-
PDF
- Split View
-
Views
-
Cite
Cite
Ting Li, Nannan Liu, The Function of G-Protein-Coupled Receptor-Regulatory Cascade in Southern House Mosquitoes (Diptera: Culicidae), Journal of Medical Entomology, Volume 55, Issue 4, July 2018, Pages 862–870, https://doi.org/10.1093/jme/tjy022
- Share Icon Share
Abstract
G-protein-coupled receptors (GPCRs) are a large family of seven-transmembrane domain proteins that exist in plants and animals, playing critical physiological functions through intracellular cascades. Previous studies revealed an important regulation pathway of GPCR/Guanine nucleotide-binding protein (G-protein)/Adenylyl Cyclase (AC)/cAMP-dependent protein kinase A (PKA) in the insecticide resistance and regulation of resistance-related P450 gene expression in highly resistant southern house mosquitoes, Culex quinquefasciatus Say (Diptera: Culicidae). However, the function of this regulation pathway in field-collected and laboratory-susceptible mosquitoes is still unknown. In the current study, we characterized the function of each effector (GPCR, G-protein, AC, and PKA) in this GPCR intracellular pathway in both field-collected and laboratory Cx. quinquefasciatus strains, showing that knockdown of the expression of each effector gene can cause 1) decreased expression of their downstream respective genes and 2) increased sensitivity of the mosquitoes to permethrin insecticide. These results, together with our previous findings, strongly suggest the universal function of the GPCR-regulation cascade in the mosquito’s sensitivity to insecticides and its regulation of resistance development through P450-mediated detoxification.
The southern house mosquito, Culex quinquefasciatus Say (Diptera: Culicidae), is a primary vector of a number of serious human diseases including West Nile encephalitis, St. Louis encephalitis, and lymphatic filariasis (Farajollahi et al. 2011). Pyrethroids represent as one of the major insecticide classes available for use on long-lasting insecticide nets (LLINs) used for Anopheles mosquito control in many countries (WHO 2016a) and also used for ultra-low volume (ULV) application for adult Culex control to interrupt outbreaks of MNV (Lothrop et al. 2007). However, decreased sensitivity of mosquitoes to insecticides has been reported in 60 countries since 2010 and has led to a dramatic increase in mosquito-borne disease prevalence worldwide (WHO 2016b). To prevent the development of insecticide resistance in mosquito populations, a better understanding of the resistance mechanisms involved and the regulation pathways governing resistance genes is vital, if current mosquito control approaches are to remain effective and innovative vector–control tools developed (Liu 2015). Among the insect resistance mechanisms identified so far, cytochrome P450-mediated detoxification has been widely recognized and characterized in mosquitoes (Liu 2015). However, as yet our understanding of the regulation, regulatory intracellular cascades, and effectors involved in P450-mediated resistance is in its infancy.
G-protein-coupled receptors (GPCRs) are a large family of seven-transmembrane domain proteins that are present in both plants and animals (Perez 2005). GPCRs are known to be extremely versatile receptors that transduce diverse extracellular signals into intracellular signaling pathways to regulate cellular physiological responses (Ferguson 2001). Therefore, exploring the functions of GPCR-regulated cell signaling pathways is critical for therapeutic and new drug discovery (Jacoby et al. 2006). In insects, GPCRs have been identified that are involved in a number of physiology functions, including locomotor activity (Socha et al. 1999), development (Bai et al. 2011), reproduction (Yapici et al. 2008), and immune response (Di Prisco et al. 2013). However, as yet, the precise role of GPCRs in insecticide resistance remains largely unclear. In a previous study, we characterized the function of constitutively expressed GPCRs in insecticide resistant (laboratory selected) Cx. quinquefasciatus through regulating downstream gene expression including G-protein subunit-Gαs, ACs, PKAs, and resistance-related P450 genes (Li et al. 2014, 2015; Li and Liu 2017). However, the critical role of this intracellular cascade in the field strains HAmCqG0 and MAmCqG0 (Liu et al. 2004) and susceptible strains are still unclear. To identify the universal function of these effectors in insecticide susceptibility and/or resistance, the current study focused on identifying the inclusive role of this GPCR-regulation pathway in mosquitoes’ susceptibility to insecticides by characterizing the precise function of the GPCR, G-protein, ACs, and PKAs in laboratory susceptible and field strains of Cx. quinquefasciatus.
Materials and Methods
Mosquito Strains
Three strains of Cx. quinquefasciatus were utilized in this study. The insecticide susceptible S-Lab strain was obtained from Dr. Laura Harrington (Cornell University) and is routinely used as a reference strain for insecticide resistance comparisons. HAmCqG0 and MAmCqG0 strains were colonized from collections made from Madison County and Mobile County, AL, respectively, in 2002 (Liu et al. 2004). These two counties are located more than 600 km apart, in the north and the south of the state, respectively. Neither mosquito strain had been treated with insecticides since collection and both were reared at 25 ± 2°C under a photoperiod of 12:12 (L:D) h and 70–80% humidity. Larvae bioassay results showed a ~10-fold resistance to permethrin in both strains in comparison with S-Lab strain (Li and Liu 2010). Female adults were fed on blood from cows obtained from the Large Animal Teaching Hospital, College of Veterinary Medicine, Auburn University, AL.
Double-Stranded RNA Synthesis and Microinjection
To better understand the resistance mechanisms in mosquitoes, we conducted double-stranded RNA (dsRNA) injection in the early third-instar mosquitoes, which showed higher resistance levels compared to adult stages (Li and Liu 2010). The dsRNA with length of ~300 to 600 bp was synthesized according to the length of the target genes following the manufacturer’s instructions using a MEGAscript T7 High Yield Transcription Kit (Ambion by Life technologies Corporation, Grand Island, NY). The primers were designed for each gene (CPIJ020021, -006458, -004739, -007240, -000798, -018257) and modified with the T7 promotor sequences (Table 1). The dsRNA synthesized was purified by phenol/chloroform extraction followed by ethanol precipitation. A dsRNA of a green fluorescent protein (GFP) gene with a sequence that was complementary to the pMW1650 plasmid served as the negative control. Noninjected mosquitoes were used for the calibration. The larval microinjection method was as previously described (Li et al. 2015). In brief, the early-third-instar larvae were transferred from clean water to dry filter paper to immobilize the larvae and each was individually injected with ~400 ng of dsRNA-GPCR, -Gαs, -ACs, -PKAs, or the GFP control using the Picospritzer III injector system (Parker Instrumentation) under a Nikon Eclipse TS100 microscope (Nikon Instruments). After injection, the larvae were transferred immediately from the filter paper to distilled water and then reared under insectary conditions. After 72 h, the late-third instar or early-fourth instar were separated into two groups, one of which was utilized for the gene expression examination (~30 larvae), and the other group utilized for larval bioassays (~150 larvae). Each experiment was repeated at least three times on different days with independent injection and RNA extraction.
