-
PDF
- Split View
-
Views
-
Cite
Cite
J. A. Bisset, R. Marín, M. M. Rodríguez, D. W. Severson, Y. Ricardo, L. French, M. Díaz, O. Pérez, Insecticide Resistance in Two Aedes aegypti (Diptera: Culicidae) Strains From Costa Rica, Journal of Medical Entomology, Volume 50, Issue 2, 1 March 2013, Pages 352–361, https://doi.org/10.1603/ME12064
- Share Icon Share
Abstract
Dengue (family Flaviridae, genus Flavivirus, DENV) and dengue hemorrhagic fever (DHF) are presently important public health problems in Costa Rica. The primary strategy for disease control is based on reducing population densities of the main mosquito vector Aedes aegypti (L.) (Diptera: Culicidae). This is heavily dependent on use of chemical insecticides, thus the development of resistance is a frequent threat to control program effectiveness. The objective of this study was to determine the levels of insecticide resistance and the metabolic resistance mechanisms involved in two Ae. aegypti strains collected from two provinces (Puntarenas and Limon) in Costa Rica. Bioassays with larvae were performed according to World Health Organization guidelines and resistance in adults was measured through standard bottle assays. The activities of β-esterases, cytochrome P450 monooxygenases, and glutathione S-transferases (GST), were assayed through synergists and biochemical tests, wherein the threshold criteria for each enzyme was established using the susceptible Rockefeller strain. The results showed higher resistance levels to the organophosphate (OP) temephos and the pyrethroid deltamethrin in larvae. The efficacy of commercial formulations of temephos in controlling Ae. aegypti populations was 100% mortality up to 11 and 12 d posttreatment with daily water replacements in test containers. Temephos and deltamethrin resistance in larvae were associated with high esterase activity, but not to cytochrome P450 monooxygenase or GST activities. Adult mosquitoes were resistant to deltamethrin, and susceptible to bendiocarb, chlorpyrifos, and cypermethrin. Because temephos and deltamethrin resistance are emerging at the studied sites, alternative insecticides should be considered. The insecticides chlorpyrifos and cypermethrin could be good candidates to use as alternatives for Ae. aegypti control.
In Latin America, dengue (family Flaviridae, genus Flavivirus, DENV) and dengue hemorrhagic fever (DHF) represent increasing challenges despite advances in health care and prevention systems (San Martin et al. 2010). In Costa Rica, dengue fever (DF) is one of the most prevalent diseases transmitted by mosquitoes, mainly affecting the regions of the North Pacific, Atlantic, and Central Pacific. Its main vector, Aedes aegypti (L.) 1762, was eradicated from the country in 1960, but frequent reinfestations occurred during the years 1970 and 1980. After vector reintroduction, the transmission of DF was reported in 1993 in Puntarenas and Liberia, extending later to other regions of Costa Rica (WHO 1994). Since then, DF has remained endemic in the provinces of Puntarenas and Limon (Troyo et al. 2009).
In general, during periods of high vector populations or the occurrence of DF outbreaks or epidemics, the use of chemical insecticides is a standard practice because of their effectiveness in reducing larval and adult populations. The most common insecticides used for control of Ae. aegypti in Costa Rica over the last 10 yr are the larvicide temephos and adulticide deltamethrin in its formulation as Super K-Othrine Solfac. However, the continued effectiveness of insecticides for controlling mosquito populations is threatened because of strong selection pressure for insecticide resistance. Previous studies on several Latin American Ae. aegypti strains, including populations from Costa Rica, have revealed the existence of resistance mechanisms to both temephos and pyrethroids (Rodríguez et al. 2007, Saavedra et al. 2007).
As DF and DHF are significant public health problems in Costa Rica, and vector control remains the primary strategy to avoid or prevent the transmission of this disease, it is necessary to conduct research on the emergence of insecticide resistance. These results are critical to development of effective biomarkers for resistance that can be used to facilitate better insecticide use and achieve effective vector control with insecticides currently available for use in public health programs. The aim of this study was to measure the levels of insecticide resistance and the mechanisms involved in Ae. aegypti populations from Costa Rica.
Materials and Methods
The following Ae. aegypti strains were used:
Laboratory Strains
Rockefeller.
is an insecticide susceptible strain of Caribbean origin colonized during the 1930s and was provided by the Center for Disease Control and Prevention laboratory in San Juan, Puerto Rico.
SANtem-F11.
is a temephos-resistant strain colonized in 1997 from Santiago de Cuba and subjected to selection pressure with this insecticide for 11 generations. This strain is routinely maintained in the laboratory under selection pressure by using the LC90 (90% lethal concentration) of temephos.
