-
PDF
- Split View
-
Views
-
Cite
Cite
Kevin B. Temeyer, Pia U. Olafson, Robert J. Miller, Genotyping Mutations in BmAChE3: A Survey of Organophosphate-Resistant and -Susceptible Strains of Rhipicephalus (Boophilus) microplus, Journal of Medical Entomology, Volume 46, Issue 6, 1 November 2009, Pages 1355–1360, https://doi.org/10.1603/033.046.0614
- Share Icon Share
Abstract
Mutations I48L, I54V, R86Q, V137I, I492M, and T548A were identified previously in BmAChE3, a gene encoding acetylcholinesterase, from the organophosphate (OP) acaricide-resistant San Román strain of Rhipicephalus (Boophilus) microplus. Recombinant BmAChE3 acetylcholinesterase containing the R86Q mutation was shown to exhibit nearly 20-fold reduction in the rate of phosphorylation by paraoxon relative to the wild-type sequence. In addition, the R86Q mutation was present in resistant laboratory strains at elevated frequency compared with OP-susceptible strains but was insufficient to alone generate the OP-resistant phenotype (J. Med. Entomol. 44: 1013–1018). Here, we developed assays to genotype the remaining five mutations and evaluated frequency of all six BmAChE3 mutations in individual R. microplus ticks from laboratory and Mexican field-collected strains. We found a substantial number of individuals in known OP-susceptible strains that seemed to be homozygous for each of the mutations surveyed, the exception being I48L, which was infrequent in all strains, leading us to conclude that none of the mutations alone were responsible for generation of phenotypic resistance to OP acaricide.
The southern cattle tick, Rhipicephalus (Boophilus) microplus (Canestrini), a vector of bovine babesiosis and anaplasmosis, is endemic in Mexico. The Cattle Fever Tick Eradication Program maintained by the Veterinary Services (VS) branch of the Animal and Plant Health Inspection Service (APHIS) of the U.S. Department of Agriculture uses inspection and acaricide treatment of imported cattle together with a permanent quarantine zone along the U.S.-Mexico border as a barrier to tick reentry (Graham and Hourrigan 1977, George 1996). Acaricide resistance is widespread in Mexico and potential tick reentry remains a threat to the U.S. cattle industry (Davey et al. 2003, Miller et al. 2005, Temeyer et al. 2004b). The continuing threat of tick reintroduction is further exemplified by the placement (3 July 2008) of a total area exceeding a million acres under temporary quarantine due to tick infestation of 139 premises in six south Texas counties (Hillman 2008).
Acetylcholinesterase (AChE) is the primary target of organophosphate (OP) acaricides (Lee and Bantham 1966, Fournier and Mutero 1994), such as coumaphos, used in the dip vats maintained by VS-APHIS at the border to treat Mexican cattle presented for importation (Davey et al. 2003, Temeyer et al. 2004b). Previous studies identified at least three R. microplus genes presumptively encoding acetylcholinesterases—BmAChE1 (Baxter and Barker 1998), BmAChE2 (Hernandez et al. 1999, Baxter and Barker 2002), and BmAChE3 (Temeyer et al. 2004a)—later confirmed to encode acetylcholinesterases by baculovirus expression and biochemical characterization (Temeyer et al. 2006; K.B.T. et al., unpublished). BmAChE3 mutations I48L, I54V, R86Q, V137I, I492M, and T548A (Table 1) were identified previously in the San Román strain of R. microplus resistant to OP acaricide (Temeyer et al. 2007). Recombinant BmAChE3 containing the R86Q mutation was shown to exhibit nearly 20-fold reduction in the rate of phosphorylation by paraoxon relative to the wild-type sequence and was found in resistant laboratory strains at elevated frequency compared with OP-susceptible strains but was insufficient to alone generate the OP-resistant phenotype. Recombinant BmAChE3 constructs containing various combinations of mutations I48L, I54V, V137I, I492M, and T548A expressed in the baculovirus system exhibited reduced AChE activity that precluded biochemical characterization in crude preparations. Here, we report development of molecular assays for the BmAChE3 mutations I48L, I54V, R86Q, V137I, I492M, and T548A. These assays were used to genotype individual tick larvae from laboratory strains and (field-collected) Mexican ticks of known resistance phenotypes to test association of the identified BmAChE3 mutations with phenotypic resistance to OP acaricide.