Primer description . | Primer name . | Primer sequence . |
---|---|---|
18S Ribosomal RNA | 18S rRNA F1 | 5ʹCGCGGTAATTCCAGCTCCACTA3ʹ |
18S rRNA R1 | 5ʹ GCATCAAGCGCCACCATATAGG3ʹ | |
GPCR020021 Real-time PCR | qPCR GPCR020021 F | 5ʹACTACCTCACCGACACCTTCTC3ʹ |
qPCR GPCR020021 R | 5ʹGCCTTGATGATGAAGATG3ʹ | |
Gαs006458 Real-time PCR | qPCRGαs006458F | 5ʹCATCCGGTCCTGACTTCAATTA3ʹ |
qPCRGαs006458R | 5ʹTTCGTTTGATCGCTCGTAGG3ʹ | |
AC004739 Real-time PCR | qRT AC004739 F | 5ʹGATTCTCGGCGATTGCTACT3ʹ |
qRT AC004739 R | 5ʹCTTCGCGGACGAACCTTATAG3ʹ | |
AC007240 Real-time PCR | qRT AC007240 F | 5ʹGCGAGAAGAACATCGAGGAA3ʹ |
qRT AC007240 R | 5ʹGCCCTCCAGTTCGATGTAAA3ʹ | |
PKA000798 Real-time PCR | qPCRPKA000798F | 5ʹTTGATTGGTGGGCATTAGGCGTTC3ʹ |
qPCRPKA000798R | 5ʹAGCAGCTTCTTGACCAGGTCCTTT3ʹ | |
PKA018257 Real-time PCR | qPCRPKA018257F | 5ʹATACCGTGACTTGAAGCCGGAGAA3ʹ |
qPCRPKA018257R | 5ʹAATTTGTATTGGCTGATCAGC3ʹ | |
CYP9M10 Real-time PCR | qPCRP4509M10F | 5ʹATGCAGACCAAGTGCTTCCTGTAC3ʹ |
qPCRP4509M10R | 5ʹAACCCACTCAACGTATCCAGCGAA3ʹ | |
CYP9J40 Real-time PCR | qPCRP4509J40F | 5ʹACCCGAATCCGGGCAAGTTTGAT3ʹ |
qPCRP4509J40R | 5ʹAACTCCAAACGGTAAATACGCCGC3ʹ | |
CYP6AA7 Real-time PCR | qPCRP4506AA7F | 5ʹATGACGCTGATTCCCGAGACTGTT3ʹ |
qPCRP4506AA7R | 5ʹTTCATGGTCAAGGTCTCACCCGAA3ʹ | |
CYP9J34 Real-time PCR | qPCRP4509J34F | 5ʹATCCGATGTCGGTAAAGTGCAGGT3ʹ |
qPCRP4509J34R | 5ʹTGTACCTCTGGGTTGATGGCAAGT3ʹ | |
GPCR020021dsRNAsynthesis | dsRNAGPCR020021F | 5ʹTAATACGACTCACTATAGGGGCCATCTTCTTCCTGTGC3ʹ |
dsRNAGPCR020021R | 5ʹTAATACGACTCACTATAGGGCGGGGCGAAGTACACGAA3ʹ | |
Gαs006458 dsRNA synthesis | dsRNAGαs006458F | 5ʹTAATACGACTCACTATAGGGTCGCTGGAGCTGTTCAAA3ʹ |
dsRNAGαs006458R | 5ʹTAATACGACTCACTATAGGGTGTCTACCGCACAGGTAAAG3ʹ | |
AC007240dsRNA synthesis | dsRNAAC007240 F | 5ʹTAATACGACTCACTATAGGGCAAGGTGTGCGTGATGTTTG3ʹ |
dsRNAAC007240 R | 5ʹTAATACGACTCACTATAGGGCGTTCACGTCCGTAATCTTCT3ʹ | |
AC004739dsRNA synthesis | dsRNAAC004739F | 5ʹTAATACGACTCACTATAGGGCCTGTACTACCGCATCATGTC3ʹ |
dsRNAAC004739R | 5ʹTAATACGACTCACTATAGGGCTTCGCGGACGAACCTTATAG3ʹ | |
PKA000798 dsRNA synthesis | dsRNAPKA000798F | 5ʹTAATACGACTCACTATAGGGTGAAGCAGATCGAGCACGTCAA3ʹ |
dsRNAPKA000798R | 5ʹTAATACGACTCACTATAGGGAGATGCCGAACGGATTATCGTC3ʹ | |
PKA018257 dsRNA synthesis | dsRNAPKA18257F | 5ʹTAATACGACTCACTATAGGGCAAGTTGAGCACACCCTAAA3ʹ |
dsRNAPKA18257R | 5ʹTAATACGACTCACTATAGGGCTTCAAGTCACGGTATATCA3ʹ | |
GFP gene dsRNA synthesis | dsRNAGFPF | 5ʹTAATACGACTCACTATAGGGAGAAGAACTTTTCACTGG3ʹ |
dsRNAGFPR | 5ʹTAATACGACTCACTATAGGGCTTCTACCTAGGCAAGTT3ʹ |
Primer description . | Primer name . | Primer sequence . |
---|---|---|
18S Ribosomal RNA | 18S rRNA F1 | 5ʹCGCGGTAATTCCAGCTCCACTA3ʹ |
18S rRNA R1 | 5ʹ GCATCAAGCGCCACCATATAGG3ʹ | |
GPCR020021 Real-time PCR | qPCR GPCR020021 F | 5ʹACTACCTCACCGACACCTTCTC3ʹ |
qPCR GPCR020021 R | 5ʹGCCTTGATGATGAAGATG3ʹ | |
Gαs006458 Real-time PCR | qPCRGαs006458F | 5ʹCATCCGGTCCTGACTTCAATTA3ʹ |
qPCRGαs006458R | 5ʹTTCGTTTGATCGCTCGTAGG3ʹ | |
AC004739 Real-time PCR | qRT AC004739 F | 5ʹGATTCTCGGCGATTGCTACT3ʹ |
qRT AC004739 R | 5ʹCTTCGCGGACGAACCTTATAG3ʹ | |
AC007240 Real-time PCR | qRT AC007240 F | 5ʹGCGAGAAGAACATCGAGGAA3ʹ |
qRT AC007240 R | 5ʹGCCCTCCAGTTCGATGTAAA3ʹ | |
PKA000798 Real-time PCR | qPCRPKA000798F | 5ʹTTGATTGGTGGGCATTAGGCGTTC3ʹ |
qPCRPKA000798R | 5ʹAGCAGCTTCTTGACCAGGTCCTTT3ʹ | |
PKA018257 Real-time PCR | qPCRPKA018257F | 5ʹATACCGTGACTTGAAGCCGGAGAA3ʹ |
qPCRPKA018257R | 5ʹAATTTGTATTGGCTGATCAGC3ʹ | |
CYP9M10 Real-time PCR | qPCRP4509M10F | 5ʹATGCAGACCAAGTGCTTCCTGTAC3ʹ |
qPCRP4509M10R | 5ʹAACCCACTCAACGTATCCAGCGAA3ʹ | |
CYP9J40 Real-time PCR | qPCRP4509J40F | 5ʹACCCGAATCCGGGCAAGTTTGAT3ʹ |
qPCRP4509J40R | 5ʹAACTCCAAACGGTAAATACGCCGC3ʹ | |
CYP6AA7 Real-time PCR | qPCRP4506AA7F | 5ʹATGACGCTGATTCCCGAGACTGTT3ʹ |
qPCRP4506AA7R | 5ʹTTCATGGTCAAGGTCTCACCCGAA3ʹ | |
CYP9J34 Real-time PCR | qPCRP4509J34F | 5ʹATCCGATGTCGGTAAAGTGCAGGT3ʹ |
qPCRP4509J34R | 5ʹTGTACCTCTGGGTTGATGGCAAGT3ʹ | |
GPCR020021dsRNAsynthesis | dsRNAGPCR020021F | 5ʹTAATACGACTCACTATAGGGGCCATCTTCTTCCTGTGC3ʹ |
dsRNAGPCR020021R | 5ʹTAATACGACTCACTATAGGGCGGGGCGAAGTACACGAA3ʹ | |
Gαs006458 dsRNA synthesis | dsRNAGαs006458F | 5ʹTAATACGACTCACTATAGGGTCGCTGGAGCTGTTCAAA3ʹ |
dsRNAGαs006458R | 5ʹTAATACGACTCACTATAGGGTGTCTACCGCACAGGTAAAG3ʹ | |
AC007240dsRNA synthesis | dsRNAAC007240 F | 5ʹTAATACGACTCACTATAGGGCAAGGTGTGCGTGATGTTTG3ʹ |
