-
PDF
- Split View
-
Views
-
Cite
Cite
Eric Dumonteil, Weihong Tu, F Agustín Jiménez, Claudia Herrera, Ecological interactions of Triatoma sanguisuga (Hemiptera: Reduviidae) and risk for human infection with Trypanosoma cruzi (Kinetoplastida: Trypanosomatidae) in Illinois and Louisiana, Journal of Medical Entomology, Volume 61, Issue 6, November 2024, Pages 1282–1289, https://doi.org/10.1093/jme/tjae017
- Share Icon Share
Abstract
Triatoma sanguisuga (Leconte) is one of the most widely distributed kissing bugs in the United States, associated with an extensive zoonotic circulation of Trypanosoma cruzi, the agent of Chagas disease, in a large part of the country. However, the actual risk for human infection in the United States is poorly understood. Here, we further assessed the ecology of T. sanguisuga bugs collected in residents’ houses in Illinois and Louisiana, using a metagenomic approach to identify their blood-feeding sources, T. cruzi parasites and gut microbiota. Blood meal analysis revealed feeding on domestic animals (dogs, cats, pigs, goats, and turkeys), synanthropic species (raccoons, opossums, and squirrels), as well as the more sylvatic white-tail deer. Human blood was identified in 11/14 (78%) of bugs, highlighting a frequent vector–human contact. The infection rate with T. cruzi was 53% (8/15), and most infected bugs (6/8) had fed on humans. A total of 41 bacterial families were identified, with significant differences in microbiota alpha and beta diversity between bugs from Louisiana and Illinois. However, predicted metabolic functions remained highly conserved, suggesting important constraints to fulfill their role in bug biology. These results confirmed a significant risk for vector-borne transmission of T. cruzi to humans in Louisiana and Illinois, which warrants more active screening for human infections. Also, while there is broad plasticity in the bacterial composition of T. sanguisuga microbiota, there are strong constraints to preserve metabolic profile and function, making it a good target for novel vector control strategies.
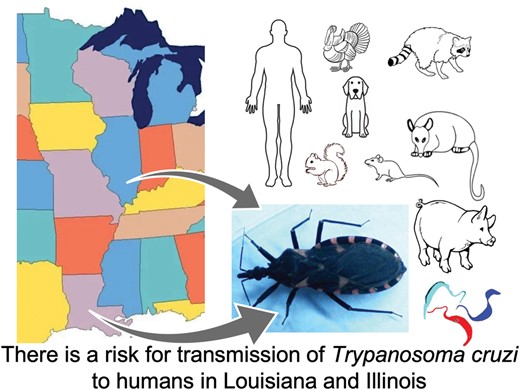
Introduction
Triatoma sanguisuga (Leconte) (Hemiptera: Reduviidae) is one of the most widely distributed kissing bug species in North America, with a geographic range spanning across most of the eastern half of the United States, from Florida to Texas in the south and Pennsylvania to Wyoming in the north (Porter 1965, Klotz et al. 2014a, Reeves and Miller 2020, Nielsen et al. 2021). This bug is associated with an extensive zoonotic circulation of Trypanosoma cruzi, the agent of Chagas disease, in the southeastern part of the country. High levels of T. cruzi infection can be found in many wild mammalian species, such as opossums (Didelphis virginiana), raccoons (Procyon lotor), mice or rats (Mus musculus, Peromyscus spp., Sigmodon spp., Neotoma spp., Rattus rattus), with infection rates as high as 40%–70% (Hodo and Hamer 2017). Infections in domestic animals such as dogs (Canis lupus familiaris) and cats (Felis catus) are less frequent but nonetheless of growing concern as indicative of spillover of the parasite into the domestic habitat and an increased risk for human infection (Tenney et al. 2014, Castillo-Neyra et al. 2015). Thus, infection rates around 10%–20% are often observed in dogs from kennels, animal shelters, and even service dogs in the southern United States (Kjos et al. 2008, Barr 2009, Meyers et al. 2017, Elmayan et al. 2019, Hodo et al. 2019, Allen and Lineberry 2022). These studies were prompted by the initial documentation of domestic parasite transmission in Texas (Beard et al. 2003). Similarly, cats can present a comparable level of infection in Texas and Louisiana (Zecca et al. 2020, Dumonteil et al. 2021).