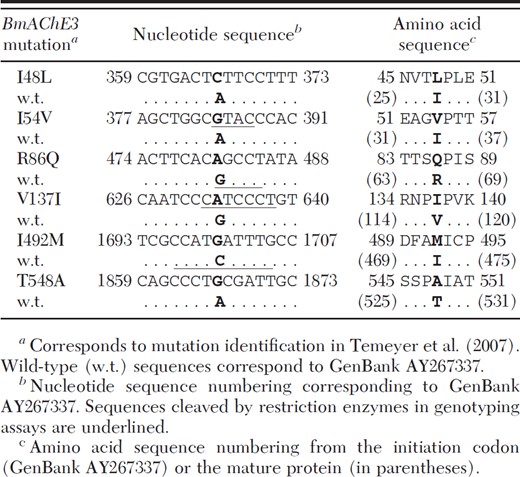
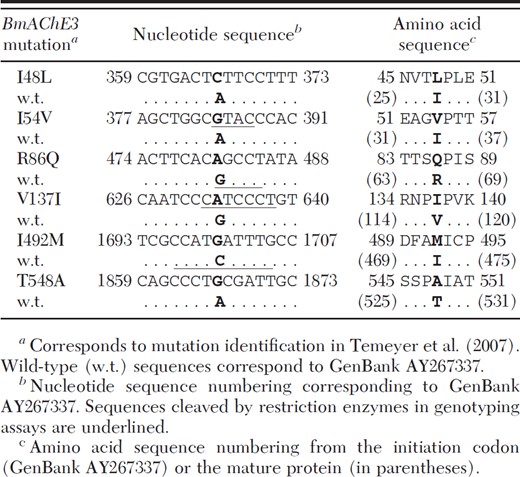
Materials and Methods
Ticks.
R. microplus laboratory strains were maintained at the Cattle Fever Tick Research Laboratory (Edinburg, TX). Laboratory-reared strains differed in their OP-resistance status (S, susceptible; R, resistant) as follows: Gonzalez (S), Muñoz (S), Deutch (S), Caporal (S), Caporal-R (R), Santa Luiza (R), Pesqueria (R), San Román (R), and Tuxpan (R). Origin and OP bioassay of the strains are described in Li et al. (2003) and Temeyer et al. (2007). Laboratory strain larvae (14–16 d) were frozen at –80°C until use. Larvae from Mexican R. microplus field collections (Table 2) were stored in ethanol (75–80%) for up to 2 wk at ambient temperature before shipment. Upon receipt, ethanol-preserved larvae were stored at 4°C until use.
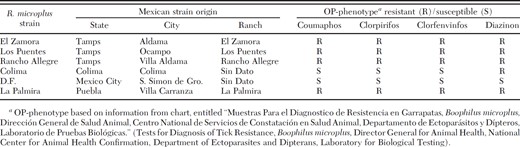
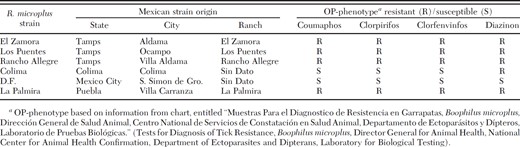
Preparation of Genomic DNA from Individual Tick Larvae.
Individual Mexican tick larvae stored in ethanol were rinsed in water (4°C), placed in prechilled 0.5 ml Kontes homogenizers on dry ice and ground to powder using pestles prechilled in liquid nitrogen, and then returned to dry ice. Extraction buffer (0.1 M Tris-HCl, pH 8.0, and 0.5 M KCl) was added (25 μl) to each ground larva, allowed to thaw on ice, reground before removal of the pestle, and the tube containing the larval extract was sealed and placed in boiling water for 8 min. Insoluble material was removed, and the supernatant was collected after centrifugation (18,000 × g) for 5 min at 4°C. Samples were stored at –20°C until use and were used directly or were diluted up to 100-fold in TEN buffer (10 mM Tris-HCl, pH 8.0, 25 mM NaCl, and 0.1 mM Na2EDTA) as template for polymerase chain reaction (PCR). Genomic DNA samples were prepared from individual tick larvae of laboratory strains by grinding in 100 μl of TEN buffer. Samples were heated 10 min at 99.5°C and centrifuged for 2 min at 14,000 × g to remove debris.
General PCR Procedure.