dsRNAAC007240 R | 5ʹTAATACGACTCACTATAGGGCGTTCACGTCCGTAATCTTCT3ʹ | |
AC004739dsRNA synthesis | dsRNAAC004739F | 5ʹTAATACGACTCACTATAGGGCCTGTACTACCGCATCATGTC3ʹ |
dsRNAAC004739R | 5ʹTAATACGACTCACTATAGGGCTTCGCGGACGAACCTTATAG3ʹ | |
PKA000798 dsRNA synthesis | dsRNAPKA000798F | 5ʹTAATACGACTCACTATAGGGTGAAGCAGATCGAGCACGTCAA3ʹ |
dsRNAPKA000798R | 5ʹTAATACGACTCACTATAGGGAGATGCCGAACGGATTATCGTC3ʹ | |
PKA018257 dsRNA synthesis | dsRNAPKA18257F | 5ʹTAATACGACTCACTATAGGGCAAGTTGAGCACACCCTAAA3ʹ |
dsRNAPKA18257R | 5ʹTAATACGACTCACTATAGGGCTTCAAGTCACGGTATATCA3ʹ | |
GFP gene dsRNA synthesis | dsRNAGFPF | 5ʹTAATACGACTCACTATAGGGAGAAGAACTTTTCACTGG3ʹ |
dsRNAGFPR | 5ʹTAATACGACTCACTATAGGGCTTCTACCTAGGCAAGTT3ʹ |
Primer description . | Primer name . | Primer sequence . |
---|---|---|
18S Ribosomal RNA | 18S rRNA F1 | 5ʹCGCGGTAATTCCAGCTCCACTA3ʹ |
18S rRNA R1 | 5ʹ GCATCAAGCGCCACCATATAGG3ʹ | |
GPCR020021 Real-time PCR | qPCR GPCR020021 F | 5ʹACTACCTCACCGACACCTTCTC3ʹ |
qPCR GPCR020021 R | 5ʹGCCTTGATGATGAAGATG3ʹ | |
Gαs006458 Real-time PCR | qPCRGαs006458F | 5ʹCATCCGGTCCTGACTTCAATTA3ʹ |
qPCRGαs006458R | 5ʹTTCGTTTGATCGCTCGTAGG3ʹ | |
AC004739 Real-time PCR | qRT AC004739 F | 5ʹGATTCTCGGCGATTGCTACT3ʹ |
qRT AC004739 R | 5ʹCTTCGCGGACGAACCTTATAG3ʹ | |
AC007240 Real-time PCR | qRT AC007240 F | 5ʹGCGAGAAGAACATCGAGGAA3ʹ |
qRT AC007240 R | 5ʹGCCCTCCAGTTCGATGTAAA3ʹ | |
PKA000798 Real-time PCR | qPCRPKA000798F | 5ʹTTGATTGGTGGGCATTAGGCGTTC3ʹ |
qPCRPKA000798R | 5ʹAGCAGCTTCTTGACCAGGTCCTTT3ʹ | |
PKA018257 Real-time PCR | qPCRPKA018257F | 5ʹATACCGTGACTTGAAGCCGGAGAA3ʹ |
qPCRPKA018257R | 5ʹAATTTGTATTGGCTGATCAGC3ʹ | |
CYP9M10 Real-time PCR | qPCRP4509M10F | 5ʹATGCAGACCAAGTGCTTCCTGTAC3ʹ |
qPCRP4509M10R | 5ʹAACCCACTCAACGTATCCAGCGAA3ʹ | |
CYP9J40 Real-time PCR | qPCRP4509J40F | 5ʹACCCGAATCCGGGCAAGTTTGAT3ʹ |
qPCRP4509J40R | 5ʹAACTCCAAACGGTAAATACGCCGC3ʹ | |
CYP6AA7 Real-time PCR | qPCRP4506AA7F | 5ʹATGACGCTGATTCCCGAGACTGTT3ʹ |
qPCRP4506AA7R | 5ʹTTCATGGTCAAGGTCTCACCCGAA3ʹ | |
CYP9J34 Real-time PCR | qPCRP4509J34F | 5ʹATCCGATGTCGGTAAAGTGCAGGT3ʹ |
qPCRP4509J34R | 5ʹTGTACCTCTGGGTTGATGGCAAGT3ʹ | |
GPCR020021dsRNAsynthesis | dsRNAGPCR020021F | 5ʹTAATACGACTCACTATAGGGGCCATCTTCTTCCTGTGC3ʹ |
dsRNAGPCR020021R | 5ʹTAATACGACTCACTATAGGGCGGGGCGAAGTACACGAA3ʹ | |
Gαs006458 dsRNA synthesis | dsRNAGαs006458F | 5ʹTAATACGACTCACTATAGGGTCGCTGGAGCTGTTCAAA3ʹ |
dsRNAGαs006458R | 5ʹTAATACGACTCACTATAGGGTGTCTACCGCACAGGTAAAG3ʹ | |
AC007240dsRNA synthesis | dsRNAAC007240 F | 5ʹTAATACGACTCACTATAGGGCAAGGTGTGCGTGATGTTTG3ʹ |
dsRNAAC007240 R | 5ʹTAATACGACTCACTATAGGGCGTTCACGTCCGTAATCTTCT3ʹ | |
AC004739dsRNA synthesis | dsRNAAC004739F | 5ʹTAATACGACTCACTATAGGGCCTGTACTACCGCATCATGTC3ʹ |
dsRNAAC004739R | 5ʹTAATACGACTCACTATAGGGCTTCGCGGACGAACCTTATAG3ʹ | |
PKA000798 dsRNA synthesis | dsRNAPKA000798F | 5ʹTAATACGACTCACTATAGGGTGAAGCAGATCGAGCACGTCAA3ʹ |
dsRNAPKA000798R | 5ʹTAATACGACTCACTATAGGGAGATGCCGAACGGATTATCGTC3ʹ | |
PKA018257 dsRNA synthesis | dsRNAPKA18257F | 5ʹTAATACGACTCACTATAGGGCAAGTTGAGCACACCCTAAA3ʹ |
dsRNAPKA18257R | 5ʹTAATACGACTCACTATAGGGCTTCAAGTCACGGTATATCA3ʹ | |
GFP gene dsRNA synthesis | dsRNAGFPF | 5ʹTAATACGACTCACTATAGGGAGAAGAACTTTTCACTGG3ʹ |
dsRNAGFPR | 5ʹTAATACGACTCACTATAGGGCTTCTACCTAGGCAAGTT3ʹ |
Primer description . | Primer name . | Primer sequence . |
---|---|---|
18S Ribosomal RNA | 18S rRNA F1 | 5ʹCGCGGTAATTCCAGCTCCACTA3ʹ |
18S rRNA R1 | 5ʹ GCATCAAGCGCCACCATATAGG3ʹ | |
GPCR020021 Real-time PCR | qPCR GPCR020021 F | 5ʹACTACCTCACCGACACCTTCTC3ʹ |
qPCR GPCR020021 R | 5ʹGCCTTGATGATGAAGATG3ʹ | |
Gαs006458 Real-time PCR | qPCRGαs006458F | 5ʹCATCCGGTCCTGACTTCAATTA3ʹ |
qPCRGαs006458R | 5ʹTTCGTTTGATCGCTCGTAGG3ʹ | |
AC004739 Real-time PCR | qRT AC004739 F | 5ʹGATTCTCGGCGATTGCTACT3ʹ |
qRT AC004739 R | 5ʹCTTCGCGGACGAACCTTATAG3ʹ | |
AC007240 Real-time PCR | qRT AC007240 F | 5ʹGCGAGAAGAACATCGAGGAA3ʹ |
qRT AC007240 R | 5ʹGCCCTCCAGTTCGATGTAAA3ʹ | |
PKA000798 Real-time PCR | qPCRPKA000798F | 5ʹTTGATTGGTGGGCATTAGGCGTTC3ʹ |
qPCRPKA000798R | 5ʹAGCAGCTTCTTGACCAGGTCCTTT3ʹ | |
PKA018257 Real-time PCR | qPCRPKA018257F | 5ʹATACCGTGACTTGAAGCCGGAGAA3ʹ |
qPCRPKA018257R | 5ʹAATTTGTATTGGCTGATCAGC3ʹ | |
CYP9M10 Real-time PCR | qPCRP4509M10F | 5ʹATGCAGACCAAGTGCTTCCTGTAC3ʹ |
qPCRP4509M10R | 5ʹAACCCACTCAACGTATCCAGCGAA3ʹ | |
