in 2007. Predicted means and 95% CIs are shown. Statistically significant differences between local and foreign ploidy levels or populations, respectively, are marked by asterisks (***P ≤ 0.001, *P ≤ 0.05, n.s. = non-significant).
After 5 years of growth (2006–11), plants originating from all populations of origin remained at all target sites. Survival of the plants derived from sowed bulbils ranged from 0 to 70% per cell but on average reached 25%. The plot within a site and population of origin had a consistent effect on the survival of plants, while the effect of the interaction of target ploidy level habitat × ploidy level of origin was weak and hardly significant (at P = 0.07; Table 1a). However, significant contrasts between local and foreign ploidy levels and between local and foreign populations were detected in survival, with local ploidies (Fig. 2c) and populations (Fig. 2d), respectively, appearing significantly superior (Table 1b). Survival of the local ploidy was always higher than foreign ploidies, but differences among cytotypes were small, especially in tetraploid target habitat (online supplementary Fig. S1). Separate analyses of each target site showed significantly higher survival of the local population compared to the foreign population of the same cytotype at two out of six sites; local plants did not survive significantly better than foreign plants at both transplanting sites at any pair of sites (online supplementary Table S2b).
Except for the target site and ploidy level of origin, all factors had a significant influence on the survival of established plants (Table 1a). Survival of the established plants after 4 years differed among target ploidy level habitats with significantly higher survival in the tetraploid habitat (mean, 95% CI; 56.1%, 42.9–69.8%) compared to the hexaploid habitat (25.1%, 15.2–35.9%) and was intermediate in the pentaploid habitat (33.0%, 21.2–45.5%). A significant interaction of target ploidy level habitat × ploidy level of origin and significant contrast between local and foreign ploidy levels were recorded (Table 1a and b; Fig. 2e), but the greater survival of local compared to foreign cytotypes was observed in target hexaploid habitat, while an only slightly greater survival of local than foreign cytotypes was found in target tetra- and pentaploid habitats (online supplementary Fig. S1). In the survival of established plants contrast between local and foreign populations was non-significant (Table 1b; Fig. 2f). Separate analyses of each target site showed significantly higher survival of the local population than the foreign population of the same cytotype at one out of six sites, but at any pair of sites at both transplanting sites, local plants did not survive significantly better than foreign plants (online supplementary Table S2c).
In all three models, marginal R2 values were relatively small (4–12%) compared with conditional R2 (14–20%). Plot within a site explained most variance of the random factors (Table 1a).
Plant performance
During 5 years of growth, no plant reached the flowering stage. The majority of surviving plants reached an immature stage, displaying one or two thin leaves or as a small sterile adult with two to three leaves. A significant interaction of target ploidy level habitat × ploidy level of origin was recorded in terms of the number of leaves (Table 1a): local cytotypes had slightly more leaves compared to foreign cytotypes (online supplementary Fig. S2). However, no contrast between local and foreign ploidy levels was significant in plant height and number of leaves (Table 1b; Fig. 3a and c). On the other hand, a contrast between local and foreign populations was significant in the number of leaves and marginally (P = 0.079) also in height (Table 1b; Fig. 3b and d). Considering each target site separately, local site plants produced significantly more leaves than foreign plants of the same cytotype at two out of six sites, but at any pair of sites, local plants did not produce significantly more leaves than foreign plants (online supplementary Table S3a). Separate analyses of each target site showed plants from the local population to be significantly taller than foreign plants of the same cytotype at one out of six sites, but at any pair of sites, local plants were not significantly taller than foreign plants (online supplementary Table S3b).
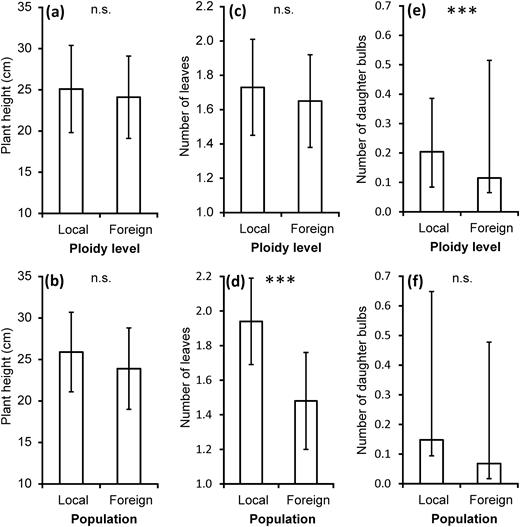
local versus foreign ploidy level contrast in (a) the plant height in 2011, (c) the number of leaves in 2011 and (e) the cumulative number of daughter bulbs 2008–11. Local versus foreign population contrast in (b) the plant height in 2011, (d) the number of leaves in 2011 and (f) the cumulative number of daughter bulbs produced by survived plants from 2008 to 2011. Predicted means and 95% CIs are shown. Statistically significant differences (P ≤ 0.05) between local and foreign ploidy levels or populations, respectively, are marked by asterisks (***P ≤ 0.001, n.s. = non-significant).
