-
PDF
- Split View
-
Views
-
Cite
Cite
Linda Kothera, Marvin Godsey, John-Paul Mutebi, Harry M. Savage, A Comparison of Aboveground and Belowground Populations of Culex pipiens (Diptera: Culicidae) Mosquitoes in Chicago, Illinois, and New York City, New York, Using Microsatellites , Journal of Medical Entomology, Volume 47, Issue 5, 1 September 2010, Pages 805–813, https://doi.org/10.1093/jmedent/47.5.805
- Share Icon Share
Abstract
Aboveground and belowground populations of the mosquito Culex pipiens L. are traditionally classified as form pipiens and form molestus, respectively, and gene flow between forms is thought to be limited. Relatively few f. molestus populations have been found in the United States, which has hindered their study in North America. In this study, we used microsatellites to characterize a newly discovered population of f. molestus in Chicago, IL, and compared levels of genetic diversity and differentiation in aboveground and belowground populations from Chicago and New York City, NY. Levels of genetic diversity, as measured by expected heterozygosity and allelic richness, were markedly lower in both f. molestus populations. Allele frequencies were distinctly different between the two f. molestus populations, and some alleles were present in one belowground population and not the other. Pairwise FST values between populations indicated that f. molestus populations were highly divergent from each other, as well as from their associated aboveground populations. Cluster analysis suggested the most likely number of groups was three, with the four f. pipiens populations in one cluster, and each of the f. molestus populations in its own cluster. Admixture analysis detected a low number of hybrids, 8%, between forms. We also tested the efficacy of two assays purported to distinguish between the forms, the CQ11 assay and a restriction fragment-length polymorphism assay of the COI gene, and found neither assay reliable in this regard. Our findings support the hypothesis that f. molestus populations in Chicago and New York City arose from local aboveground populations.
A hallmark of a subdivided population is the presence of genetic structure, which arises from limited gene flow between subpopulations. Populations of Culex pipiens may occupy open, aboveground habitats or confined, belowground habitats, the latter of which is thought to have arisen as an adaptation to coexistence with humans (Barr 1967, Spielman 1967, Chevillon et al. 1995). Physiological characteristics associated with living belowground include autogeny, or the ability to produce the first egg raft without a blood meal, and a lack of winter diapause, despite living in a temperate climate (Chevillon et al. 1995, 1998; Vinogradova 2000).
These populations are currently recognized as two forms of Cx. pipiens: form pipiens and form molestus Forskäl (Harbach et al. 1984). The f. molestus individuals are associated with belowground habitats and are stenogamous (able to mate in confined spaces), whereas f. pipiens occupy aboveground habitats and are eurygamous (mate in swarms in large areas). Although f. molestus has been studied often in Europe and the Middle East, where it is thought to have originated (Knight and Abdel Marek 1951, Urbanelli et al. 1981, Nudelman et al. 1988, Byrne and Nichols 1999, Weitzel et al. 2009, Gomes et al. 2009), relatively few populations have been found in the United States (Boston, MA, Spielman 1957; New York City, NY, Kent et al. 2007, Huang et al. 2008; San Mateo County, CA, McAbee et al. 2004; Philadelphia, PA, Kilpatrick et al. 2007; and Chicago, IL, Wray 1946, Mutebi and Savage 2009), which has impeded their study in North America. Both f. pipiens and f. molestus are efficient vectors of West Nile and other arboviruses (Tahori et al. 1955, Turell et al. 2006, Kramer et al. 2008).