CYP9J40 Real-time PCR | qPCRP4509J40F | 5ʹACCCGAATCCGGGCAAGTTTGAT3ʹ |
qPCRP4509J40R | 5ʹAACTCCAAACGGTAAATACGCCGC3ʹ | |
CYP6AA7 Real-time PCR | qPCRP4506AA7F | 5ʹATGACGCTGATTCCCGAGACTGTT3ʹ |
qPCRP4506AA7R | 5ʹTTCATGGTCAAGGTCTCACCCGAA3ʹ | |
CYP9J34 Real-time PCR | qPCRP4509J34F | 5ʹATCCGATGTCGGTAAAGTGCAGGT3ʹ |
qPCRP4509J34R | 5ʹTGTACCTCTGGGTTGATGGCAAGT3ʹ | |
GPCR020021dsRNAsynthesis | dsRNAGPCR020021F | 5ʹTAATACGACTCACTATAGGGGCCATCTTCTTCCTGTGC3ʹ |
dsRNAGPCR020021R | 5ʹTAATACGACTCACTATAGGGCGGGGCGAAGTACACGAA3ʹ | |
Gαs006458 dsRNA synthesis | dsRNAGαs006458F | 5ʹTAATACGACTCACTATAGGGTCGCTGGAGCTGTTCAAA3ʹ |
dsRNAGαs006458R | 5ʹTAATACGACTCACTATAGGGTGTCTACCGCACAGGTAAAG3ʹ | |
AC007240dsRNA synthesis | dsRNAAC007240 F | 5ʹTAATACGACTCACTATAGGGCAAGGTGTGCGTGATGTTTG3ʹ |
dsRNAAC007240 R | 5ʹTAATACGACTCACTATAGGGCGTTCACGTCCGTAATCTTCT3ʹ | |
AC004739dsRNA synthesis | dsRNAAC004739F | 5ʹTAATACGACTCACTATAGGGCCTGTACTACCGCATCATGTC3ʹ |
dsRNAAC004739R | 5ʹTAATACGACTCACTATAGGGCTTCGCGGACGAACCTTATAG3ʹ | |
PKA000798 dsRNA synthesis | dsRNAPKA000798F | 5ʹTAATACGACTCACTATAGGGTGAAGCAGATCGAGCACGTCAA3ʹ |
dsRNAPKA000798R | 5ʹTAATACGACTCACTATAGGGAGATGCCGAACGGATTATCGTC3ʹ | |
PKA018257 dsRNA synthesis | dsRNAPKA18257F | 5ʹTAATACGACTCACTATAGGGCAAGTTGAGCACACCCTAAA3ʹ |
dsRNAPKA18257R | 5ʹTAATACGACTCACTATAGGGCTTCAAGTCACGGTATATCA3ʹ | |
GFP gene dsRNA synthesis | dsRNAGFPF | 5ʹTAATACGACTCACTATAGGGAGAAGAACTTTTCACTGG3ʹ |
dsRNAGFPR | 5ʹTAATACGACTCACTATAGGGCTTCTACCTAGGCAAGTT3ʹ |
Permethrin Insecticide Bioassay
The bioassay method used for the larvae was as described in previous studies (Li and Liu, 2010). Stock and serial dilutions of permethrin (94.34%, supplied by FMC Corp., Princeton, NJ) for the insecticide bioassays were prepared in acetone. Each bioassay consisted of 30 third-instar mosquito larvae 3 d after each GPCR-, Gαs-, AC, PKA-dsRNA-, or GFP-injection or noninjection in tap water with 1% insecticide solution (in acetone) at the required concentration. Three or four concentrations that give >0 and <100% mortality. Control groups received only 1% acetone. Mortality was assessed after 24 h. All tests were run at 25oC and each assay was replicated at least three times. Bioassay data were pooled and analyzed by a standard probit analysis (Raymond 1985). The statistical analysis for the insecticide bioassay was conducted by examining the LC50 values based on nonoverlapping 95% CIs. Resistance ratios (RRs) were calculated by dividing the LC50 of the specific gene- or GFP-injected mosquitoes by the LC50 of the noninjected mosquitoes.
RNA Extraction, cDNA Preparation, and Quantitative Real-Time Polymerase Chain Reaction
Three mosquito strains, S-Lab, HAmCqG0, and MAmCqG0, were utilized in this study. Total RNAs were extracted from dsRNA-injected and noninjected mosquito larvae. Prior to cDNA synthesis, genomic DNA was removed from the total RNA using a TURBO DNA-free kit (Ambion) following the manufacturer’s instructions. cDNA was synthesized by SuperScript® IV Reverse Transcriptase (Invitrogen) following the method specified in the kit manual. The quantitative real-time polymerase chain reaction (qRT-PCR) was performed using the All-in-One qPCR Mix (GeneCopoeia) and CFX96 Real Time system. Each qRT-PCR (25 µl) consisted of SYBR Green master mix, specific primer pairs for genes (Table 1) at final concentrations of 0.2 µM, and a 1 µg cDNA template from each mosquito sample site. The negative control was a ‘no-template’ reaction. The reaction cycle applied the following PCR program: a melting step of 95°C for 10 min, followed by 40 cycles of 95°C for 10 s, 60°C for 20 s, and 72°C for 15 s. At the end of the final cycle, the temperature was increased from 72 to 95°C to produce a melting curve to assess the specificity of each PCR, which was done using Dissociation Curves software (Wittwer et al. 1997). All the reactions were run on three technical replicates. Relative expression levels of target genes were analyzed by the 2-∆∆CT method using SDS RQ software (Livak and Schmittgen 2001). The 18S ribosomal RNA (rRNA) gene served as an endogenous control because of its constitutive expression in all samples (Li et al. 2014, 2015). Each experiment was repeated on more than three independent isolated RNA mosquito samples.