On the other hand, human infections are more rarely detected in the United States (Beatty and Klotz 2020), although an increasing number of locally acquired cases have been reported in Texas, Louisiana, Arkansas, California, Arizona, and Mississippi (Lynn et al. 2020). Risk factors are still poorly defined but may include living in rural areas, history of hunting, camping or outdoor employment, or triatomine vector sighting (Lynn et al. 2020); in the southwest, bug feeding on humans is believed to be a significant factor (Klotz et al. 2014b). However, the lack of widespread screening, underreporting of cases, low health provider awareness of Chagas disease, and low sensitivity of diagnostic tests all contribute to a poor understanding of the actual risk of human infection in the United States (Truyens et al. 2021, Proano et al. 2023).
Previous studies on the natural history of T. sanguisuga suggest that these bugs are very opportunistic in their blood-feeding sources, and these appear to be shaped by their habitat and host availability (Waleckx et al. 2014). For example, bugs from animal shelters mostly feed on animals, as can be expected, yet feeding on humans was observed in bugs collected from residents’ houses (Dumonteil et al. 2020). Bugs also harbor a diverse gut microbiota, which plays a role in the biology of the bug and may affect their vectorial capacity (Weiss and Aksoy 2011, Miller et al. 2018, Rodriguez-Ruano et al. 2018, Wang et al. 2023). This microbiota covers multiple bacterial families, which may provide key metabolic functions such as the provision of B-complex vitamins by the endosymbiont Rhodococcus rhodnii in Rhodnius prolixus bugs (Harington 1960). The microbiota and its associated metabolic network may thus be critical for bug development, as proposed for T. sanguisuga (Teal et al. 2023). Some studies have also highlighted differences in microbiota composition associated with T. cruzi infection (Dumonteil et al. 2020, Mann et al. 2020, Murillo-Solano et al. 2021, Eberhard et al. 2022), suggesting tight interactions among parasites and bacteria that may modulate vectorial capacity (Weiss and Aksoy 2011, Wang et al. 2023). Nonetheless, a precise characterization of these processes and their role in bug development and parasite transmission is still needed. Here, we further assessed the ecology of T. sanguisuga bugs between Illinois and Louisiana, which represent the northern and southern distribution range, respectively; we focused on bugs collected in residents’ houses to better understand the risk for human T. cruzi infection. For this, we assessed ecological interactions among bugs with their blood-feeding sources, T. cruzi parasites and gut microbiota.
Materials and Methods
Bug Collections and DNA Sequencing
All bugs were collected by residents aware of the risks of Chagas disease, who sent them to the research team. These sporadic collections occurred during 2017–2022, and bugs were collected on the front porch of 4 households in Louisiana and from a single household in Illinois, all located in rural areas (Fig. 1). Bugs were stored at −20 °C until they were used. We used a metagenomic approach to characterize bugs’ genetics, their blood meal sources, their bacterial microbiota and the presence of T. cruzi as described previously (Dumonteil et al. 2018, 2020). Briefly, DNA was extracted from the distal abdomen and markers for triatomine ITS-2 (250 bp), blood meal source (12s gene, 215 bp), bacteria (16s, 430 bp), and T. cruzi parasites (mini-exon marker, 300–400 bp) were amplified by PCR. Blank DNA extractions for each batch of extraction and negative PCR controls for each reaction were included to detect potential contaminations. Amplicons were pooled for each bug for indexing and the preparation of individual libraries that were sequenced on a MiSeq Illumina platform. Raw sequences have been deposited in the NCBI SRA database under BioProject #PRJNA999759 and BioSamples #SAMN36749451-SAMN36749465.