SAN-F14.
is a deltamethrin-resistant strain colonized in 1997 from Santiago de Cuba and subjected to selection pressure with this insecticide for 14 generations. This strain is routinely maintained in the laboratory under selection pressure by using the LC90 (90% lethal concentration) of deltamethrin.
Field Strains
Puntarenas.
One strain was colonized from the Herradura locality (Fig. 1), Jaco District, and Garabito canton, Puntarenas Province is located in the western part of Costa Rica (09° 56′55″ N, 84° 58′24″ W) and has an area of 11.266 km2. The human population is estimated at 358,137; it has a population density of 32 inhabitants per km2. It is divided into 11 cantons and 43 districts.
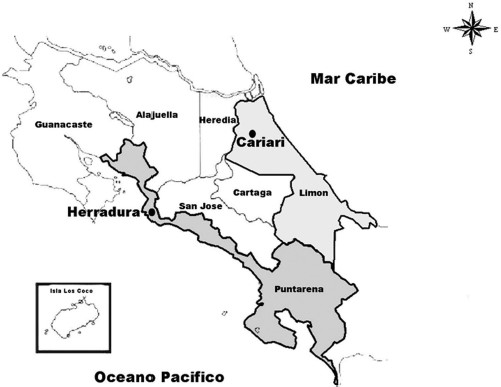
Provinces in Costa Rica, where Ae. aegypti strains were collected. Herradura locality, Jaco District, and Garabito canton (Puntarenas Province). Cariari locality, Cariari District, Pococí canton (Limon Province).
Limon.
One strain was colonized from the Cariari locality (Fig. 1), Cariari District, Pococí canton, Limon Province (10° 00′29″ N, 83° 15′06″ W), located in the eastern side of the country and has an area of 9,188 km2. The human population is estimated at 432,923, with a population density of 80 inhabitants per km2. It is divided in 6 cantons and 27 districts.
Egg papers collected from the two localities were transferred to insectaries at the Institute of Tropical Medicine, Pedro Kouri, Cuba and reared to adults. Individuals form the F1 or F2 generations were used for all the assays.
Insecticides
Temephos.
o-dimethyl phosphorothioate odiéster with 4.4 'tiodifenol, with 93.3% purity was provided by BASF AG, Ludwigshafen, Germany. The temephos granulate formulation (BIOLARV G1) was acquired from BIOQUIM Centroamericana SA, San José, Costa Rica.
Bendiocarb.
2,2-dimethyl-1, 3-benzodioxol-4-yl methylcarbamate; 98% purity; supplied by Bayer SA (Heredia, Costa Rica).
Fenitrothion.
O,O-Dimethyl O-(3-methyl-4-nitrophenyl) phosphorothioate; 97% supplied by Bayer SA (Costa Rica).
Deltamethrin.
(S)-α-cyano-3-phenoxybenzyl (1R, 3R) −3 - (2, 2-dibromovinyl) −2,2-dimethylcyclopropane carboxylate; with 96.8% purity; supplied by Roussel Uclaf (Romainville, France).
Cypermethrin.
(1RS)-cis, trans-3-(2,2-dichlorovinyl) −2,2-dimethylcyclopropanecarboxylate (RS)-cyano-3-phenoxybenzyl (IUPAC); with 90.5% purity; supplied by Chemotécnica SA (Buenos Aires, Argentina).
Chlorpyrifos.
O, O-diethyl O-(3, 5, 6-trichloro-2-pyridyl) phosphorothioate; with 94% purity; supplied by supplied by Chemotécnica SA (Buenos Aires, Argentina).
Larval Bioassay.
Standard bioassays were used to establish complete dosage–mortality lines (WHO 1981). Twenty early fourth-instar larvae of uniform size were placed in plastic cups containing 99 ml of tap water and 1 ml of insecticide solution. Five or more concentrations of each insecticide, prepared in standard (wt:vol) acetone solution, were tested independently on different days. Controls were treated with 1 ml of acetone. All mosquitoes were reared and maintained at 27 ± 1°C, 70–80% RH, and a photoperiod of 12:12 (L:D) h. Mortality was determined after 24 h of insecticide exposure. Each bioassay was replicated at least twice (i.e., 1,000 larvae were assayed for each insecticide). Probit analysis (Finney 1971) was used to determine the lethal concentrations that induced 50% mortality (LC50) as described elsewhere (Raymond 1985). Resistance ratios (RR50) were calculated by LC50 rates in Ae. aegypti populations from each field strain compared with those of the susceptible Rockefeller strain.