Statistical Analysis
The statistical data analysis was conducted using a Student’s t-test for all two-sample comparisons and a one-way analysis of variance for multiple sample comparisons (Statistical Package for the Social Sciences-SPSS software with both least significant difference and Tukey’s tests for analysis of significance of means through multiple comparison); a value of P ≤ 0.05 was considered statistically significant.
Results
The Function of the GPCR and Gαs in the S-Lab and Field Mosquito Strains
To identify the primary function of the GPCR in the insecticide sensitivity of susceptible S-Lab and field strains of HAmCqG0 and MAmCqG0 (Liu et al. 2004), we utilized the RNAi technique to knockdown one GPCR020021 gene, examining its downstream respective gene expression and insecticide sensitivity of mosquitoes to permethrin insecticide. Knockdown of GPCR020021 gene expression in S-Lab caused a significant increase in the susceptibility of mosquitoes to permethrin compared with noninjected and GFP-injected mosquitoes (Table 2). Meanwhile, the expression of the downstream respective genes (AC007240, PKA-018257, and PKA-00798), and P450 genes (CYP9M10, CYP6AA7, and CYP9J40) was decreaed in S-Lab following GPCR020021 knockdown (Fig. 1A, Supp Table 1 [online only]). Knockdown of GPCR020021 gene expression in HAmCqG0 resulted in a decreased resistance to permethrin (Table 2) and reduced expression of AC007240, PKA018257, PKA000798, and all four P450 genes tested (Fig. 1B, Supp Table 1 [online only]). Knockdown of GPCR020021 gene in MAmCqG0 showed decreased resistance of mosquito to permethrin (Table 2) and decreased expression of AC004739, -007240, PKA000798, CYP6AA7, CYP9J34, and CYP9J40 (Fig. 1C, Supp Table 1 [online only]).
Insecticide resistance in mosquitoes microinjected with RNAi to silence specific genes
Target gene . | RNAi . | S-Lab . | HAmCqG0 . | MAmCqG0 . | |||
---|---|---|---|---|---|---|---|
LC501 (CI)2 . | Ratio3 (mean ± SE) . | LC501(CI)2 . | Ratio3 (mean ± SE) . | LC501 (CI)2 . | Ratio3 (mean ± SE) . | ||
Control | No-injection | 0.0033 ± 0.0002a | 1 | 0.005 ± 0.001a | 1 | 0.028 ± 0.009a | 1 |
GFP-injection | 0.0032 ± 0.0005a | 0.97 ± 0.01 | 0.004 ± 0.001a | 0.80 ± 0.09 | 0.031 ± 0.016a | 1.11 ± 0.21 | |
GPCR020021 | GPCR020021- injection | 0.0020 ± 0.0001b | 0.61 ± 0.02 | 0.003 ± 0.0005b | 0.60 ± 0.10 | 0.019 ± 0.001b | 0.67 ± 0.02 |
Gαs006458 | Gαs006458- injection | 0.0013 ± 0.0005b | 0.39 ± 0.07 | 0.002 ± 0.0004b | 0.41 ± 0.04 | 0.005 ± 0.001b | 0.17 ± 0.05 |
AC007240 | AC007240-injection | 0.0012 ± 0.0004b | 0.36 ± 0.06 | 0.002 ± 0.0003b | 0.40 ± 0.04 | 0.011 ± 0.007b | 0.36 ± 0.04 |
AC004739 | AC004739-injection | 0.0009 ± 0.0002b | 0.27 ± 0.03 | 0.002 ± 0.0005b | 0.40 ± 0.05 | 0.010 ± 0.005b | 0.36 ± 0.05 |
PKA000798 | PKA000798- injection | 0.0019 ± 0.0010b | 0.58 ± 0.16 | 0.001 ± 0.0004b | 0.24 ± 0.05 | 0.012 ± 0.003b | 0.43 ± 0.06 |
PKA018257 | PKA018257- injection | 0.0014 ± 0.0006b | 0.42 ± 0.08 | 0.002 ± 0.0007b | 0.37 ± 0.06 | 0.014 ± 0.003b | 0.51 ± 0.06 |
Target gene . | RNAi . | S-Lab . | HAmCqG0 . | MAmCqG0 . | |||
---|---|---|---|---|---|---|---|
LC501 (CI)2 . | Ratio3 (mean ± SE) . | LC501(CI)2 . | Ratio3 (mean ± SE) . | LC501 (CI)2 . | Ratio3 (mean ± SE) . | ||
Control | No-injection | 0.0033 ± 0.0002a | 1 | 0.005 ± 0.001a | 1 | 0.028 ± 0.009a | 1 |
GFP-injection | 0.0032 ± 0.0005a | 0.97 ± 0.01 | 0.004 ± 0.001a | 0.80 ± 0.09 | 0.031 ± 0.016a | 1.11 ± 0.21 | |
GPCR020021 | GPCR020021- injection | 0.0020 ± 0.0001b | 0.61 ± 0.02 | 0.003 ± 0.0005b | 0.60 ± 0.10 | 0.019 ± 0.001b | 0.67 ± 0.02 |
Gαs006458 | Gαs006458- injection | 0.0013 ± 0.0005b | 0.39 ± 0.07 | 0.002 ± 0.0004b | 0.41 ± 0.04 | 0.005 ± 0.001b | 0.17 ± 0.05 |
AC007240 | AC007240-injection | 0.0012 ± 0.0004b | 0.36 ± 0.06 | 0.002 ± 0.0003b | 0.40 ± 0.04 | 0.011 ± 0.007b | 0.36 ± 0.04 |
AC004739 | AC004739-injection | 0.0009 ± 0.0002b | 0.27 ± 0.03 | 0.002 ± 0.0005b | 0.40 ± 0.05 | 0.010 ± 0.005b | 0.36 ± 0.05 |
PKA000798 | PKA000798- injection | 0.0019 ± 0.0010b | 0.58 ± 0.16 | 0.001 ± 0.0004b | 0.24 ± 0.05 | 0.012 ± 0.003b | 0.43 ± 0.06 |
PKA018257 | PKA018257- injection | 0.0014 ± 0.0006b | 0.42 ± 0.08 | 0.002 ± 0.0007b | 0.37 ± 0.06 | 0.014 ± 0.003b | 0.51 ± 0.06 |
1LC50 values in ppm
295% CI, toxicity of insecticide is considered significantly different when the 95% CI fail to overlap; no significant difference (a) and significant difference (b) compared with no-injection mosquitoes.
3Ratios were calculated by dividing the LC50 of the GFP or target gene injection mosquito groups by the LC50 of the non-injection mosquito group of same mosquito strain.