With the exception of target ploidy level habitat and population of origin, all factors had significant effects on the number of daughter bulbs produced by plants (Table 1a). The production of daughter bulbs varied among target sites and was significantly higher in hexaploids in comparison with tetra- and pentaploids (mean, 95% CI; 4×: 0.036, 0.008–0.165; 5×: 0.060, 0.013–0.278; 6×: 0.214, 0.053–0.879). Although a significant local ploidy level contrast (Table 1b) indicated the superiority of the local cytotype in the production of daughter bulbs (Fig. 3e), this could be attributed to an extreme (10×) difference between local and foreign cytotypes at one hexaploid site (Potštát). Overall, local ploidy levels produced a similar number or fewer daughter bulbs than foreign ploidies in tetra- and pentaploid habitats (online supplementary Fig. S2). The contrast between local and foreign populations was not significant (Fig. 3f). Separate analyses of each target site showed a significantly higher production of daughter bulbs by local plants than by foreign plants of the same cytotype at two out of six sites but at any pair of sites at both transplanting sites, local plants did not produce significantly more daughter bulbs than foreign plants (online supplementary Table S3c). At one target site (Lísky), no daughter bulbs were produced.
In all three models, marginal R2 values were relatively small (5–15%) compared with conditional R2 (27–66%). Plot within a site and target site explained most variance of the random factors (Table 1a).
DISCUSSION
Habitat differentiation among cytotypes
The commonly observed spatial segregation of cytotypes is usually interpreted as a consequence of niche differentiation between cytotypes (Levin 2002). Duchoslav et al. (2010) showed that realized niches of A. oleraceum cytotypes were significantly different but exhibited high overlap. The composition of vegetation varied both within and among sites used for transplant experiments, but inspection of the main gradient by DCA (Fig. 1) and the results of multivariate tests correspond well with the ecological preferences of the cytotypes reported by Duchoslav et al. (2010). The selected sites are thus typical of individual cytotypes, but did not encompass the environmental extremes (e.g. rocks, arable land and forests) that most significantly contribute to niche differentiation among cytotypes. We avoided such sites for the experiment because of difficulties with the establishment and maintenance of experimental plots or because they provide an insufficient number of propagule samples (e.g. forests; Duchoslav 2001, 2009).
Target and origin effects
The emergence of bulbils was high at all target sites (~70% on average) and in contrast with generally observed low establishment rate by seeds in natural undisturbed perennial vegetation (Harper 1977; Münzbergová and Herben 2005; Raabová et al. 2007). The high emergence rate of bulbils under natural conditions corresponds well with our previous experiments, which found that (i) a large initial size of individual bulbils in all studied cytotypes with bulbils heavier than seeds (Fialová et al. 2014) results in (ii) high emergence rates (>80%) of bulbils of all cytotypes under laboratory conditions (Fialová et al. 2014) and (iii) higher survival of established plants from bulbils compared to seeds under competition from the surrounding vegetation (Fialová 2012; Fialová and Duchoslav 2014). The advantage of using propagules of a large mass for successful establishment in specific environments (e.g. under shade, in dry habitats, under competition) is well known in plants (Moles and Westoby 2004; Westoby et al. 2002). Environmental conditions nevertheless affected emergence, survival and subsequent performance of established plants. Microsite heterogeneity affected most studied traits, as indicated by significant plot effects. Some traits were also affected by target site or target ploidy level habitat. This suggests that A. oleraceum plants can respond plastically to their environment (Becker et al. 2006).