RR50 = LC50 studied population/LC50 susceptible reference strain.
Values of resistance ratios (<fivefold) were diagnosed as low resistance, medium, (between five- and 10-fold), and high resistance as >10-fold (Mazzarri and Georghiou 1995).
Effectiveness of Temephos Granulate Formulation.
Larval bioassays were performed using BIOLARV G1. One gram of this formulation was added to 10 liters of water (1 ppm) and left for 24 h. Fifty early fourth-instar larvae of uniform size were added to five containers (4 replicates and 1 control) and mortality was recorded after 24 h of exposure. Every 24 h, all the water was removed, replaced, and a fresh set of 50 larvae added. Hence, the only temephos remaining was the residual temephos adhering to the container from the initial treatment. This was repeated every day. The experiment was designed to reflect the field context in which water containers are frequently and completely emptied and refilled on a daily basis. The experiment was replicated twice for each strain on different days for a total of 8 test containers and 2 controls per strain.
Synergist Bioassay.
Similar procedures for larval bioassays were followed (WHO 1981), except that 0.5 ml of the required concentration of each synergist solution in acetone was applied to each bioassay 4 h before the application of the desired concentration of insecticide. The total volume of acetone per bioassay did not exceed 1 ml. Synergists were used at sublethal concentrations corresponding to 0.008 mg/liter of piperonyl butoxide (PBO) and 5.0 mg/liter of triphenyl phosphate (TPP) and ethacrinic acid (EA). At these concentrations there was no mortality with the synergist alone.
Biochemical Assays.
Individual fourth-instar larvae were homogenized in 200 μl of 0.01-M sodium phosphate buffer (pH 7.5). Three replicates of 20 μl of crude homogenate were transferred separately to a fresh microtitre plate with a 12-channel multipipette for testing esterase, glutathione S-transferase (GST), and cytochrome P450 monooxygenase activities, and two replicates of 10 μl were added to another plate for protein assays. Each microtitre plate was used to measure 29 larvae of each of the field strains, 18 larvae of the Rockefeller strain, as well as 3 positive and negative controls per plate. The absorbance level of the negative control was subtracted from the value for each mosquito for each enzyme, thus eliminating the reaction background. The Rockefeller strain was used as a susceptible reference population in all of the biochemical analyses performed.
Esterase Activity.
Esterase activity was determined by using the methods of Peiris and Hemingway (1990), with some modification to detect esterase activity in Ae. aegypti (Rodríguez et al. 2001); 200 μl of 0.7-mm β-naphthyl acetate substrate was added to each microtitre plate well. The enzyme reaction was left for 10 min at room temperature before 40 μl of Fast-blue B salt (0.3% in 3.5% sodium dodecyl sulfate) (Sigma Aldrich, St. Louis, MO) was added to stop the reaction. Absorbance was read at 570 nm in a VersaMax microplate reader (Molecular Devices Corporation, Sunnyvale, CA). Esterase activity was expressed as μmol β- naphthol per minute per milligram protein.
Cytochrome P450 Monooxygenases.
Assays were conducted using methods as described elsewhere (Brogdon et al. 1997). Briefly, 20 mg of 3, 3, 5, 5-tetramethylbenzidine dihydrochloride (TMBZ) was diluted in 25 ml of methanol and then mixed with 75 ml of 0.25-M sodium acetate (pH 5.0); 200 μl of the TMBZ solution then was added to each microtitre plate well, followed by 25 μl of 3% hydrogen peroxide. The plate was incubated for 5 min and read at 620 nm. Cytochrome P450 monooxygenase activity was expressed as nmol cytochrome c per minute per milligram protein.
Glutathione S-Transferase Activity.
Glutathione S-transferase activity was determined using a modification of the method of Booth et al. (1961). Briefly, 20 μl of larval homogenate was added to each microtitre plate well followed by 200 μl of reaction mixture (250 ml of 50-mM 1-chloro-2, 4-dinitrobenzene [CDNB], and 5 ml of 20-mM of reduced glutathione [GSH]). After 3 min, the optical density (O.D.) was read at 340 nm. GST activity was expressed as μmol CDNB per minute per milligram protein.
Protein Concentration.
Assays were conducted using previously described methods (Brogdon 1984); 80 μl of sodium phosphate buffer (NaPO4) was added to each microtitre plate well followed by 200 μl of Bio-Rad (Hercules, CA) solution (diluted 1:4 in distilled water), and reactions were read immediately at 620 nm. Values of protein concentration in milligrams per meter for individual mosquitoes were calculated from a standard curve of known concentrations of bovine serum albumin.