Insecticide resistance in mosquitoes microinjected with RNAi to silence specific genes
Target gene . | RNAi . | S-Lab . | HAmCqG0 . | MAmCqG0 . | |||
---|---|---|---|---|---|---|---|
LC501 (CI)2 . | Ratio3 (mean ± SE) . | LC501(CI)2 . | Ratio3 (mean ± SE) . | LC501 (CI)2 . | Ratio3 (mean ± SE) . | ||
Control | No-injection | 0.0033 ± 0.0002a | 1 | 0.005 ± 0.001a | 1 | 0.028 ± 0.009a | 1 |
GFP-injection | 0.0032 ± 0.0005a | 0.97 ± 0.01 | 0.004 ± 0.001a | 0.80 ± 0.09 | 0.031 ± 0.016a | 1.11 ± 0.21 | |
GPCR020021 | GPCR020021- injection | 0.0020 ± 0.0001b | 0.61 ± 0.02 | 0.003 ± 0.0005b | 0.60 ± 0.10 | 0.019 ± 0.001b | 0.67 ± 0.02 |
Gαs006458 | Gαs006458- injection | 0.0013 ± 0.0005b | 0.39 ± 0.07 | 0.002 ± 0.0004b | 0.41 ± 0.04 | 0.005 ± 0.001b | 0.17 ± 0.05 |
AC007240 | AC007240-injection | 0.0012 ± 0.0004b | 0.36 ± 0.06 | 0.002 ± 0.0003b | 0.40 ± 0.04 | 0.011 ± 0.007b | 0.36 ± 0.04 |
AC004739 | AC004739-injection | 0.0009 ± 0.0002b | 0.27 ± 0.03 | 0.002 ± 0.0005b | 0.40 ± 0.05 | 0.010 ± 0.005b | 0.36 ± 0.05 |
PKA000798 | PKA000798- injection | 0.0019 ± 0.0010b | 0.58 ± 0.16 | 0.001 ± 0.0004b | 0.24 ± 0.05 | 0.012 ± 0.003b | 0.43 ± 0.06 |
PKA018257 | PKA018257- injection | 0.0014 ± 0.0006b | 0.42 ± 0.08 | 0.002 ± 0.0007b | 0.37 ± 0.06 | 0.014 ± 0.003b | 0.51 ± 0.06 |
Target gene . | RNAi . | S-Lab . | HAmCqG0 . | MAmCqG0 . | |||
---|---|---|---|---|---|---|---|
LC501 (CI)2 . | Ratio3 (mean ± SE) . | LC501(CI)2 . | Ratio3 (mean ± SE) . | LC501 (CI)2 . | Ratio3 (mean ± SE) . | ||
Control | No-injection | 0.0033 ± 0.0002a | 1 | 0.005 ± 0.001a | 1 | 0.028 ± 0.009a | 1 |
GFP-injection | 0.0032 ± 0.0005a | 0.97 ± 0.01 | 0.004 ± 0.001a | 0.80 ± 0.09 | 0.031 ± 0.016a | 1.11 ± 0.21 | |
GPCR020021 | GPCR020021- injection | 0.0020 ± 0.0001b | 0.61 ± 0.02 | 0.003 ± 0.0005b | 0.60 ± 0.10 | 0.019 ± 0.001b | 0.67 ± 0.02 |
Gαs006458 | Gαs006458- injection | 0.0013 ± 0.0005b | 0.39 ± 0.07 | 0.002 ± 0.0004b | 0.41 ± 0.04 | 0.005 ± 0.001b | 0.17 ± 0.05 |
AC007240 | AC007240-injection | 0.0012 ± 0.0004b | 0.36 ± 0.06 | 0.002 ± 0.0003b | 0.40 ± 0.04 | 0.011 ± 0.007b | 0.36 ± 0.04 |
AC004739 | AC004739-injection | 0.0009 ± 0.0002b | 0.27 ± 0.03 | 0.002 ± 0.0005b | 0.40 ± 0.05 | 0.010 ± 0.005b | 0.36 ± 0.05 |
PKA000798 | PKA000798- injection | 0.0019 ± 0.0010b | 0.58 ± 0.16 | 0.001 ± 0.0004b | 0.24 ± 0.05 | 0.012 ± 0.003b | 0.43 ± 0.06 |
PKA018257 | PKA018257- injection | 0.0014 ± 0.0006b | 0.42 ± 0.08 | 0.002 ± 0.0007b | 0.37 ± 0.06 | 0.014 ± 0.003b | 0.51 ± 0.06 |
1LC50 values in ppm
295% CI, toxicity of insecticide is considered significantly different when the 95% CI fail to overlap; no significant difference (a) and significant difference (b) compared with no-injection mosquitoes.
3Ratios were calculated by dividing the LC50 of the GFP or target gene injection mosquito groups by the LC50 of the non-injection mosquito group of same mosquito strain.
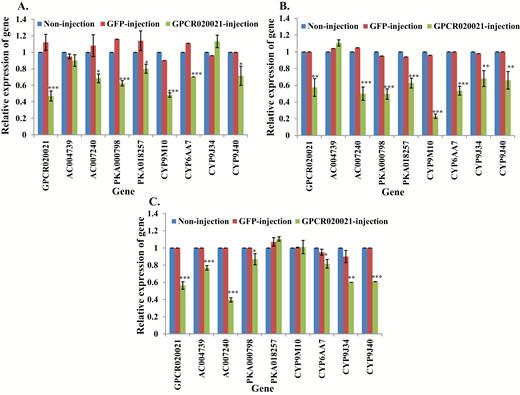
Relative expression of genes in GPCR020021 knockdown mosquitoes. mRNA levels of different genes (GPCR020021, AC004739, -007240, PKA000798, -018257, CYP9M10, CYP6AA7, CYP9J34, CYP9J40) were quantitatively tested by qRT-PCR at 3 d after dsRNA of GPCR020021 injection (green column), GFP-injected (red column), or non-injection mosquitoes (blue column). (A) Relative expression of genes following GPCR020021 knockdown in S-Lab strain. (B) Relative expression of genes following GPCR020021 knockdown in HAmCqG0 strain. (C) Relative expression of genes following GPCR020021 knockdown in MAmCqG0 strain. The results are shown as the mean ± SE (n ≥ 3). Statistical significance is represented by P ≤ 0.05 in the level of the gene expression among the gene-injected, GFP-injected and no-injected mosquitoes with *P < 0.05, **P < 0.01, ***P < 0.001.
As an essential component in the GPCR regulation pathway, the particular function of Gαs in insecticide resistance was investigated. The expression of Gαs006458 was suppressed by RNAi injection, causing increased susceptibility of S-Lab larvae to permethrin (Table 2). Following Gαs006458 knockdown, its downstream respective genes (2ACs, 2PKAs) and 4 P450 genes showed significantly decreased expression by comparison with that seen in noninjected and GFP-injected S-Lab mosquitoes (Fig. 2A, Supp Table 2 [online only]). Knockdown of Gαs006458 gene in the HAmCqG0 and MAmCqG0 strains produced a decrease of resistance to permethrin in both strains (Table 2). Knockdown of Gαs006458 also decreased the expression of downstream respective genes tested, except for AC007240 and PKA000798 in HAmCqG0, which showed no significant changes compared with non-Gαs006458-injected mosquitoes (Fig. 2B and C, Supp Table 2 [online only]).