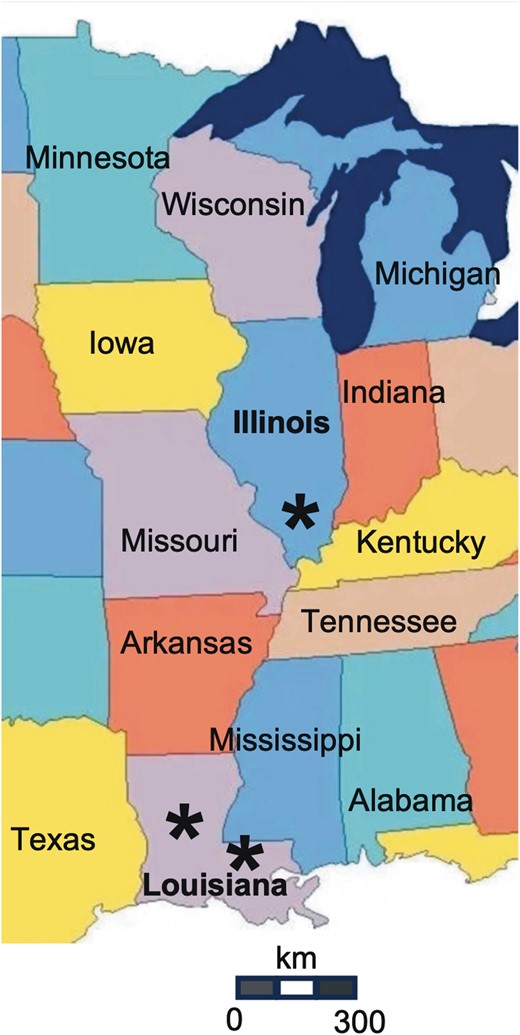
Map of bug collection sites. * indicates the location of bug collections in Illinois and Louisiana.
DNA Sequence Analysis
Raw sequences were filtered for quality, mapped to reference sequences from each marker and analyzed following protocols described elsewhere (Dumonteil et al. 2018, 2020). Briefly, for blood meal source identification, 12s sequences were analyzed using MEGABlast against NCBI Genbank database, and taxonomic identification of vertebrate hosts was performed with a threshold of ≥97% sequence identity of the full-length amplicon (215 bp). Read counts matching each host were used to calculate the proportion of blood meals on each host at the individual and group levels. The statistical significance of differences in feeding profiles was assessed by 1-way permutational ANOVA (PERMANOVA).
To assess the genetic diversity of T. cruzi parasites, mini-exon sequences were mapped to reference sequences from each parasite discrete typing unit (DTU) and used to identify haplotypes based on FreeBayes variant caller to identify SNPs. The resulting haplotypes and reference sequences were aligned with MAFFT, and Templeton, Crandall, and Sing (TCS) networks were constructed using PopArt. New T. cruzi sequences have been deposited in the NCBI Genbank database (Accession #OR296220-OR296259).
For the identification of bacteria, 16s sequences were analyzed with a Bayesian classifier from the Ribosomal Database Project (Cole et al. 2014) as implemented in Geneious Prime, with a threshold of ≥97% sequence identity for taxonomic identification at the family or genus levels. Count data were normalized using the Total Sum Scaling method. Chao1 and Shannon alpha diversity indices were calculated and compared between groups using t-tests. For beta diversity, NonMetric Dimensional Scaling (NMDS) was used based on Bray–Curtis distances, and the statistical significance of differences was assessed by 1- or 2-way PERMANOVA.
The metabolic profile of the microbiota was predicted using the AGORA database from the Virtual Metabolic Human as described elsewhere (Teal et al. 2023). This database provides metabolic reconstruction of over 800 bacterial species from microbiomes (Magnusdottir et al. 2017). The relative levels of carbon sources and fermentation products from the microbiota of T. sanguisuga were extracted for each bacterial genus and weighted based on the proportion of species within each genus able to use/produce each metabolite and the composition of the microbiota. This provided the predicted integrated metabolic profile of the bacterial community and the contribution of bacterial genera to the profile.