Data Analyses.
Enzymatic activities for each strain were compared using one-way analysis of variance (ANOVA). Differences were considered significant at P < 0.05. Tukey's test was conducted after significant differences had been identified by ANOVA to establish which means differed from one another. All statistical tests were carried out using Statistica 6.0 (Statsoft Inc., Tulsa, OK).
Estimates of resistance gene frequencies for esterase, cytochrome P450 monooxygenase, and GST activity resistance mechanisms were calculated from the number of homozygous susceptible individuals for each assay, assuming that each population was in Hardy–Weinberg equilibrium. The range of esterase activity was established for 100 larvae of the Rockefeller strain. The mean ± standard deviation (SD) value for specific esterase activity was 0.547 ± 0.143. Values above 0.984-μmol β-naphthol acetate/min/mg protein (mean + 3SD) were considered as elevated esterase activity and defined larvae as resistant. The range of cytochrome P450 monooxygenase activity was established for 135 larvae of the Rockefeller strain. The mean ± SD value for cytochrome P450 monooxygenase activity was 0.758 ± 0.408. Values above 1.98 nmol cytochrome c/min/mg protein (mean + 3SD) were considered as elevated monooxygenase activity and defined larvae as resistant. The mean ± SD value for GST activity was 189.39 ± 14.65. Values above 233.34-μmol CDNB /min/mg protein (mean + 3SD) were considered as elevated GST activity and defined larvae as resistant.
Electrophoresis.
Individual larval from field strains from Costa Rica and the susceptible and resistant reference strains were homogenized in 200 μl of 0.01-M sodium phosphate buffer (pH 7.5). Individual homogenates were subjected to 10% polyacrylamide gel electrophoresis (PAGE). Electrophoresis was performed in Trisborate/EDTA 0.1-M (pH 8.0) buffer. The gels were run at 150 V. Esterases were visualized by adding 4 ml of α-naphthyl acetate (70 mM), 4 ml of β-naphthyl acetate (70 mM), and 10 ml of Fast-blue solution (0.03 g Fast-blue salt, 3-ml distilled water, and 7-ml 5% sodium dodecyl sulfate in 50 ml of phosphate buffer 0.1 M at pH 7.5). Enzyme activity was stopped by replacing the staining medium with 7% acetic acid.
Adult Bioassays.
Bioassays were conducted by using the methods of Brogdon and McAllister (1998). Each population was measured against the diagnostic dosage (DD, the lowest dose that caused 100% mortality in 30 min of exposure) determined previously for the Rockefeller strain for each insecticide (M.M.R., unpublished data): chlorpyrifos (90 μg/bottle), cypermethrin (13.5 μg/bottle), and deltamethrin (6.5 μg/bottle). In total, 20–25 nonblood-fed females from each site were introduced into 250-ml glass bottles (Wheel Brand, Shanghai, China) coated with the diagnostic dose of each insecticide. Each test consisted of 4 treatment bottles and 1 control bottle. At least three replicates were conducted for each insecticide and mosquito population. All insecticide solutions were prepared fresh from stock solutions immediately before use. Every 5 min, the numbers of dead mosquitoes in each bottle were recorded. This process was repeated for 1 h or until all of the mosquitoes were dead. The mortality criteria included mosquitoes with difficulty flying or standing on the bottle's surface. Mosquitoes that survived the appropriate DD for each insecticide were considered to be resistant (Brogdon and McAllister 1998).
Results
Larval Bioassays.
Resistance ratios at the LC50 level showed that both strains collected from Costa Rica displayed high temephos resistance (resistance ratio [RR50] > 10X). The highest level of resistance was observed for the pyrethroid deltamethrin in both strains, Limon (81.48X) and Puntarenas (59.25X). Low resistance ([RR50] < 10X) was observed for the OP fenitrothion, the carbamate bendiocarb, and the pyrethroid cypermethrin with RR50 values of 1.47, 2.21, and 4.76, respectively. Based on the confidence intervals for LC95 values, temephos and deltamethrin resistance in the larvae differed significantly between the two field strains (Table 1).