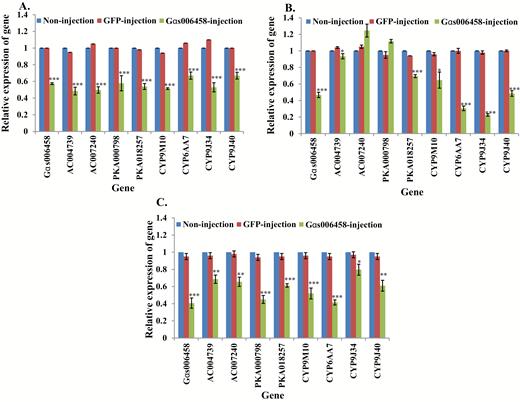
Relative expression of genes in Gαs006458 knockdown mosquitoes. mRNA levels of different genes (Gαs006458, AC004739, -007240, PKA000798, -018257, CYP9M10, CYP6AA7, CYP9J34, CYP9J40) were quantitatively tested by qRT-PCR at 3 d after dsRNA of Gαs006458 injection (green column), GFP-injected (red column), or non-injection mosquitoes (blue column). (A) Relative expression of genes following Gαs006458 knockdown in S-Lab strain. (B) Relative expression of genes following Gαs006458 knockdown in HAmCqG0 strain. (C) Relative expression of genes following Gαs006458 knockdown in MAmCqG0 strain. The results are shown as the mean ± SE (n ≥ 3). Statistical significance is represented by P ≤ 0.05 in the level of the gene expression among the gene-injected, GFP-injected and no-injected mosquitoes with *P < 0.05, **P < 0.01, ***P < 0.001.
The Function of the Adenylate Cyclase in the S-Lab and Field Strains
To identify the critical role of two ACs (AC004739 and AC007240) in the insecticide sensitivity of S-Lab and HAmCqG0 and MAmCqG0, we tested the function of AC004739 and AC007240 individually by RNAi. Microinjection of dsRNA-AC007240 in S-Lab increased susceptibility of S-Lab larvae to permethrin (Table 2), as well as significantly decreased the expression of its downstream respective genes-PKA000798, -018257, and 4 CYPs in comparison with controls (Fig. 3A, Supp Table 3 [online only]). Similar results were detected in HAmCqG0 and MAmCqG0, showing knockdown of AC007240 genes was associated with decreased resistance of HAmCqG0 and MAmCqG0 to permethrin (Table 2), as well as causing significantly decreased expression of downstream PKA and CYP genes (Fig. 3B and C, Supp Table 3 [online only]).
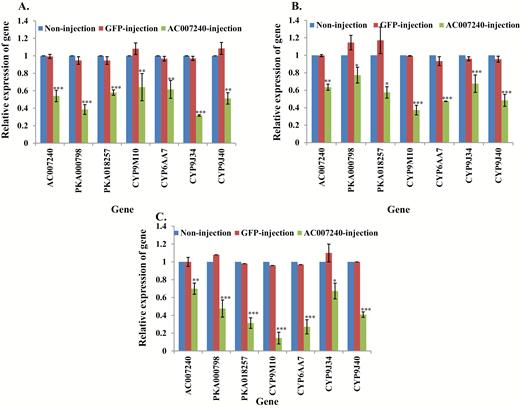
Relative expression of genes in AC007240 knockdown mosquitoes. mRNA levels of different genes (AC007240, PKA000798, -018257, CYP9M10, CYP6AA7, CYP9J34, CYP9J40) were quantitatively tested by qRT-PCR at 3 d after dsRNA of AC007240 injection (green column), GFP-injected (red column), or non-injection mosquitoes (blue column). (A) Relative expression of genes following AC007240 knockdown in S-Lab strain. (B) Relative expression of genes following AC007240 knockdown in HAmCqG0 strain. (C) Relative expression of genes following AC007240 knockdown in MAmCqG0 strain. The results are shown as the mean ± SE (n ≥ 3). Statistical significance is represented by P ≤ 0.05 in the level of the gene expression among the gene-injected, GFP-injected and no-injected mosquitoes with *P < 0.05, **P < 0.01, ***P < 0.001.
Microinjection with dsRNA of AC004739 also was conducted in these three strains. Knockdown of AC004739 gene in S-Lab resulted in an increase of susceptibility to permethrin (Table 2) and a decrease of downstream PKA and CYP genes by comparison with controls (Fig. 4A, Supp Table 4 [online only]). Similar results were identified in strain MAmCqG0, in which suppression of AC004739 caused decreased resistance to permethrin (Table 2) and the expression of PKA and CYP genes (Fig. 4C, Supp Table 4 [online only]). In the HAmCqG0 strain knockdown of the AC004739 gene also was associated with decreased resistance to permethrin (Table 2) and downstream genes; however, no significant changes were produced in the expression of PKA018257, CYP9J34, and CYP9J40 (Fig. 4B, Supp Table 4 [online only]).
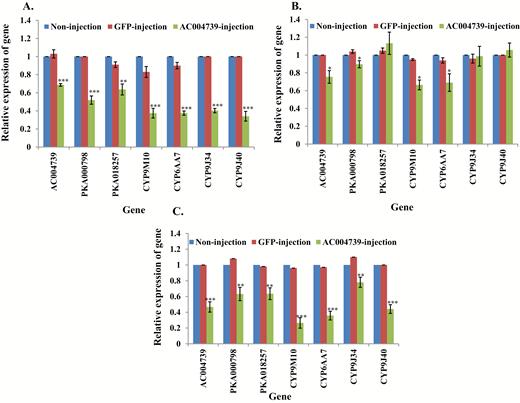
Relative expression of genes in AC004739 knockdown mosquitoes. mRNA levels of different genes (AC004739, PKA000798, -018257, CYP9M10, CYP6AA7, CYP9J34, CYP9J40) were quantitatively tested by qRT-PCR at 3 d after dsRNA of AC004739 injection (green column), GFP-injected (red column), or noninjection mosquitoes (blue column). (A) Relative expression of genes following AC004739 knockdown in S-Lab strain. (B) Relative expression of genes following AC004739 knockdown in HAmCqG0 strain. (C) Relative expression of genes following AC004739 knockdown in MAmCqG0 strain. The results are shown as the mean ± SE (n ≥ 3). Statistical significance is represented by P ≤ 0.05 in the level of the gene expression among the gene-injected, GFP-injected and no-injected mosquitoes with *P < 0.05, **P < 0.01, ***P < 0.001.
The Function of the Protein Kinase A in the S-Lab and HAmCqG0 and MAmCqG0
The important involvement of two PKA genes (PKA018257 and PKA000798) in insecticide resistance was investigated in susceptible and field strains. The expression of one PKA gene (PKA000798) was suppressed by RNAi in S-Lab, HAmCqG0, and MAmCqG0, causing either increased susceptibility of S-Lab to permethrin (Table 2) or decreased resistance to permethrin in HAmCqG0 and MAmCqG0 (Table 2). The expression of its downstream respective four P450 genes were all significantly decreased following PKA000798 knockdown in all three strains (Fig. 5A–C, Supp Table 5 [online only]).