The persistence of f. molestus in enclosed, isolated habitats raises the question of how this form evolved. Early cytological work by Laven (1959) was supportive of the idea that f. molestus populations did not share a common origin. Although Byrne and Nichols (1999) found little genetic similarity between populations of f. molestus in the London subway system and the nearby aboveground populations of f. pipiens, they argued their results were still consistent with a single colonization event by local f. pipiens. According to their most parsimonious scenario, colonization was followed by a subsequent increase in genetic differentiation and a decrease in genetic diversity among underground populations. Weitzel et al. (2009) examined populations of both forms from locations in Europe and asserted that belowground f. molestus populations could not have arisen from their associated aboveground f. pipiens populations because the degree of genetic divergence was lower among populations of f. molestus than between f. molestus and f. pipiens. With regard to United States populations, Huang et al. (2008) could not exclude the possibility that the belowground f. molestus population on 91st Street in New York City (designated NYMolG0 in the current study) originated from colonization by aboveground f. pipiens. Fonseca et al. (2004) asserted that in the United States: 1) f. pipiens populations show a high degree of hybrid (with f. molestus) ancestry and that 2) f. molestus is derived from European f. molestus. However, that study failed to include United States populations of f. molestus in the analyses. If f. molestus in the United States is derived from European populations, we would expect f. molestus populations in the United States to be genetically similar to one another. However, if each population of f. molestus arose independently from pipiens ancestors, we would expect f. molestus populations in the United States to be genetically diverged from one another, and to share their complement of alleles with nearby aboveground populations.
In this study, we use the tools of population genetics to describe a heretofore uncharacterized f. molestus population collected from Chicago, IL, and to compare aboveground and belowground populations of Cx. pipiens from Chicago and New York City, NY.
Materials and Methods
Specimens were collected from three locations (two aboveground and one belowground), each in Chicago and New York City between 2005 and 2009 (Table 1; Fig. 1). The low number of individuals (n = 20) collected from the belowground location in New York City precluded colonization attempts, so those specimens were frozen and used for genetic analysis as representative of the wild population and designated NYMolG0. For comparative purposes, we established a colony of f. molestus from colony specimens originally obtained from the NYMolG0 site. Aboveground specimens from New York City, collected from Fort Totten in Queens (NYFT) and Greenwood Cemetery in Brooklyn (NYGC), were colonized, and a subsample of field-collected individuals from each site was used for this study. Similarly, field-collected belowground individuals from the Calumet Water Reclamation Plant in Chicago (Mutebi and Savage 2009), designated ChiMolG0, were used in this study and also established in colony. Aboveground specimens from Chicago, designated Chi16 and Chi17A, were collected as part of an earlier study (Kothera et al. 2009). A total of 242 specimens was examined in our study.
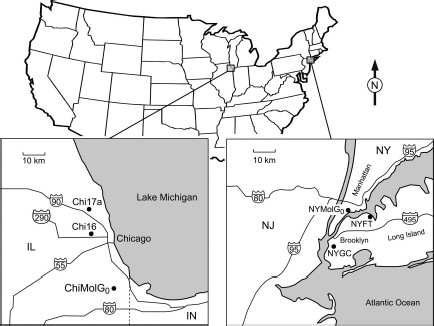
Map showing locations of populations used in this study. Maps of individual sites are at the same scale.


Initial Morphology and Genetic Screening.
Field-collected specimens were examined morphologically, as described in Savage et al. (2007), to determine species or lowest taxonomic unit. Specimens from the two belowground populations were previously determined to be autogenous (Huang et al. 2008, Mutebi and Savage 2009; Table 1). Diapausing females from the aboveground populations in New York City were collected in winter from hibernacula, and autogeny was confirmed when in colony by delaying blood meals for 2 wk after placing pupae into clean cages as part of routine colony maintenance. Egg raft production ceased until blood meals were resumed, so the NYFT and NYGC populations were deemed anautogenous. The aboveground populations in Chicago, Chi16 and Chi17A, were not tested for autogeny, as they were field collected and not colonized (Table 1).
DNA extraction and visualization of polymerase chain reaction (PCR) fragments were described previously (Kothera et al. 2009). Briefly, individual mosquitoes were homogenized with BA-1 diluent, and DNA was extracted from a portion of the sample. Specimens were screened initially with the internal transcribed spacer(ITS) assay (Crabtree et al. 1995, Aspen et al. 2003) to confirm membership in the Cx. pipiens species complex. A panel of eight microsatellite loci was used to assess genetic diversity and differentiation. All loci were from previously published work (Fonseca et al. 1998, Keyghobadi et al. 2004, Smith et al. 2005, Edillo et al. 2007; Table 2). PCR conditions were as described in the original sources, and loci were multiplexed into two groups. Fragments were visualized on a Beckman Coulter (Fullerton, CA) CEQ8000 sequencer, and a multilocus genotype was generated for each individual with the sequencer's fragment analysis module. Approximately 10% of the individuals in the study were analyzed twice, and results from both runs were identical.