Resistance ratio (RR50), for organophosphates (temephos and fenitrothion), the carbamate bendiocarb and pyrethroids (cypermethrin and deltamethrin), in larvae of Ae. aegypti from two strains from Costa Rica (Limon and Puntarenas)
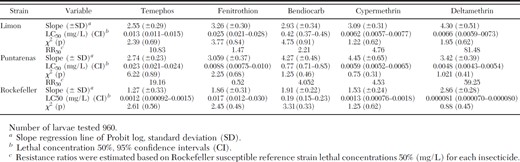
Resistance ratio (RR50), for organophosphates (temephos and fenitrothion), the carbamate bendiocarb and pyrethroids (cypermethrin and deltamethrin), in larvae of Ae. aegypti from two strains from Costa Rica (Limon and Puntarenas)
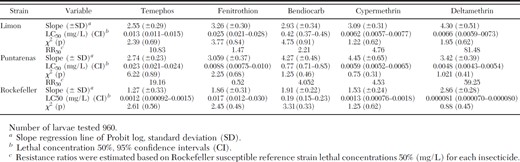
Effectiveness of Temephos in the Commercial Formulation (BIOLARV G1).
In this assay, insecticide was applied to the container on day 1 and the water was replaced every day without the further addition of insecticide. New batches of larvae were introduced each day and mortality was recorded after 24 h. A single temephos application resulted in 100% mortality for up to 22 d for the susceptible Rockefeller strain, but mortality rates dropped below 75% after just 9 d when the resistant reference strain (SANtem-F11) was tested (Fig. 2). The temephos efficacy in both the Puntarenas and Limon strains showed 100% mortality until 11 and 12 d with daily water replacements, which would be equivalent to a duration of 22 and 24 d without water replacement.
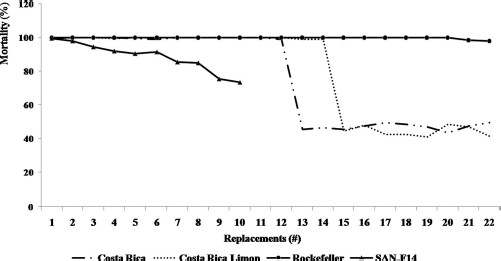
Effectiveness of the commercial temephos formulation (BIOLARV G1) in two strains of Ae. aegypti larvae collected in Costa Rica (Limon and Puntarenas) and the temephos-resistant (SANtem-F11) and susceptible (Rockefeller) reference strains.
Synergist Bioassays.
The synergists TPP, PBO, and EA were used to determine the role of esterases, cytochrome P450 monooxygenase, and glutathione S-transferase (GST) in conferring temephos and deltamethrin resistance (Table 2). Only TPP increased the toxicity of both temephos and deltamethrin. This synergist is a known inhibitor of esterase activity (Whyard et al. 1995), and therefore this result provides additional evidence for the importance of esterases in conferring insecticide resistance. According to the synergism ratio values (SR < 5), cytochrome P450 monooxygenase and glutathione S-transferase enzymes were not associated with temephos and deltamethrin resistance.
Lethal concentrations that caused 50% mortality (LC50) using the synergists triphenyl phosphate (TPP), piperonyl butoxide (PBO) and ethacrinic acid (EA), and synergism ratio (SR) for temephos and deltamethrin in the Costa Rican strains of Ae. aegypti
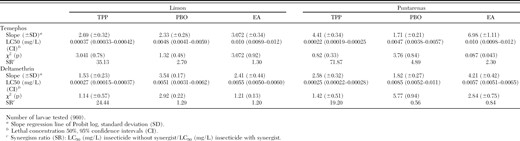
Lethal concentrations that caused 50% mortality (LC50) using the synergists triphenyl phosphate (TPP), piperonyl butoxide (PBO) and ethacrinic acid (EA), and synergism ratio (SR) for temephos and deltamethrin in the Costa Rican strains of Ae. aegypti
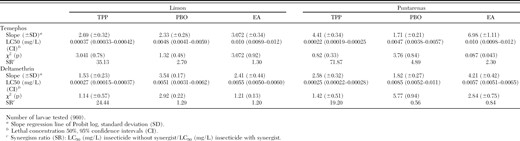
Biochemical Assays.
There were significant differences in the levels of esterase (P = 0.000001) and cytochrome P450 monooxygenase activities (P = 0.00002) between the Puntarenas and Limon strains and the susceptible Rockefeller strain, but there was no significant difference for GST (P = 0.33237) (Fig. 3A–C).