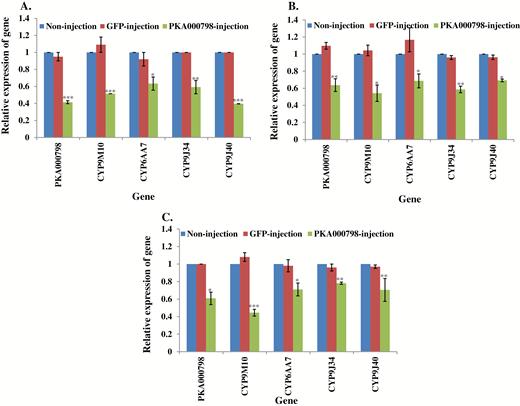
Relative expression of genes in PKA000798 knockdown mosquitoes. mRNA levels of different genes (PKA000798, CYP9M10, CYP6AA7, CYP9J34, CYP9J40) were quantitatively tested by qRT-PCR at 3 d after dsRNA of PKA000798 injection (green column), GFP-injected (red column), or noninjection mosquitoes (blue column). (A) Relative expression of genes following PKA000798 knockdown in S-Lab strain. (B) Relative expression of genes following PKA000798 knockdown in HAmCqG0 strain. (C) Relative expression of genes following PKA000798 knockdown in MAmCqG0 strain. The results are shown as the mean ± SE (n ≥ 3). Statistical significance is represented by P ≤ 0.05 in the level of the gene expression among the gene-injected, GFP-injected and no-injected mosquitoes with *P < 0.05, **P < 0.01, ***P < 0.001.
For the functional study of the PKA018257 gene, the same approach as in PKA00798 knockdown was conducted in all three strains. The results showed knockdown of PKA018257 gene caused either increased susceptibility of S-Lab to permethrin (Table 2) or decreased resistance of field mosquito strains to permethrin (Table 2) by comparison with controls. The expression of downstream respective CYPs all showed a reduction following PKA018257 knockdown (Fig. 6A–6C, Supp Table 6 [online only]).
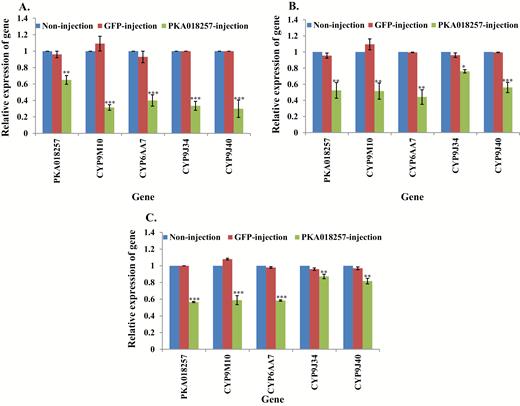
Relative expression of genes in PKA018257 knockdown mosquitoes. mRNA levels of different genes (PKA018257, CYP9M10, CYP6AA7, CYP9J34, CYP9J40) were quantitatively tested by qRT-PCR at 3 d after dsRNA of PKA018257 injection (green column), GFP-injected (red column), or noninjection mosquitoes (blue column). (A) Relative expression of genes following PKA018257 knockdown in S-Lab strain. (B) Relative expression of genes following PKA018257 knockdown in HAmCqG0 strain. (C) Relative expression of genes following PKA018257 knockdown in MAmCqG0 strain. The results are shown as the mean ± SE (n ≥ 3). Statistical significance is represented by P ≤ 0.05 in the level of the gene expression among the gene-injected, GFP-injected and no-injected mosquitoes with *P < 0.05, **P < 0.01, ***P < 0.001.
Discussion
GPCR-mediated physiological pathways have been identified as being responsible for hormones, neurotransmitters, and environmental stimulates in a number of different organisms (Vassilatis et al. 2003, Rosenbaum et al. 2009). The activation of the G-proteins involved in the GPCR regulatory pathway is triggered by GPCRs to regulate downstream intracellular cascades (Whorton et al. 2007). GPCR-Gαs is engaged in the activation of AC and is consequently also involved in PKA activity as it is necessary for converting the ATP into cyclic AMP (Bauman et al. 2006; Hur and Kim 2002; Agarwal et al. 2009) . Previous studies by Li et al. (2014, 2015) and Li and Liu (2017) have pinpointed a GPCR-regulatory pathway in laboratory selected insecticide resistant Cx. quinquefasciatus. In these studies, knockdown of the expression of GPCR, Gαs, AC, PKA, and P450 genes resulted in decreases in both permethrin resistance and the expression of their downstream respective genes in two permethrin selected resistant mosquito strains, HAmCqG8 and MAmCqG6, highlighting the critical roles of each effector in this intracellular pathway (Li et al. 2015, Li and Liu 2017). However, the function of this GPCR regulation pathway in insecticide susceptible and field strains has yet identified. In the current study, we built on this earlier work in an attempt to understand the general function and inherited characteristics of this GPCR pathway in an insecticide susceptible strain (S-Lab) and two field strains HAmCqG0 and MAmCqG0 (Liu et al. 2004), which were the parental strains of HAmCqG8 and MAmCqG6, respectively, without insecticide selection in laboratory after collection. Similar functions of each effector were found in the S-Lab, HAmCqG0, and MAmCqG0, showing their important involvement in permethrin sensitivity. Taken together with the findings of the previous studies (Li et al. 2015, Li and Liu 2017), our results confirmed the general function of this GPCR regulation pathway in the regulation of insecticide susceptibility/resistance in mosquitoes.
Although gene expression suppression of all effectors obviously caused decreased resistance or increased susceptibility to permethrin in mosquito strains, the unique involvement of each effector in this GPCR-regulation pathway were identified by comparing the regulatory role on the expression changes of downstream respective genes among different Cx. quinquefasciatus strains. Knockdown of GPCR020021 and Gαs006458 genes showed dynamic changes of different AC genes in S-Lab and HAmCqG0 mosquitoes implying a possibility of other GPCR or G-protein subunits involved in the GPCR regulation pathway in resistance development, as well as the differences of AC gene expression in GPCR020021 and Gαs006458 knockdown of highly resistant (HAmCqG8) and field (HAmCqG0) strains provided a new information for the evolutionary development of insecticide resistance that is associated with multiple GPCR-regulation pathways responding to insecticide selection (Li and Liu 2017). Knockdown of different AC genes caused expression changes of PKA genes indicating a strong collaboration between these ACs and PKAs, which are able to work together to regulate resistance-related P450 gene expression, consequently engaging in resistance development in Cx. quinquefasciatus mosquitoes.
In summary, the universal roles and inherited characteristics of each effector in the development of permethrin resistance and the regulation of downstream gene expression clearly indicated the importance of this GPCR-regulation pathway in mosquitoes. The specific function of each effector demonstrated the collaborative approach among the effectors across the whole GPCR regulation pathway. In spite of variations in the effectors involved in the GPCR regulatory pathway in mosquito strains, the GPCR/Gαs/AC/PKA/P450 mediated permethrin resistance represents a very similar function across the susceptible, field, and highly resistant mosquito strains, indicating the universal function of this intracellular pathway and the complementary interactions among these effectors in the development of permethrin resistance in Cx. quinquefasciatus.
Supplementary Data
Supplementary data are available at Journal of Medical Entomology online.
Acknowledgments
This study was supported by an NIH grant (1R21AI076893) to N.L.; AAES Hatch/Multistate Grant ALA08-045 to N.L..; ALA015-1-10026 to N.L.; and AU Intramural Grant (AU-IPG) to N.L.