Genomic DNA from individual tick larvae was used in PCR amplifications as the basis for genotyping assays or sequencing. In general, 1 μl of genomic DNA preparation was added as template to 15 μl of PCR master mix [0.5 μM each primer, 50 μM each dNTP, and 0.005 U/μl of a hot-start DNA polymerase, either AmpliTaq Gold DNA polymerase (Applied Biosystems, Foster City, CA) or Jumpstart DNA polymerase (Sigma, St. Louis, MO) and incubated at 95–96°C as specified by the manufacturer to activate the polymerase, followed by 35 cycles of PCR (see indicated mutations for specific parameters), with a final incubation for 5 min at 72°C. Five microliters of the PCR mix containing the amplification product was verified by agarose electrophoresis using Hi-Lo DNA Marker (Minnesota Molecular Inc., Minneapolis, MN) or the 1 Kb Plus DNA ladder (Invitrogen, Carlsbad, CA) as size standards. Remaining PCR reaction mix was used directly for restriction enzyme digestion or for DNA sequencing as described below. In general, we set out to obtain genotype data for each of the mutations from 25 individual tick larvae; however, due to the cost and labor involved in sequencing both strands of DNA, genotyping of mutations by sequencing was generally limited to 10 individuals. It was our intention to genotype additional individuals by sequencing if the data indicated a potential association with phenotypic resistance.
Genotyping Assays for BmAChE3 Mutations.
I48L. A 201-bp fragment of BmAChE3 DNA containing the I48L site (Table 1) was amplified by PCR using primers BmAChE3–279U18 (5′-CATGCGAGATGCTACATA-3′) and BmAChE3-462L18 (5′-TGAAGTGGTCGTCGATAG-3′) and verified by electrophoresis on a 2.5% agarose gel by using Hi-Lo DNA Marker as standards. Each PCR cycle consisted of 30 s at 95°C, 30 s at 54°C, and 1 min at 72°C. PCR-amplified fragments of genomic DNA encompassing the mutation were treated with ExoSAP-IT (USB Corporation, Cleveland, OH) and were sequenced using the same primers as for PCR with BigDye terminator (Applied Biosystems) and analysis on an ABI3130XL Genetic Analyzer (Applied Biosystems) according to the manufacturer's instructions.
I54V. A segment of BmAChE3 DNA containing the I54V site was amplified by PCR using the same primers and PCR conditions as for I48L. Five microliters of the PCR mix containing the 201-bp amplification product was incubated for 2 h at 37°C with 5–10 U of RsaI restriction endonuclease (GT/AC, New England Biolabs, Ipswich, MA) in the supplier-recommended buffer. The PCR product produced from the I54V mutant template is cleaved by RsaI (Table 1) to yield 94-bp + 107-bp digestion products. The PCR product produced from the wild-type template is not cleaved by RsaI. Products of the restriction digestion were analyzed by electrophoresis on a 3.5% Metaphor agarose (Lonza Rockland, Inc., Rockland, ME) gel by using Hi-Lo DNA Marker as standards (Fig. 1).
![I54V genotyping assay. Lane 1, Hi-Lo DNA marker size standards (band sizes in bp labeled at left); lane 2, homozygous I54V mutant (94-bp + 107-bp bands); lane 3, homozygous wild-type sequence at I54 (201-bp band not cut by RsaI); and lane 4, heterozygous individual (201 bp + [107 bp + 94 bp]).](https://oup.silverchair-cdn.com/oup/backfile/Content_public/Journal/jme/46/6/10.1603_033.046.0614/2/m_jmedent46-1355-fig1.jpeg?Expires=1750305806&Signature=NXVrNqlLEmUEd52FsTUDjgkpERgUqKuJwWXSwSPJ-uc6GeflIZrkmMn4aQXLahtArhzv8pWkzRkUprwNnpFZvdcX5hqUZbN5Bja7mpj4SWk3fowo3VonBFEOXsjvoVRqUaXZmEgh34aAs2PR1Yr1HPsMns-tvS4wVuxT6lyLz71pnYcxH6wCwQLW7jDkm3m4Zr8rLFnCzpLO3L5bWFFTX1eWGMgRy8VXCJAEFKxiv0kzdI~FBu1cBPRQhY32ZEf1-2S2fOlO7ulNhalvRZs5WIyPdgeI8OKVD3Cj2kNrnEylELWKpzeFHrFEKY0ZNsU419xcAHjNdAoKpkp0omQtrA__&Key-Pair-Id=APKAIE5G5CRDK6RD3PGA)
I54V genotyping assay. Lane 1, Hi-Lo DNA marker size standards (band sizes in bp labeled at left); lane 2, homozygous I54V mutant (94-bp + 107-bp bands); lane 3, homozygous wild-type sequence at I54 (201-bp band not cut by RsaI); and lane 4, heterozygous individual (201 bp + [107 bp + 94 bp]).