![A,B,C. Graphs showing the activity of esterase (A), cytochrome P450 monooxygenase (B), and GST (C) in larvae Ae. aegypti individuals from the Rockefeller susceptible strain and the two strains from Costa Rica (Puntarenas and Limon). Statistical comparisons of enzymatic activities were performed with ANOVA results for esterase (F[2.610] = 16. 309, P = 0.000001), cytochrome P450 monooxygenase (F[2.610] = 11. 184, P = 0.00002), and GST (F[2.76] = 1.1176, P = 0.33237 0.00002). Vertical bars denote 95% confidence intervals. Different letters denote differences in means according to Tukey's test.](https://oup.silverchair-cdn.com/oup/backfile/Content_public/Journal/jme/50/2/10.1603_ME12064/2/m_jmedent50-0352-fig3.jpeg?Expires=1750270632&Signature=Duy~cnpf1A-LQaQSDTVatz8XtPkFixGqHJn6tRTZmys~lK~89aW1OmByEY8-1LqOLIdJs8RiEwYZ709Si4PneqNSdDq0qA0H~1Cn4aQxmaH4sSFNH7d4qoIle7wMT~FJGoc-bNj9DcCxHiGNVXDjAaffeA8x2C0AhkdtCN-SE1mZ6qzdY0DMLSySmoGRnWuHJVSE8DWQ8mobsOthV4ooD0BBzlGcQpjIV8kSIiBC6T1QYvFHjPUr84e19NHzphHk1K1kEYb7GIvYqAff3esve9CtjBeMC7OnUM-UGiaXh2KjK6pEnUMV1w0DmdkRR3Ot6~6RTK9DXqeeUYeobvTc5g__&Key-Pair-Id=APKAIE5G5CRDK6RD3PGA)
A,B,C. Graphs showing the activity of esterase (A), cytochrome P450 monooxygenase (B), and GST (C) in larvae Ae. aegypti individuals from the Rockefeller susceptible strain and the two strains from Costa Rica (Puntarenas and Limon). Statistical comparisons of enzymatic activities were performed with ANOVA results for esterase (F[2.610] = 16. 309, P = 0.000001), cytochrome P450 monooxygenase (F[2.610] = 11. 184, P = 0.00002), and GST (F[2.76] = 1.1176, P = 0.33237 0.00002). Vertical bars denote 95% confidence intervals. Different letters denote differences in means according to Tukey's test.
Further results showed that 57 and 68% (Limon and Puntarenas) of larvae displayed elevated esterase activity (Table 3) relative to the activity cutoff values of larvae from the Rockefeller strain (mean + 3SD: 0.984-μmol β naphthol/min/mg protein). Cytochrome P450 monooxygenase activity in both strains was 8.5 and 15% (Limon and Puntarenas, respectively) above the cytochrome P450 monooxygenase activity cutoff for the Rockefeller strain (1.98-nmol cytochrome c/min/mg protein), indicating an “altered” activity. There was no evidence for increased GST activity among larvae from either Costa Rican strain (Table 3).
Frequency (%) of increased esterase and cytochrome P450 monooxygenase activities in Ae. aegypti larvae from two strains from Costa Rica
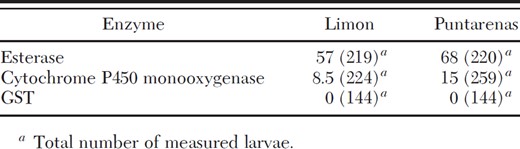
Frequency (%) of increased esterase and cytochrome P450 monooxygenase activities in Ae. aegypti larvae from two strains from Costa Rica
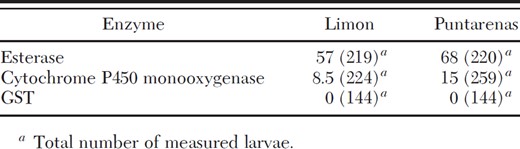
Acrylamide Gel Electrophoresis (PAGE).
Staining of the polyacrylamide electrophoresis gels with α- and β-napthyl acetate revealed three intense staining bands (Fig. 4) for strains from Limon and Puntarenas as well as the temephos resistant reference strain (SANtem-F11). Of note, each band appears in the susceptible Rockefeller strain but at very low intensities. One esterase band was determined to be an esterase type A, with a relative mobility (Rm) value of 0.78, which was classified previously as representing esterase A4 in a Cuban temephos-resistant strain (Rodríguez et al. 1999). The other two bands correspond to esterase type B, one of which is evident in SANtemF11 with an Rm value of 0.6 and the other in the deltamethrin resistant reference strain (SAN-F14) with an Rm value of 0.86.
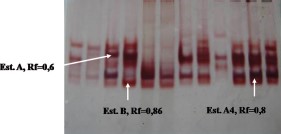
Zymogram of esterases in larvae from Ae. aegypti strains: susceptible Rockefeller (lanes 1 and 2), resistant to temephos SANtem-F11 (lane 3), resistant to deltamethrin, SAN-F14 (lane 4), SANtem-F11 strain survivors of selection with temephos (lane 5), SAN-F14 strain survivors of selection with deltamethrin (lane 6), Puntarenas strain (lanes 7–9), and Limon (lanes 10–12). (Online figure in color.)