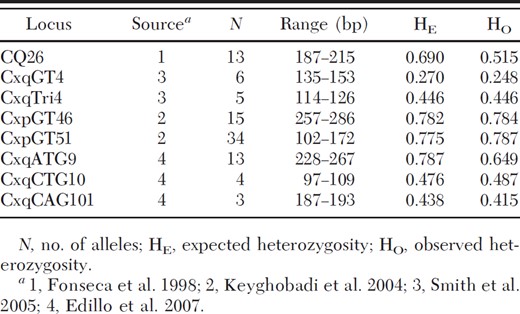
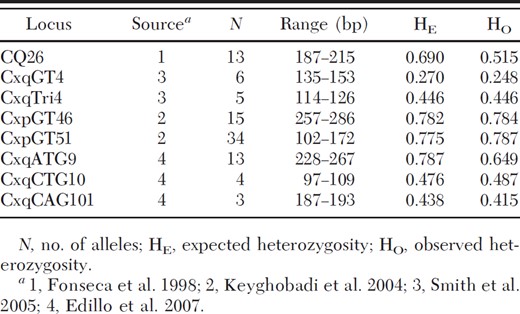
In addition, specimens were evaluated with two assays that purportedly differentiate the two forms: the CQ11 locus (Bahnck and Fonseca 2006), and Shaikevich's (2007) restriction fragment-length polymorphism assay of the COI gene. CQ11 is a microsatellite marker that reportedly produces form-specific bands, with both bands present in hybrids. The restriction fragment-length polymorphism assay takes advantage of a reported one-nucleotide difference between the forms in a region of the COI gene. PCR was conducted as per their respective published protocols. Results of the CQ11 assay were visualized on 2% agarose gels, and individuals were scored as f. molestus, f. pipiens, or hybrid. PCR products from the restriction fragment-length polymorphism assay were cloned and sequenced from one individual from each of the aboveground populations in Chicago and New York City (n = 4), as well as two individuals each from the belowground populations (n = 4).
Data Analysis.
Convert (Glaubitz 2004) was used to format genotypic data for subsequent analyses in Arlequin 3.1 (Excoffier et al. 2005), Structure (Pritchard et al. 2000), and FSTAT 2.9.3 (Goudet 1995), as well as to generate tables of allele frequencies. Genetic diversity as measured by observed heterozygosity and expected heterozygosity (HE) was calculated using Nei's (1987) unbiased estimate in Arlequin, which was also used to evaluate departures from Hardy-Weinberg (HWE) and linkage equilibrium. FSTAT was used to derive values for allelic richness, which were calculated using a sample size corrected method. The program Bottleneck (Piry et al. 1999) was used to determine whether any of the populations displayed signs of having undergone a recent reduction in effective population size. A statistically significant (P < 0.05) Wilcoxon signed rank test is interpreted as evidence of a genetic bottleneck. The program also uses a graphical descriptor called a mode-shift, which notes changes in the distribution of allele frequencies characteristically associated with populations that have undergone bottlenecks.
An analysis of molecular variance (AMOVA) was conducted in Arlequin to quantify genetic variation at various levels in this system: within individuals, within populations, and among populations. Arlequin was also used to generate fixation indices, or F statistics, FST, FIT, FIS (Wright 1995) which assess the degree of inbreeding and genetic differentiation in and among populations. Statistical significance was obtained via permutation tests. Microsatellite Analyzer (Dieringer and Schlötterer 2003) was used to produce a matrix of percent shared alleles between pairs of populations, and a neighbor-joining tree was generated from the resulting matrix using Phylip (Felsenstein 1993).