We also detected an effect of population origin on establishment and survival but not on performance traits. Although these origin effects could indicate genetic differentiation (Linhart and Grant 1996), we must interpret them cautiously as there are alternative explanations, e.g. a maternal carry-over effect (see below). On the other hand, only one trait (production of daughter bulbs) differed among ploidy levels of origin. This is in agreement with our previous common garden and field studies, which found that all studied cytotypes are highly variable and mutually similar in most morphological traits (Fialová and Duchoslav 2014; Fialová et al. 2014). However, our previous common garden experiments also indicated three fitness-related traits (production of seeds, daughter bulbs and height of reproductive plants) that differentiated tetra- and pentaploids from hexaploids (Fialová and Duchoslav 2014; Fialová et al. 2014). Because plants in the reciprocal experiment did not reach the reproductive stage, only one clear difference among cytotypes was in line with our previous studies: hexaploids produced on average more daughter bulbs than tetra- and pentaploids. This resulted in situations wherein hexaploids produced similar or more daughter bulbs than other cytotypes even at foreign ploidy sites. The observed increase in the production of daughter bulbs in hexaploids early in plant life may explain the persistence of the hexaploids despite very little sexual reproduction (Fialová et al. 2014) and potentially give hexaploids fitness advantage over other cytotypes. Further research of how clonality via daughter bulbs may enhance the establishment of higher cytotypes and influence population dynamics is of great importance (see Baldwin and Husband 2013).
Niche differentiation among cytotypes
The lack of differences in emergence percentage between local and foreign cytotypes provides no evidence for niche differentiation among cytotypes (i.e. the first year of the experiment showed that all cytotypes are equivalent during the critical early phase of establishment). We infer from our other experiments (Fialová and Duchoslav 2014) that early developing plants generated from bulbils are probably able to mitigate the influence of local environmental conditions and competition due to their stored reserves (so-called maternal carry-over effect) and that habitat differentiation of cytotypes could appear later, after the depletion of these reserves. We indeed observed that A. oleraceum survival rates in the following years gradually decreased to ~25% survival after 5 years, but local ploidy levels fared better than foreign cytotypes. Furthermore, plants of local ploidy tended to have more leaves than those of foreign ploidies. These results thus suggest niche differentiation, albeit not large, among cytotypes.
However, our results also indicate the absence of local cytotype superiority in plant height and production of daughter bulbs despite significant local ploidy contrast found for the latter mentioned trait. Virtual superiority of local ploidy in production of daughter bulbs was caused by the extremely high production of daughter bulbs by hexaploids at one local site. Lacking or weak evidence for adaptation in vegetative growth, in contrast to reproductive traits, is frequently reported in plants (Bischoff et al. 2006) and has been reported in previous reciprocal studies comparing the vegetative traits of local and foreign cytotypes. However, under natural conditions, the transition of A. oleraceum to the flowering stage takes a long time (Duchoslav 2005). The slow development of plants observed in the transplant experiment was similar to that reported in common garden experiments utilizing A. oleraceum cytotypes (Fialová and Duchoslav 2014). Target Allium plants under strong interspecific competition were small and did not flower, whereas plants grown without competition quickly developed and flowered during the second year (Fialová and Duchoslav 2014).
This study is thus one of the few to provide evidence for the superiority of local ploidy over foreign ploidy levels. Reciprocal transplants indicate little to no ecological divergence between cytotypes (Baack and Stanton 2005; Flegrová and Krahulec 1999) or superiority of one cytotype (Buggs and Pannell 2007). Only local cytotypes of Aster amellus (Raabová et al. 2008) and C. angustifolium (Martin and Husband 2013) survived better than foreign cytotypes. However, evidence of local ploidy superiority in these two studies was dependent on the ontogenetic stage that was reciprocally transplanted.
It is likely that the studied A. oleraceum cytotypes are able to establish, at least at the studied time scale, in various types of habitats, including those occupied by other cytotypes via asexual aerial propagules (bulbils). Whether the cytotypes prosper is clearly a long-term process, and it is likely that a 5-year experiment is insufficient to fully manifest niche-based differences among cytotypes. Our results may therefore help to explain the frequent occurrence of multiple-cytotype populations, even those with unusual combinations of cytotypes (e.g. 4× + 5×, 5× + 6×), that have been observed for A. oleraceum (Duchoslav et al. 2010; Šafářová and Duchoslav 2010). We consider the origin of some of these populations to be the result of secondary contact among cytotypes (sensuPetit et al. 1999) caused by a mass effect from the surroundings or by anthropogenic dispersal as a result of agricultural practices and transport of crops and hay (Duchoslav et al. 2010). The existence of such multiple-cytotype stands is probably transient. Moreover, reproductive interactions among coexisting cytotypes resulting in gene flow (Duchoslav and Staňková 2015) further blur niche differences.
Limited local adaptation within cytotypes
Our results showed that emergence, survival and number of leaves of local plants were on average higher than those of foreign plants of the same ploidy level. The significantly better performance of local plants indicates local adaptation. However, taking a stricter approach, not all site pairs meet local vs. foreign criterion (Kawecki and Ebert 2004). On the other hand, we did not observe overall superiority for some populations. Taking into account the interpretational limits of our experiment stemming from the availability of just two sites per cytotype (see Kawecki and Ebert 2004), our results might support contributions of both adaptive and non-adaptive divergence to the pattern of fitness differentiation between A. oleraceum study populations within cytotypes.