Adult Bottle Assays.
The pyrethroids deltamethrin and cypermethrin, and the organophosphate chlorpyrifos were measured for resistance in adult mosquitoes. The Limon and Puntarenas strains showed complete susceptibility to chlorpyrifos and cypermethrin, but resistance to deltamethrin was detected in both strains. The DD for cypermethrin, deltamethrin, and chlorpyrifos in the Rockefeller strain was 13.5 μg/bottle, 6.5 μg/bottle, and 90 μg/bottle in 30 min, respectively. When the Limon and Puntarenas strains similarly were tested, 100% mortality was observed for cypermethrin (Fig. 5A) as well as for chlorpyrifos (Fig. 5B). However, both strains showed deltamethrin resistance, wherein 7.55 and 9.52% survival were observed in the Limon and Puntarenas strains, respectively (Fig. 5C).
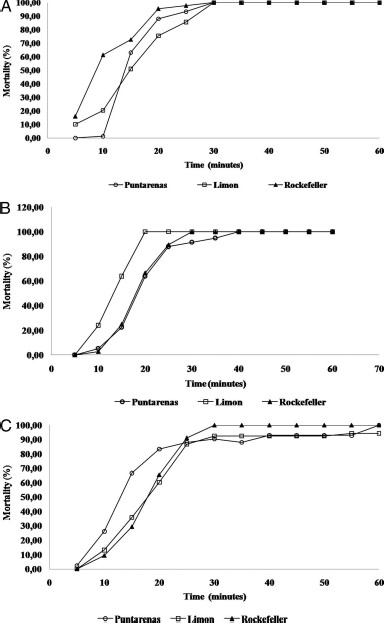
A,B,C. Percent mortality and mortality time of Ae. aegypti Rockefeller, Puntarenas, and Limon strains after exposure to 13.5 ìg/bottle cypermethrin (A), 90 ìg/bottle chlorpyrifos (B), and 6.5 ìg/bottle deltamethrin.
Discussion
The larvicide temephos is the most widely used chemical in Costa Rica for suppression of Ae. aegypti populations through source reduction. Because this remains a primary strategy for DENV control, development of temephos resistance would have a significant impact on disease control efforts. To date, there is little information available on insecticide resistance among Ae. aegypti populations from Costa Rica, although temephos resistance was reported among populations from several Latin American countries, including Costa Rica, Panama, Jamaica, Nicaragua, Peru, and Venezuela (Rodríguez et al. 2007). Subsequent work also demonstrated the presence of temephos resistance in a strain of Ae. aegypti from El Salvador (Bisset et al. 2009), and additional studies have confirmed the trend toward increased resistance to this larvicide in Brazil (Lima et al. 2011) and Cuba (Bisset et al. 2011).
The Costa Rican strains showed high resistance to temephos, wherein the efficacy of the commercial product and the duration of its residual effect was 100% mortality for up to 11 and 12 d with daily water replacement. This indicates that this larvicide should still be effective if it is applied within a frequency of ≈22–24 d. Montella et al. (2007) confirmed a decrease in temephos residual effect in Brazil where the RR exceeded 10. In Ae. aegypti from Cuba for strains with high resistance (RRs > 10.0), the residual effect of temephos was between 9 and 13 d (Bisset et al. 2011). Moderately resistant populations (RR between five and 10) and susceptible populations displayed residual effect susceptibility to temephos for ≈14–18 d. These results suggest the importance of including other larvicides such as Bti or pyriproxyfen in a control program, as both these products have been approved by WHO for use in drinking water (WHO 2003, 2006). Including these in mosquito control programs would help to preserve the effectiveness of temephos, which has been used in the Americas for over 25 yr.
Complete larval susceptibility was observed to the OP fenitrothion, the carbamate bendiocarb, and the pyrethroid cypermethrin, whereas high resistance to the pyrethroid deltamethrin was observed. Deltamethrin resistance in larvae could be attributed to applications targeting adults or could be transmitted from the resistant adult female to the progeny. In some Latin-American countries, including Costa Rica, it was reported previously that all the strains were susceptible to the OP fenitrothion and resistant to the pyrethroids deltamethrin and cypermethrin (Rodríguez et al. 2007). The level of Ae. aegypti susceptibility to fenitrothion and bendiocarb should be measured in the adult mosquitoes from Costa Rica, to know if they could be included as good candidates in the Ae. aegypti control strategies. In Cuba, fenitrothion was used for Ae. aegypti control since 1981–1986 for residual treatment and this species continuously showed susceptibility in Ae. aegypti (Rodríguez et al. 2010). In a small trial conducted in Villa Clara, Cuba, it was demonstrated that bendiocarb, in its formulation FICAM 80 WP, was effective for Ae. aegypti control for up to a 3-mo period (Castex et al. 2007).