Results
Blood Feeding Sources
Analysis of ITS-2 sequences confirmed the taxonomic identity of all bugs collected in residents’ homes in Louisiana and Illinois as T. sanguisuga. Blood meal analysis revealed a broad diversity of feeding hosts, with the detection of 11 species in variable proportions among 14 bugs (Fig. 2A). Most feeding sources corresponded to a variety of domestic animals, including dogs, cats, pigs (Sus scrofa), goats (Ovis aries), and turkeys (Meleagris gallopavo), as can be expected in rural habitats. However, synanthropic species, including raccoons, opossums, and eastern gray squirrels (Sciurus carolensis) were detected, as well as the more sylvatic white-tail deer (Odocoilus virginianus). The later feeding sources suggest some important dispersal capabilities of the bugs from more sylvatic areas to human housing. Importantly, human blood was identified in 11/14 (78%) of bugs, highlighting a frequent vector–human contact for these bugs. Feeding on humans appeared more frequent in bugs from Illinois compared to those from Louisiana (Fig. 2A and B). Overall, the feeding profile was significantly different between Louisiana and Illinois (PERMANOVA F = 4.32, R2 = 0.26, P = 0.016), which is consistent with an opportunistic feeding of the bugs collected in localities with differences in host composition/availability. Furthermore, most bugs presented blood meals on multiple hosts, ranging from 2 to 4, further highlighting dispersal capabilities, as reported for several other triatomine species (Dumonteil et al. 2018, 2020, Murillo-Solano et al. 2021).
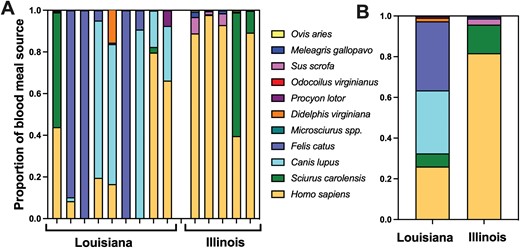
Blood meal sources of Triatoma sanguisuga. A) Individual bugs and B) grouped blood-feeding profiles are shown for bugs collected in houses from Louisiana and Illinois. Host species are color-coded as indicated. The feeding profile was significantly different between Louisiana and Illinois (PERMANOVA F = 4.32, R2 = 0.26, P = 0.016).
T. cruzi Infection
An overall infection rate with T. cruzi of 53% (8/15) was observed, corresponding to 60% (6/10) in Louisiana and 40% (2/5) in Illinois (Χ2 = 0.53, d.f. = 1, P = 0.46). Most infected bugs (6/8) had fed on humans, highlighting the potential for human infection in both regions. Infected bugs had also fed on dogs, cats, opossums, raccoons, deers, squirrels, pigs, and goats, suggesting broad potential sources of parasites.
Genotyping of T. cruzi indicated a mixture of TcI and TcIV DTUs in variable proportions in bugs from Lousiana and only TcIV in bugs from Illinois. A total of 40 haplotypes from TcI (31 haplotypes) and TcIV (9 haplotypes) were identified; these were closely related to previously reported sequences from mammalian hosts and vectors in Louisiana (Fig. 3), expanding the diversity of parasite strains circulating in the United States.
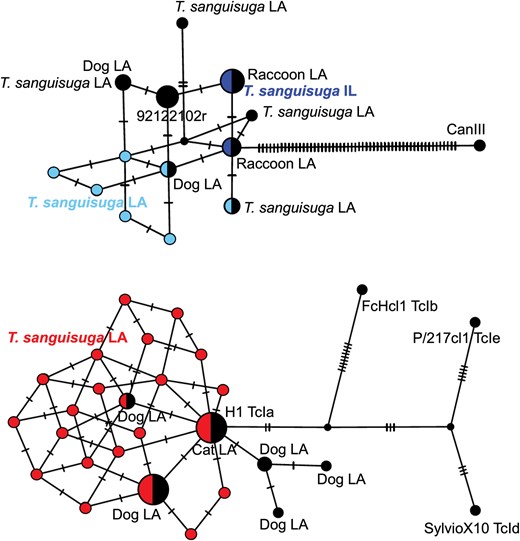
T. cruzi haplotype networks. Haplotype networks for TcIV (top) and TcI (bottom) are shown. Ticks on edges indicate nucleotide differences between pairs of sequences. Black nodes correspond to reference sequences from reference strains or previous studies in Louisiana (LA) for comparison.