Structure was first used to infer the mostly likely number of clusters (K). Fifty runs using the default parameters, without prior population information, were performed for each value of K ranging from 2 to 8, and consisted of 500,000 burn-in steps, followed by 1,000,000 data-collecting steps. We calculated both the posterior probability associated with each number of clusters, and used Evanno et al.'s (2005),ΔK method. In addition, the Structure results were used to calculate a similarity coefficient (Rosenberg et al. 2002), which evaluated the stability of individual assignments. Values for this metric can range from 0 to 1, and values closer to 1 indicate that individuals are consistently placed in the same cluster.
Structure was also run to assign individuals to clusters and to detect hybrids between the two forms. A hybrid was defined as an individual with a qi (probability of membership) value of <0.90 in any one cluster (Vaha and Primer 2006), whereas pure f. pipiens or f. molestus were defined as having qi values of ≥0.90 in a cluster. Distruct (Rosenberg 2004) was used to visualize Structure results.
Results
Within Population Patterns of Genetic Diversity.
Two instances of significant (Bonferroni corrected α = 0.001; Rice 1989) departures from HWE were found in the Chi16 population. As loci were generally in HWE in the other populations in our study, as well as in a previous study (Kothera et al. 2009), all loci were retained for further analyses. Five instances (of 168 comparisons) of significant linkage disequilibrium were found after Bonferroni correction. Because each instance involved a different pair of loci, it was assumed the loci were statistically independent of each other.
A total of 98 alleles was scored, and the number of alleles per locus ranged from 4 to 35 (Table 2). Several instances of private alleles were observed, but they were all at a frequency of 4% or less. Allelic richness in the belowground populations was about half that of the aboveground populations (range, 2.742-7.291), and a similar trend was observed for the overall measure of genetic diversity, the average HE per site (range, 0.383–0.670; Table 3).
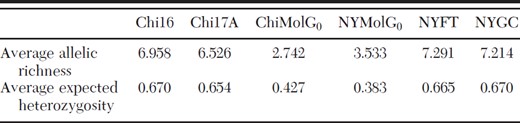
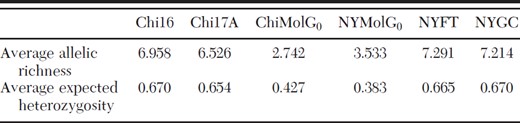
Some marked differences in frequencies were observed for several alleles in the belowground populations (Table 4). In four instances, a locus was monomorphic in one population and not the other. For example, the 149-bp allele of locus CxqGT4 is monomorphic in the NYMolG0 population, and present at a frequency of 0.490 in the ChiMolG0 population. In other cases, an allele was present in one belowground population and absent in the other, as in the 187-bp allele of locus CxqCAG101, which is absent from the ChiMolG0 population, but present in the NYMolG0 population at a frequency of 0.250. There were 31 private alleles within the two belowground populations: 11 occurred at a frequency of <5%, and 20 occurred at a frequency of ≥5%. In addition, all of the alleles found in each belowground population were also found in the local aboveground populations.
Comparison of selected allele frequencies between the two f. molestus populations
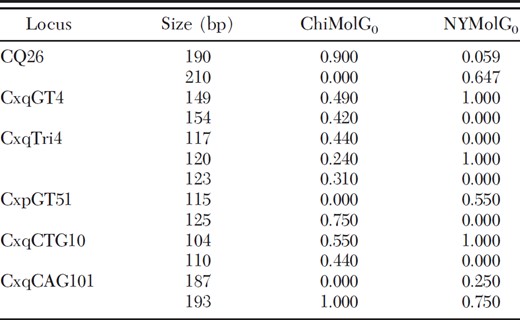
Comparison of selected allele frequencies between the two f. molestus populations
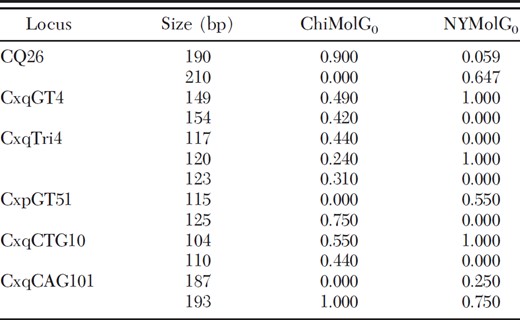
Bottleneck results indicated the ChiMolG0 population showed a statistically significant departure from mutation-drift equilibrium (one-tailed P value for Wilcoxon's test for an excess heterozygosity = 0.020). In addition, both the ChiMolG0 and the NYMolG0 populations exhibited a mode-shift in their allele frequency distributions, suggesting both populations have experienced a genetic bottleneck (Table 5).