R86Q. A fragment of BmAChE3 DNA containing the R86Q site was amplified by PCR using primers Ace3R86Q-277U20 (5′-CTCATGCGAGATGCTACATA-3′) and Ace3R86Q-568L17 (5′-GCCGACGAATTGGTAGA-3′) flanking R86Q and digested with HaeIII restriction endonuclease (GG/CC, New England Biolabs) as described previously (Temeyer et al. 2007). Diagnostic fragments after HaeIII digestion are 262 bp, indicating the R86Q mutation (eliminates a cleavage site for HaeIII; see Table 1), and 206 bp indicating the wild type sequence (cleaved by HaeIII).
V137I. A 476-bp fragment of BmAChE3 DNA containing the V137I site was amplified by PCR using primers BmAChE3-545U21 (5′-GGTATACGCCGCAAATAGGAC-3′) and BmAChE3–1002L19 (5′-TGGCCAGAAGCTGATCGTG-3′) and verified by electrophoresis on a 1% agarose gel. Each PCR cycle consisted of 45 s at 95°C, 45 s at 58°C, and 1 min at 72°C. Five microliters of the PCR mix containing the 476-bp amplification product was incubated for 2 h at 50°C with BtsCI restriction endonuclease (GGATG/NN, New England Biolabs) in the supplier-recommended buffer. BtsCI cleavage of the mutant sequence (Table 1) results in digestion products of 87 bp and 389 bp. The wild-type sequence is not cleaved by BtsCI. Products of the restriction digestion were analyzed by electrophoresis on a 2% agarose gel using 1 Kb Plus DNA ladder as standards (Fig. 2).
![V137I genotyping assay. Lane 1, homozygous V137I mutant (389 bp + [87 bp, not visible]); lane 2, heterozygous V137/V137I individual (476 bp + 389 bp [+ 87 bp]); lane 3, Hi-Lo DNA marker size standards (band sizes in base pairs labeled at right); and lane 4, homozygous V137 individual (wild type 476 bp not cut by BtsCI).](https://oup.silverchair-cdn.com/oup/backfile/Content_public/Journal/jme/46/6/10.1603_033.046.0614/2/m_jmedent46-1355-fig2.jpeg?Expires=1750305806&Signature=m5fhcGEy4xpKcve-nXxFF4UM04N-lOoBcbyaqXetJjkv9a7-sK9LChFvefRuGut61srEENlcCC6RM4mieNop7OnteS0aDppUhq2tdUmWY5QGVuYMrYeAACRpLLaME9uAlzXien9YQ237Z54ZpeaVoQ2z0WD5u-2s8eu4GisZq0QQ1JB87XdmjWE7qj8Qb1QgzaDJsx6bTvQQEN3l0aSau4VDxLvJ2Iyo3ecAoX6l99bSh~3Yik5xND8SoRb6TdIhcvf3bo3SFSZs0ebLitUyr4-wE0T1tZyI1yIFMSEhQm8OOImRFyL5U4SKrVxhpvyIw8Lcqubyz3ck4d85MZftyw__&Key-Pair-Id=APKAIE5G5CRDK6RD3PGA)
V137I genotyping assay. Lane 1, homozygous V137I mutant (389 bp + [87 bp, not visible]); lane 2, heterozygous V137/V137I individual (476 bp + 389 bp [+ 87 bp]); lane 3, Hi-Lo DNA marker size standards (band sizes in base pairs labeled at right); and lane 4, homozygous V137 individual (wild type 476 bp not cut by BtsCI).
I492M. A 159-bp fragment of BmAChE3 DNA containing the I492M site was amplified by PCR using primers BmAChE3–1640U20 (5′-TGAACGCGAGAACATCAAGG-3′) and BmAChE3R-1781L18 (5′-GGCCAAGTACTGAACGAC-3′) and verified by electrophoresis on a 2.5% agarose gel. Each PCR cycle consisted of 15 s at 94°C, 30 s at 60°C, and 1 min at 68°C. Five microliters of the PCR mix containing the 159-bp amplification product was incubated for 2 h at 37°C with BccI restriction endonuclease (CCATCNNN/N, 37°C, NEB1, New England Biolabs) in the supplier-recommended buffer. Products of restriction digestion were analyzed by electrophoresis on a 3% Metaphor agarose gel using Hi-Lo DNA Marker as standards. BccI cleaves the 159-bp amplification product of the wild-type template (Table 1; Fig. 3) into two fragments of 65 bp and 94 bp. The I492M mutation prevents cleavage by BccI.