Microbiota
Gut microbiota was further investigated as it may be a critical aspect of bug development and vectorial capacity (Weiss and Aksoy 2011, Diaz et al. 2016, de la Fuente et al. 2017). A large diversity of bacterial families was identified (41 families), with important individual variations in microbiota composition among bugs (Fig. 4A). However, Enterobacteriaceae, Moraxellaceae, Pseudomonadaceae, Bacillaceae, and Brevibacteriaceae predominated and represented over 50% of the microbiota in most bugs, and the remaining families accounted for limited proportions of the microbiota composition.
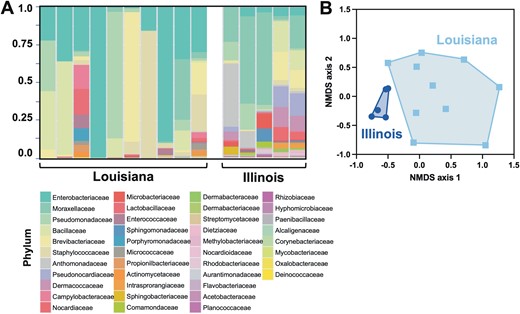
T. sanguisuga microbiota. A) Microbiota composition of individual bugs from Louisiana and Illinois. Bacterial families are color-coded as indicated. B) Beta diversity assessed by NMDS, indicating a significant difference in the composition of microbiota between bugs from Louisiana and Illinois (PERMANOVA F = 3.70, R2 = 0.22, P = 0.003).
A key factor underlying the diversity in microbiota composition was the geographic origin of the bugs, with significant differences between bugs from Louisiana and Illinois (Fig. 4A and B, PERMANOVA F = 3.70, R2 = 0.22, P = 0.003). The microbiota of bugs from Illinois also presented a significantly higher alpha diversity (34.4 ± 2.3 vs. 21.1 ± 9.8, t = 4.07, P = 0.002 and 1.92 ± 0.46 vs. 0.98 ± 0.69, t = 3.15, P = 0.009 for Chao1 and Shannon indices, respectively), with several bacterial families that were absent or underrepresented in bugs from Louisiana. For example, Pseudonocardiaceae, Caulobacteraceae, Methylobacteriaceae, Xanthomonadaceae, and Moraxellaceae were overrepresented in bugs from Illinois. On the other hand, Lactobacillaceae and Bacillaceae were more abundant in bugs from Louisiana. Microbiota composition did not vary significantly according to T. cruzi infection of the bugs (PERMANOVA F = 0.90, R2 = 0.06, P = 0.49). Also, in a multivariate analysis to assess the effect of the geographic origin of the bugs and T. cruzi infection, the effect of T. cruzi infection did not reach statistical significance (2-way PERMANOVA, F = 1.14, P = 0.25) while their geographic origin remained statistically significant (F = 2.26, P = 0.0005). Overall, these data pointed to some important plasticity in microbiota composition in T. sanguisuga based on geographic distribution.
We then assessed the potential significance of this plasticity in microbiota composition by evaluating their predicted metabolic functions. The relative abundance of carbon sources used and fermentation products was predicted based on microbial community composition. Interestingly, in spite of the major differences in bacterial composition described above, the carbon sources and fermentation products were highly similar between the microbiota of bugs from Illinois and Louisiana (Fig. 5), resulting in nearly identical metabolic profiles. Thus, specific metabolic functions were played by bacteria from different taxa in bugs from each geographic region. For example, Bacillus and Staphylococcus were major contributors for several carbon sources use in bugs from Louisiana, while this role was played by Stenotrophomonas and Micrococcus in bugs from Illinois (Fig. 5A). Similarly, Stenotrophomonas was a major producer of lactic acid in bugs from Illinois, while it was predominantly produced by Staphylococcus in bugs from Louisiana (Fig. 5B). The high similarity in metabolic profiles between the microbiota of bugs from the two regions was further demonstrated by a high pairwise correlation in carbon sources (R2 = 0.72, P < 0.0001) and in fermentation products (R2 = 0.78, P < 0.0001). These data indicate that while there is plasticity in which bacterial species may comprise T. sanguisuga gut microbiota, metabolic functions remain highly conserved, suggesting important constraints to fulfill their role in bug biology.