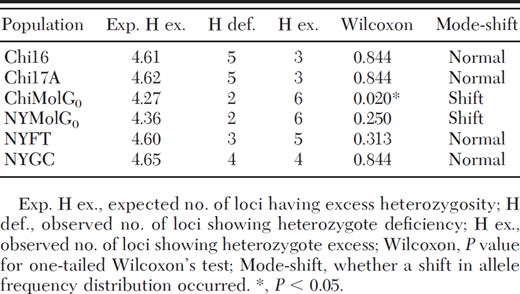
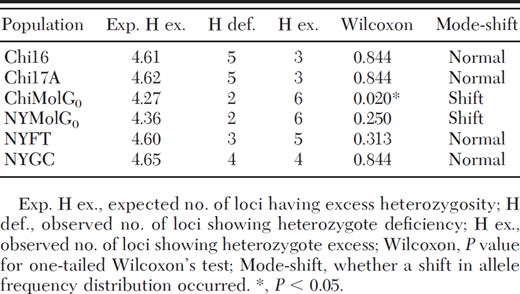
Among Population Differentiation.
Results from the AMOVA indicated the majority of genetic variation was found at the individual level (81.23%), and the remaining amount was partitioned within (6.06%) and among (12.71%) populations (Table 6). Mean fixation indices were significantly different from 0 (P < 0.0001) via permutation tests in Arlequin (FIS = 0.069; FST = 0.127; FIT = 0.188). The Chi16 site showed higher levels of inbreeding than the other sites, because of comparatively higher FIS values at two loci. Pairwise FST values among the four aboveground populations in Chicago and New York City were not significantly different (Table 7). In contrast, the two belowground populations were highly differentiated from each other (FST = 0.394) as well as from their respective aboveground populations (FST range from 0.152 to 0.253).
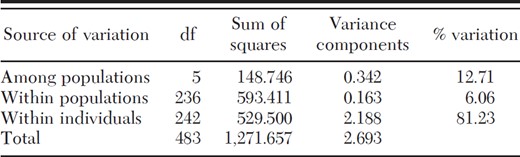
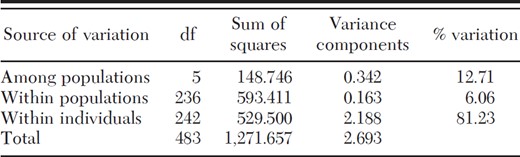
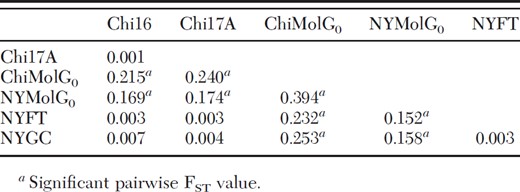
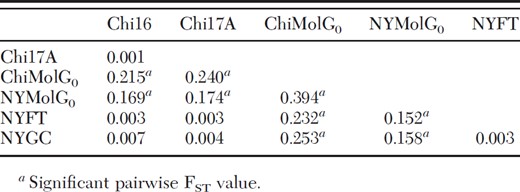
The neighbor-joining tree based on the proportion of shared alleles shows each pair of aboveground populations clustering together, and the group of aboveground populations forming a larger group (Fig. 2). The belowground populations are positioned on the tree with a maximum of distance between each other and the aboveground populations.

Neighbor-joining tree of six populations based on proportion of shared alleles. Belowground populations include “Mol” in the name, and aboveground populations include “pip” in the name.
Cluster Analyses.