![I492M genotyping assay. Lanes 1 and 5, Hi-Lo DNA marker size standards (band sizes in base pairs labeled at right); lane 2, homozygous I492 wild-type individual (65 bp + 94 bp); lane 3, heterozygous I492:I492M individual (159 bp + [65 bp + 94 bp]); and lane 4, homozygous I492M mutant individual (159 bp not cut by BccI).](https://oup.silverchair-cdn.com/oup/backfile/Content_public/Journal/jme/46/6/10.1603_033.046.0614/2/m_jmedent46-1355-fig3.jpeg?Expires=1750305806&Signature=ghTx2GF0lQQPIhSvlT0~ZkhY6TEfWVnFazvdwFb-do-XjMbPS5T5LBw~MC3ug0B3UkT96Q094XiO~5ktKW4UCAnV6lu5jgk7BKfX7DFxRRSpyQ5F-PLhzDJHG3t7I1EvddyCj0g7-zvIZFpTmw2I31jvIHiOq6cKO0Qc2xTK4Ng4H3ElkdoAqLZHkkhaNBT0I6CnKeP0B2~juSudcRugIus-nsu6-7HzRKMKQm6gRsNNHuKK8pegXdToI476AyezVv2dP3VFqQ7EHk9dqvfeSM9-7J36lvTwZrFtCgKsg8YplBl-3jjQqfrinTELSykLW4z8sAygq8d5HPU8IO9MQw__&Key-Pair-Id=APKAIE5G5CRDK6RD3PGA)
I492M genotyping assay. Lanes 1 and 5, Hi-Lo DNA marker size standards (band sizes in base pairs labeled at right); lane 2, homozygous I492 wild-type individual (65 bp + 94 bp); lane 3, heterozygous I492:I492M individual (159 bp + [65 bp + 94 bp]); and lane 4, homozygous I492M mutant individual (159 bp not cut by BccI).
T548A. A 298-bp fragment of BmAChE3 DNA containing the T548A site was amplified by PCR using primers BmAChE3–1637U17 (5′-TGGTGAACGCGAGAACA-3′) and BmAChE3–1917L18 (5′-TTTCGCGAATGTTGTCCA-3′) and verified by electrophoresis on a 2.5% agarose gel. Each PCR cycle consisted of 30 s at 95°C, 30 s at 60°C, and 15 s at 72°C. PCR-amplified fragments of genomic DNA encompassing the mutation were sequenced using the amplification primers as described above for the I48L mutation.
Data Analysis.
Results of the individual genotyping assays are presented as diploid genotypes or as mutant frequencies in the assayed population calculated as (number of mutant alleles in population assayed) ÷ (total number of alleles in population assayed) = mutant frequency. We interpreted the presence of two clear peaks at the position of the mutation on sequencing chromatograms, or partial restriction digestion of target DNA as indicative of a heterozygote. Because of the uncertainty of dominance relationships of the various mutants, the proportion of individuals containing at least one mutant allele also is presented.
Results and Discussion
Individual tick larvae from R. microplus laboratory strains, or produced by engorged females collected in Mexico (Table 2) were genotyped for the presence of mutations identified previously in BmAChE3 as potentially associated with resistance to organophosphate acaricide (Temeyer et al. 2007). In general, the PCR-based restriction enzyme assays and sequencing were robust and relatively easy to perform. It was necessary, however, to ensure that the restriction digestions were complete. This was accomplished by addition of fresh restriction enzyme after the initial digestion and reincubation to verify by comparison that the original digestion was complete. We routinely used excess enzyme and extended incubation times to allow complete digestion of the PCR products. Results of mutation-specific genotyping assays or sequencing are summarized in Table 3.