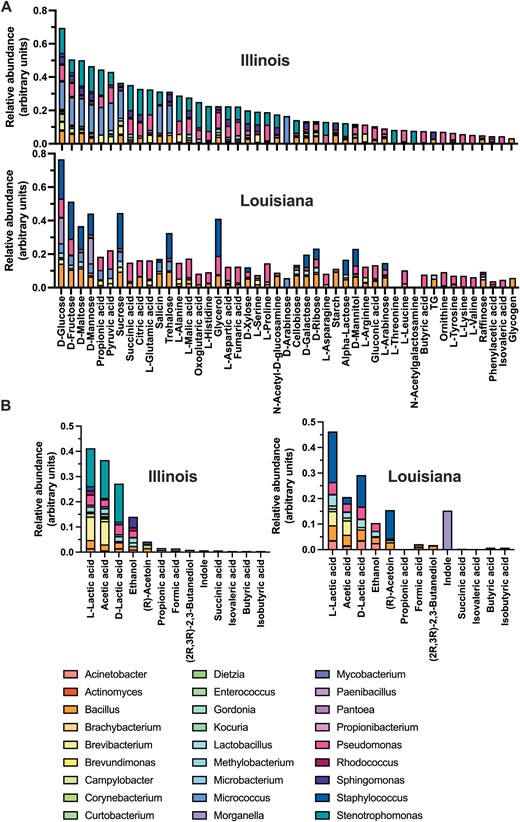
Metabolic profile of T. sanguisuga gut microbiota. A) Carbon source use and B) fermentation products relative abundance were predicted based on microbiota composition at the genus level. The contribution of each genus for the use/production of the respective metabolites is color-coded as indicated. Both carbon sources and fermentation products were highly similar between bugs from Illinois and Louisiana (R2 = 0.72, P < 0.0001 and R2 = 0.78, P < 0.0001, respectively).
Discussion
The characterization of T. sanguisuga blood-feeding profiles and ecological interactions are key to a better understanding of the risk for human infection with T. cruzi in the United States. Indeed, while the extensive zoonotic circulation of the parasite in large parts of the country is well established, the risks for parasite transmission to humans are still very poorly understood (Klotz et al. 2014a, 2014b, Beatty and Klotz 2020). Our data on T. sanguisuga blood-feeding sources and T. cruzi infection clearly point out that there is a risk for human infection over the broad geographic range of this species from Louisiana to Illinois. Indeed, we detected frequent human contact with T. cruzi-infected bugs in residents’ houses. This is similar to observations from the Southwestern United States, where triatomine bites on humans have been well documented (Klotz et al. 2014b). As the bugs analyzed here resulted from opportunistic and sporadic collections by households rather than from a rigorous sampling effort over defined geographic regions, studies with a rigorous epidemiological design are needed to assess the extent of bug presence around houses and the risk for vector–human contact. These observations are also congruent with a low probability of transmission of T. cruzi through the stercorarian route, which requires a large number of infective contacts for transmission to occur (Nouvellet et al. 2013). In addition, T. sanguisuga defecation behavior following blood feeding is very different from that of Rhodnius prolixus, resulting in a much lower probability of contaminating the host with feces (Pippin 1970). Thus, the transmission of T. cruzi vectored by T. sanguisuga bugs may require an even larger number of vector–host contacts. The diversity of feeding sources of T. sanguisuga further confirms its opportunistic feeding behavior, mostly shaped by host availability in its habitat. The feeding sources suggest some important dispersal capabilities of the bugs from more sylvatic areas to the proximity of human housing and the use of production animals as a food source.