According to their posterior probabilities, the most likely number of clusters was three, with each of the two belowground populations in its own cluster, and all four of the aboveground populations in the third cluster (Fig. 3A). At higher values of K, which are not suggested by either posterior probabilities or similarity coefficients, the number of clusters an individual belonged to increased for individuals belonging to the four aboveground populations, but remained the same for individuals in the belowground populations (Fig. 3, B and C). The similarity coefficients had a value of 1 when K = 2 or K = 3, indicating that individuals were placed into the same cluster consistently with either value of K, and larger values of K had successively smaller similarity coefficients (Fig. 4). Evanno's ΔK method suggested the most likely number of clusters was two. When Structure was constrained to two clusters, the ChiMolG0 population occupied its own cluster, with the remaining cluster comprised of all of the aboveground populations as well as the NYMolG0 population.
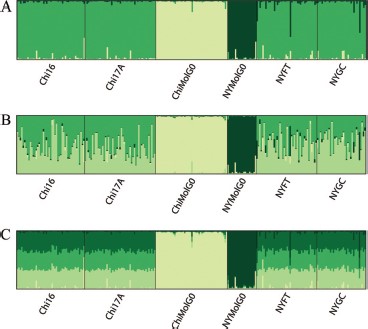
Results from Structure analysis, with default settings and no prior population information. A, Shows individual assignments for the most likely number of clusters, K = 3. B and C, Illustrate that f. molestus individuals remain in their respective clusters, and the f. pipiens cluster arbitrarily divides into further clusters when K = 4 and K = 5, respectively.
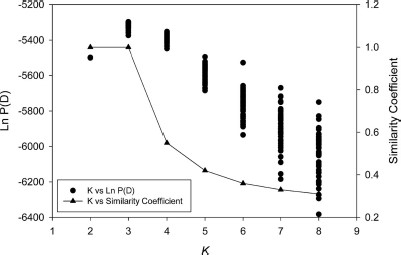
Graph showing the posterior probabilities, Ln P(D), associated with 50 Structure runs for each value of K (number of clusters), ranging from 2 to 8. Also shown, on the second y-axis, is the similarity coefficient (Rosenberg et al. 2002) for each value of K, which was calculated from the Structure results and plotted as a function of K.
Twenty individuals (8%) were classified as hybrids between forms using qi = 0.90. Hybrids were most common in the aboveground sites in New York City, which each had five hybrid individuals (Table 8).
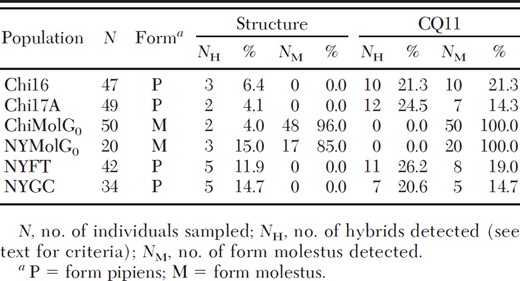
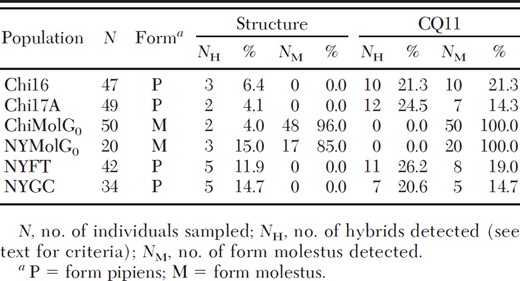
Comparison of Methods to Detect Forms and Hybrids.