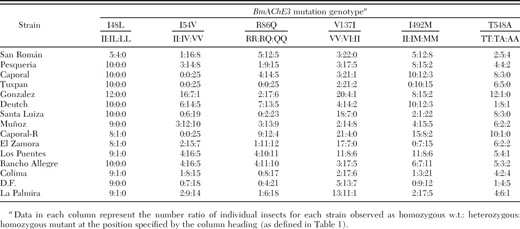
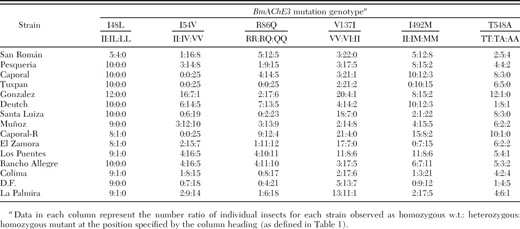
We expected that if any of the mutations (or a combination of mutations) was responsible for generation of the OP-resistant phenotype, that such mutations would be present at increased frequency in OP-resistant strains relative to susceptible strains. Table 4 presents mutant allele frequency as well as the proportion of individuals in each strain that possess at least one copy of the mutant allele (in parentheses) because the dominance relationships of individual mutations are not known. Ordering of the strain-specific data according to frequency for any of the listed BmAChE3 mutations did not produce separation or clustering of the OP-susceptible and OP-resistant phenotypes but rather resulted in a mixture of OP-R and OP-S phenotypes. Ordering strain data by proportion of individuals possessing a selected mutation also did not result in separation or clustering of the OP-phenotypes. Inspection of genotyping results for specific populations revealed a substantial fraction of individuals that were homozygous for each of the individual BmAChE3 mutations (except I48L) within at least one of the laboratory OP-susceptible strains, Gonzalez, Muñoz, and Deutch (Table 4), suggesting these mutations alone did not generate the OP-R phenotype. The BmAChE3 mutation I48L was infrequent or absent in all strains.
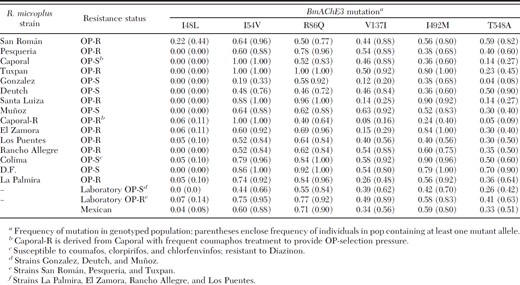
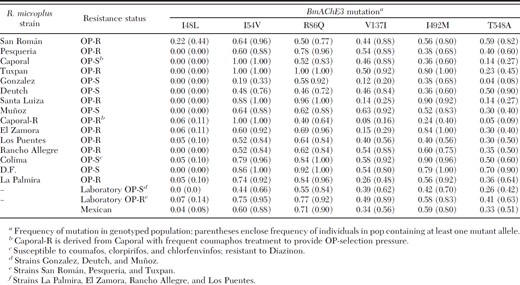
Mutant allele frequencies listed in Tables 3 and 4 are based on the assumption of two alleles per diploid individual. In addition, there are six different mutation sites within BmAChE3 presented here. Interaction between various mutation sites within the same gene product has been reported for Drosophila and other insect AChEs, and their effects were shown to be additive with respect to resistance (Villatte et al. 2000, Fournier 2005). Presumably, if a combination of BmAChE3 mutations was responsible for generating the OP-resistant phenotype, one would expect a substantial accumulation of those mutations in the OP-resistant strains where resistance is the result of target site insensitivity (OP-insensitive AChE), as has been reported for strains Tuxpan (Wright and Ahrens 1988), San Román, and Pesqueria (Pruett and Pound 2006). Comparison of the data for the OP-S strains with that of the OP-R strains reveals no clear differences in mutation frequency or content among these strains (Tables 3 and 4).
Unlike Drosophila, R. microplus contains at least three different AChEs. Failure of R. microplus BmAChE3 mutations to exhibit increased frequency in resistant strains does not indicate that they do not decrease OP-sensitivity of BmAChE3 but merely that they do not provide sufficient selective advantage to increase survival of the cohort possessing them. This failure could result from greater sensitivity of another AChE, making that AChE the primary subject of OP selection. It is also important to note that genes other than those encoding AChE may also be responsible for OP-resistance, such as esterases, mixed function oxidases, or cytochrome P450s (Hernandez et al. 2000; Chen et al. 2001; Li et al. 2003, 2005; Baffi et al. 2007). Failure to show a relationship of the OP-R strain phenotype with any of the BmAChE3 mutations suggests that none are directly responsible for primary AChE OP-insensitivity resulting in generation of the OP-resistant phenotype.
Acknowledgements
We thank Chastity Balero, Greta Buckmeier, and Kristie Schlechte for excellent technical assistance. We also thank Consuelo Almazán for providing the Mexican tick larvae used in this study.
References Cited