The existence of local adaptation in plants had been assumed, but recent meta-analysis of published data based on strict local vs. foreign criterion revealed the existence of local adaptation in only 45% of cases (Leimu and Fischer 2008). Local adaptation requires local environmental stability, sufficient genetic variation, sufficient time for selection and limited gene flow (Slatkin 1987). We recently found genetic differentiation among A. oleraceum populations within studied cytotypes (Duchoslav and Staňková 2015) that is a necessary but not sufficient condition for the occurrence of local adaptation (Galen et al. 1991). Furthermore, A. oleraceum cytotypes present a patchy distribution in the contemporary landscape, suggesting that gene flow is low, which may favour local adaptation (Leimu and Fischer 2008). These findings agree with the results of our previous study concerning the responses of tetra-, penta- and hexaploids to a common competitor, indicating different population responses within each cytotype to two contrasting treatments (Fialová and Duchoslav 2014). However, many populations of studied cytotypes occupy temporally variable environments (Duchoslav et al. 2010), and cytotypes reproduce and spread mostly clonally via aerial bulbils, while the production of sexual seeds is low (Fialová et al. 2014). Consequently, within-population genetic diversity was found to be extremely low in most populations (Duchoslav and Staňková 2015), limiting effective population size. These factors could potentially constrain the evolution of local adaptation in the studied cytotypes. Leimu and Fisher (2008) indeed found that local adaptation was very rare in small populations of <500 flowering individuals (but see Yates and Fraser 2014).
In summary, our data suggest that within studied cytotypes of A. oleraceum, some populations are likely locally adapted, while others are not. Local adaptation might contribute to ecological differentiation among cytotypes due to different selection pressures in different sites. Looking at other reciprocal transplant studies focused on cytotypes, three of four published papers reported the absence of evidence of local adaptation within cytotypes. Raabová et al. (2008) argued that the absence of evidence of local adaptation in R. adoneus (Baack and Stanton 2005) and M. annua (Buggs and Pannell 2007) is related to temporal environmental variability at sites that may involve opposing selection pressures and may thus constrain adaptation. Martin and Husband (2013) explained that the absence of adaptive divergence between populations within diploid and tetraploid C. angustifolium could be due to a significant role of both drift and localized gene flow. Only in Aster amellus did Raabová et al. (2008) find local adaptation within cytotypes that contributed to the maintenance of diploid and hexaploid populations in the contact zone, despite the relatively similar environments of the studied populations.
CONCLUSIONS
Cytotypes of A. oleraceum differ in their realized niches (Duchoslav et al. 2010). The results of a reciprocal transplant experiment with sowed bulbils suggest a weak tendency towards niche differentiation among cytotypes and limited support for the existence of local adaptation within cytotypes. We did not use seeds of the studied cytotypes in our experiment because plants of all cytotypes generally produce small numbers of seeds under natural conditions (Fialová et al. 2014; Karpavičienė 2012) which limits their usefulness in the experiment. We cannot rule out that using seeds could have produced different conclusions due to a strong effect of natural selection on seedling germination (Krahulec et al. 2006; Raabová et al. 2007).
It is likely that the observed distribution of cytotypes in nature depends on additional factors, such as phenotypic plasticity (Linhart and Grant 1996), the different evolutionary ages of cytotypes (Duchoslav et al. 2013), gene flow within and among cytotypes and stochastic events connected with anthropogenic dispersal and disturbance. To disentangle the relative roles of adaptive and non-adaptive processes on fitness differentiation between and within cytotypes, an approach using multiple populations from similar vs. contrasting environments and nearby to distant regions should be applied (Becker et al. 2006; Bischoff et al. 2006; Hereford and Winn 2008).
SUPPLEMENTARY MATERIAL
Supplementary material is available at Journal of Plant Ecology online.
FUNDING
Czech Science Foundation (206/04/P115, 206/09/1126, and 16-21053S), Palacký University (PrF 2016_1).
ACKNOWLEDGEMENTS
We would like to thank Emil Tkadlec for help with statistical analysis, and František Krahulec, two anonymous reviewers and associate editor Marcia Waterway for valuable comments on the manuscript.
Conflict of interest statement. None declared.
REFERENCES
Author notes
*Correspondence address. Plant Biosystematics and Ecology RG, Department of Botany, Faculty of Science, Palacký University, Šlechtitelů 27, CZ 783 71 Olomouc, Czech Republic. Tel: +420 585 634 805; Fax: +420 585 634 002; E-mail: [email protected]