We were unable to use Shaikevich's (2007) restriction fragment-length polymorphism assay because there were no sequence differences between aboveground and belowground individuals in the relevant region of the COI gene. Two clones were sequenced twice in each direction, and the results were consistent over all runs. Whereas f. pipiens in the Shaikevich (2007) study had a sequence of GGCC in the relevant region, and f. molestus had AGCC, all of the individuals sampled for our study had the sequence GGCC. We then direct sequenced an additional two f. molestus individuals (twice in both directions) each from NYMolG0 and ChiMolG0, and those individuals also had the GGCC sequence. Sequences have been deposited on GenBank, received 15 January 2010 (accession numbers GU473997-GU474012). The CQ11 assay and the full microsatellite analysis detected about equal numbers of f. molestus individuals in belowground populations (Table 8). However, the CQ11 assay incorrectly classified 14.3–21.3% of individuals in each aboveground population as f. molestus, whereas Structure detected no f. molestus in these populations. Overall, the CQ11 assay classified about twice as many individuals as hybrids as the Structure analysis, with the exception of the NYGC population, in which each analysis detected five hybrid individuals.
Discussion
Whereas aboveground populations in Chicago and New York City show moderately high levels of genetic diversity and little population structure, belowground populations in both locations displayed reduced genetic diversity and were highly divergent from their corresponding aboveground populations and from each other.
Inspection of pairwise FST values (Table 7) demonstrates several trends among populations. First, there is a high degree of similarity among the aboveground populations. This is notable, given that Chicago and New York City are over 1200 km apart. In contrast, each belowground population is divergent from all other populations, and the two belowground populations are the most divergent of all. Noticeably different allele frequencies, such as those shown in Table 4, may account for the observed degree of divergence. The two f. molestus populations differed not only in the frequencies of several alleles, but also in the presence or absence of certain alleles. In addition, these trends are supported by the population level measures of genetic diversity. Both allelic richness and HE were noticeably reduced in the two belowground populations, whereas values associated with the four aboveground populations were similar and comparatively larger. Given that the aboveground populations are so similar, the high degree of genetic divergence in belowground populations suggests they have persisted over time with little, if any, gene flow. Our results are also supportive of the idea that the markers used in the study are selectively neutral and therefore good indicators of genetic divergence. If any of the loci were linked to traits associated with adapting to an underground environment, we would expect to observe more similarities than were seen in our study. Finally, the neighbor-joining tree based on the proportion of shared alleles provides an additional way to visualize the degree of divergence among populations, and is consistent with the other analyses. In particular, the ChiMolG0 population appears to share the smallest proportion of alleles, and its branch is the longest on the tree (Fig. 2).
The lack of diagnostic sequence differences corresponding to a restriction enzyme site in the target region of the COI gene in the United States populations of f. pipiens and f. molestus that we analyzed is in contrast to the findings of Shaikevich (2007) based on analysis of Russian populations. All of the United States populations that we tested contained sequence indicative of f. pipiens. If the Shaikevich (2007) assay is truly diagnostic of f. pipiens and f. molestus in the Old World, our results suggest that the two United States f. molestus populations we sampled are related to and likely evolved from f. pipiens and not from Old World f. molestus. Further analysis of COI sequence in other populations, particularly those from North America and southern Europe, would help resolve this issue.
The higher posterior probability values for K = 3, combined with a similarity coefficient of 1 (Fig. 4) and the high degree of genetic differentiation observed (Table 7), indicate that the most likely number of clusters in this system is three. Even though the Evanno et al. (2005) method suggested that K = 2, the presence of two clusters is not supported by the posterior probability or the genetic differentiation data. It is clear from Fig. 3 that the assignments of individuals from the belowground populations to their clusters are very stable, even when K is increased beyond 3. The aboveground populations form one relatively homogeneous cluster, and increasing K beyond 3 does not suggest any further genetic structuring in the system. The small similarity coefficients associated with K = 4 and above (Fig. 4) fail to support additional structuring among the aboveground f. pipiens populations. Indeed, when Structure assigns specimens to a greater number of clusters than is supported by the data (Fig. 3, B and C), the proportions of each individual assigned to the additional clusters are roughly symmetric, as pointed out by Pritchard et al. (2000).
The admixture analysis revealed a number of hybrids in all aboveground populations, which ranged from 4 to 15%. It would appear from these results that gene flow between aboveground and belowground populations is generally low, and usually unidirectional from f. molestus to f. pipiens, a pattern observed in other studies (Gomes et al. 2009, Huang et al. 2009). The aboveground populations in New York City exhibit slightly more hybridization than those in Chicago, which underscores the degree to which the ChiMolG0 site was physically and genetically isolated from its associated aboveground sites (Mutebi and Savage 2009).