While autochthonous human cases have increasingly been detected in the United States (Lynn et al. 2020), these detections appear to be the result of chance, as screening for T. cruzi infection is very rarely performed due to a lack of general awareness of Chagas disease. Thus, the results of the current study warrant more extensive routine screening for T. cruzi infection; with current practices, cases may be missed due to this lack of testing (Herrera et al. 2023). In a recent evaluation of the volume of screening for T. cruzi infection performed in 2 large hospitals from New Orleans, Louisiana, only 27 patients had been screened over a 3 years period, highlighting the critical need to considerably expand testing for better identification of T. cruzi infections (Proano et al. 2023).
The analysis of T. sanguisuga ecological interactions with T. cruzi parasites and gut bacterial species further shed light on some key aspects of vector biology. The detection of TcIV parasites in bugs from Illinois expands a previous report of uninfected bugs (Porter 1965) and confirms the identification of this DTU in raccoons (Vandermark et al. 2018). The widespread circulation of TcI and TcIV in bugs from Louisiana is consistent with previous observations (Dumonteil et al. 2020). The presence of a diverse gut bacterial microbiota was also detected, in general agreement with previous reports in T. sanguisuga (Rodriguez-Ruano et al. 2018, Brown et al. 2020, Dumonteil et al. 2020, Teal et al. 2023). Microbiota composition was found to be affected by the geographic origin of the bugs but not by T. cruzi infection. The latter was unexpected as previous reports have documented changes in microbiota when T. cruzi is present in several triatomine species, including T. sanguisuga (Rodriguez-Ruano et al. 2018, Dumonteil et al. 2020, Mann et al. 2020, Eberhard et al. 2022), which argue for a role of the microbiota in shaping vector competence (Weiss and Aksoy 2011). This may be due to the predominance of differences associated with the geographic distribution of the bugs. Indeed, bugs from Illinois harbored a much greater diversity of bacterial families than those from Louisiana, resulting in a significantly different microbiota composition, which likely reflects the uptake of habitat-specific bacteria during bug development in each region. Indeed, an important remodeling of the microbiota composition has been observed during development from nymphal stages to adult triatomines, indicating that many bacterial species are not vertically transmitted but acquired during development (Rodriguez-Ruano et al. 2018, Brown et al. 2020, Murillo-Solano et al. 2021, Polonio et al. 2021, Teal et al. 2023). Similar differences in microbiota composition according to geographic location have been observed in Triatoma rubida (Brown et al. 2020).
However, the geographic differences in microbial community composition in T. sanguisuga contrasted with a highly conserved metabolic profile, which rather indicated important functional constraints associated with a key role of the microbiota in bug biology/physiology. Thus, the fulfillment of important metabolic functions is likely a predominant factor modulating microbiota composition in each habitat. These observations suggested a critical biological role of the microbiota in T. sanguisuga, as noted before (Teal et al, 2023), which strengthens the idea that it may be a good target for novel vector control strategies (Beard et al. 1998).
In conclusion, this study confirmed a significant risk for vector-borne transmission of T. cruzi to humans over the broad geographic distribution of T. sanguisuga from Louisiana to Illinois. More active screening for human infections should be implemented for an improved detection of human cases. Also, tight interactions of T. sanguisuga and its gut microbiota highlight a key biological role of the microbiota, which results in a broad plasticity in bacterial composition but strong constraints to preserve its metabolic profile and function. Targeting the microbiota should thus be further explored for novel vector control strategies.
Author Contributions
Eric Dumonteil (Conceptualization [equal], Formal analysis [equal], Investigation [equal], Resources [equal], Writing—original draft [equal], Writing—review & editing [equal]), Weihong Tu (Investigation [equal], Methodology [equal], Writing—review & editing [equal]), F. Agustín Jiménez (Conceptualization [equal], Investigation [equal], Resources [equal], Writing—review & editing [equal]), and Claudia Herrera (Conceptualization [equal], Investigation [equal], Writing—review & editing [equal])