Chemical compounds that specifically inhibit the enzymes that metabolize insecticides, thus avoiding acting on a specific target site, have been useful for studying resistance mechanisms because of metabolic activities. These chemicals are classified as synergistic, as they are able to enhance the action of insecticides through these inhibitory actions. The synergists most widely used for studying the mechanisms of resistance in Ae. aegypti are triphenyl phosphate (TPP), piperonyl butoxide (PBO), and ethacrynic acid (EA), and these represent esterase, cytochrome P450 monooxygenase, and GST inhibitors, respectively. In this study, we used synergists to demonstrate that temephos and deltamethrin resistance identified in larvae from Costa Rican strains were associated with esterase activity, but were not associated with either cytochrome P450 monooxygenase or GSTs. Recent work in Colombia (Fonseca-González et al. 2011) and Trinidad and Tobago (Polson et al. 2011) showed that both resistance to organophosphates and pyrethroids were associated with esterase and cytochrome P450 monooxygenase resistance mechanisms. Studies in Cuba (Rodríguez et al. 2002) and Brazil (Melo-Santos et al. 2010) have shown that temephos resistance is related to the increased activity of all three enzyme classes (esterase, cytochrome P450 monooxygenase, and GST) in Ae. aegypti, whereas other reports have shown that increased activities of nonspecific esterases are the most important resistance mechanisms associated with this larvicide (Bisset et al. 2011).
Pyrethroid resistance in Ae. aegypti has been associated mainly with reduced target site sensitivity arising from a single point mutation in the sodium channel gene, commonly referred to as knockdown (kdr)-type resistance (Brengues et al. 2003, Saavedra et al. 2007, Harris et al. 2010). However, metabolic resistance can also be associated with pyrethroid resistance, as has been demonstrated previously for deltamethrin in Cuba (Rodríguez et al. 2002, 2005, 2007). More recently, Polson et al. 2011 demonstrated that metabolic detoxification of enzymes was correlated with the dichlorodiphenyltrichloroethane (DDT) and pyrethroid resistance in Trinidad and Tobago populations of Ae. aegypti, but also suggested that kdr-type resistance could be involved. The presence of both metabolic and kdr resistance mechanisms was found in Ae. mosquitoes from Martinique Island (Marcombe et al. 2009).
Based on relative mobility and specificity of reaction with α naphthylacetate, the elevated esterase band observed in samples from Costa Rica is consistent with the esterase A4 found in the larvae of the temephos resistant strain collected in Santiago de Cuba in 1997 (Rodríguez et al. 1999). It has been shown in several countries that temephos resistance in Ae. aegypti is correlated with the esterase A4, including in Peru (Bisset et al. 2007), El Salvador (Bisset et al. 2009), and several other Latin-American countries (Rodríguez et al. 2007). Mazzarri et al. (1995) found moderate levels of temephos resistance in two Ae. aegypti strains collected in the Coro and Maracay states in Venezuela, which were associated with the presence of an esterase band with a relative mobility (Rm) of 0.61 with 91% of mosquitoes tested. This esterase was classified as A5 and did not show high levels of activity in the susceptible strain. The role of the variation in specific esterase activities with insecticide resistance also was demonstrated in Brazil (Sousa-Polezzi et al. 2005).
Results from adult bioassays of Costa Rican strains showed resistance to deltamethrin but complete susceptibility to cypermethrin and chlorpyrifos. Other countries in the region have successfully used these insecticides in Ae. aegypti control programs. For example, in the 1997 and 2001 DENV epidemics in Cuba, the insecticide strategy used was to apply cypermethrin in rotational schemes with chlorpyrifos, which may have limited the emergence of cypermethrin resistance and maintained its utility for successful Ae. aegypti population suppression (Montada et al. 2006).
In summary, resistance to temephos and deltamethrin is emerging at the two study sites from Costa Rica, indicating that additional research is necessary to more broadly survey other regions of the country. Such efforts are needed to improve insecticide use strategies and reverse this phenomenon. The insecticides chlorpyrifos and cypermethrin should be good candidates to use as alternatives to use for Ae. aegypti control.
References Cited