Several studies have documented a low degree of hybridization between forms, as measured by the presence of both stenogamy and autogeny in aboveground and belowground habitats outside of the United States (France, Pasteur et al. 1977; Italy, Urbanelli et al. 1981; Japan, Sasa et al. 1966; Russia, Vinogradova 2000). One well-characterized autogenous population in the United States was studied by Spielman (1957, 1964, 1971, 1973), who also found limited hybridization between forms and concluded that whereas the two forms are interfertile in the laboratory, behavioral and ecological factors reduce opportunities for hybridization to occur, and thus serve as effective reproductive isolating mechanisms.
Fonseca et al. (2004) examined the incidence of hybridization between f. pipiens and f. molestus, and the present work used some of the same markers as that study. Whereas both studies detected hybrids, the proportion of hybrids between forms in the United States differed greatly, with 40% of aboveground Cx. pipiens individuals identified as hybrids in the Fonseca study. In contrast, 8% of aboveground Cx. pipiens were identified as hybrids in our study, a finding consistent with studies by Huang et al. (2008) and Gomes et al. (2009), which identified 8–12% of aboveground Cx. pipiens individuals as hybrids. Fonseca et al. (2004) used a qi value of 0.94 when interpreting the results from their Structure analysis, which would result in a higher number of specimens being classified as hybrid, but this difference could not account for the discrepancy. One explanation for the difference could be that the Fonseca study did not include any f. molestus populations from the United States. It is possible that f. molestus in the United States is unlike European f. molestus, perhaps being more isolated and therefore more genetically differentiated, although still closely related to f. pipiens. The COI sequence data from the current study support this assertion, as does our finding that the belowground populations had a subset of the alleles found in the local aboveground populations. Future studies comparing DNA from f. molestus from within and outside the United States could shed light on this question.
Compared with the proportion of hybrids detected by the full microsatellite analysis, the CQ11 assay overestimated the number of hybrids and incorrectly classified a significant proportion of f. pipiens specimens as f. molestus (Table 8). There are several possible reasons why this is the case. First, CQ11 is a single locus assay and, as such, its inheritance pattern would be accurate only in F1 hybrids. In nature, other classes of hybrids are likely to be encountered, which could alter the frequency of genotypes observed. Also, a full microsatellite analysis that generates multilocus genotypes uses more information than a single gene assay, and therefore, portrays a more complete picture of the population. Finally, because the CQ11 marker itself is a microsatellite locus, it is possible that some f. pipiens individuals have the same allele size that Bahnck and Fonseca (2006) ascribed to f. molestus, even if that allele is monomorphic in f. molestus. The first two points above were raised by Bahnck and Fonseca (2006), but the current study raises the issue of the CQ11 assay overestimating the number of individuals classified as hybrids, as well as misclassifying some individuals as f. molestus when those individuals were collected from an aboveground population. In light of our results, the CQ11 assay should not be used to detect hybrids or f. molestus in aboveground populations.
This study describes the first population genetic study performed on a newly described population of Cx. pipiens f. molestus from Chicago. Comparisons to another f. molestus population in New York City, as well as to aboveground populations in both cities, indicate that these f. molestus populations are highly genetically divergent from each other and their associated aboveground populations.
Acknowledgements
The Andreadis laboratory at the Connecticut Agricultural Experiment Station (New Haven, CT) provided egg rafts that were used to establish the New York City colony of f. molestus. Chris Richards (United States Department of Agriculture, Center for Genetic Resources Preservation, Fort Collins, CO) provided assistance with statistical analyses and commented on an earlier draft of this manuscript. Staff from the Office of Vector Surveillance and Control in the New York City Department of Health and Mental Hygiene located and provided safe access to specimens. Staff from the Metropolitan Water Reclamation District of Greater Chicago provided assistance with locating and providing safe access to specimens. We appreciate the helpful comments of two anonymous reviewers.
References Cited