-
PDF
- Split View
-
Views
-
Cite
Cite
William E. Walton, Alex R. Van Dam, David A. Popko, Ovipositional Responses of Two Culex (Diptera: Culicidae) Species to Larvivorous Fish , Journal of Medical Entomology, Volume 46, Issue 6, 1 November 2009, Pages 1338–1343, https://doi.org/10.1603/033.046.0612
- Share Icon Share
Abstract
Species-specific differences in the responses of egg-laying mosquitoes (Diptera: Culicidae) to the presence of fish exudates in oviposition sites in laboratory bioassays have been related to the likelihood of encountering mosquito-eating fish in natural oviposition sites. We examined the responses of egg-laying mosquitoes to the presence of larvivorous fish in oviposition sites to test this hypothesis in the field. The number of Culex tarsalis Coquillett egg rafts laid on mesocosms (15.5 m2; 8 m3) containing caged mosquitofish (0.066 Gambusia affinis per liter) was reduced by 84% relative to mesocosms lacking fish. Egg-laying Culex quinquefasciatus Say did not differentiate significantly between comparatively small (0.11 m2) oviposition sites containing water conditioned with mosquitofish (0.3 G. affinis per liter) versus aged reservoir water. Cx. quinquefasciatus egg rafts were not collected from the larger mesocosms, and Cx. tarsalis egg rafts were rarely collected from the smaller oviposition sites. Oviposition preferences for characteristics of aquatic habitats that lack fish (e.g., small size, semipermanence, and high levels of organic enrichment in which hypoxic conditions are prevalent) may limit the coexistence of immature stages of some mosquito species such as Cx. quinquefasciatus and insectivorous fish. Mosquito species such as Cx. tarsalis that also use comparatively large water bodies as developmental sites may have evolved the ability to detect the presence of predatory fish.
Direct interactions and indirect effects between organisms on different trophic levels (Kerfoot and Sih 1987, Wellborn et al. 1996) and within a trophic level (Blaustein and Chase 2007) are important selective agents generating patterns in community structure of aquatic ecosystems. In addition to the strong, direct effects of prey consumption by predators for structuring freshwater lentic communities, indirect effects resulting from consumer-mediated trophic interactions and from predator-induced changes in prey individuals can significantly influence the composition of aquatic communities (Sih et al. 1985, Kerfoot and Sih 1987). The interaction of biotic effects and physical factors (i.e., permanence and winter anoxia) determines fitness tradeoffs of life history traits in individuals along the environmental continuum from small ephemeral ponds to large permanent lakes (Wellborn et al. 1996, Chase and Knight 2003).
The aquatic stages of mosquitoes are found in a variety of habitats ranging in size from small containers (i.e., treeholes, phytotelmata, jars, and tires) and water-filled depressions (i.e., hoofprints) to edges of large water bodies, such as lakes and emergent vegetation in wetlands. Many mosquito species are found in particular habitat types within this continuum of habitat size, but habitat preferences also are linked to other factors, including levels of organic enrichment and hydrological periodicity (i.e., floodwater or ephemeral habitats versus permanent pools; Laird 1988). Gravid female mosquitoes use a combination of environmental cues, including chemical, physical, and biological cues, to select oviposition sites (Benzon and Apperson 1988, Bentley and Day 1989, Isoe and Millar 1995).
Chemical cues produced by predators and by conspecific mosquitoes attacked by predaceous insects have been shown to reduce oviposition by some mosquito species (Sih et al. 1985, Blaustein 1999). Egg-laying mosquitoes have been shown to reduce oviposition into habitats containing planktonic hemipteran predators (Notonecta:Chesson 1984, Kiflawi et al. 2003, Blaustein et al. 2005; and Anisops:Eitam et al. 2002). The abundance of mosquito eggs in soil adjacent to pools containing fish was lower than in the soil adjacent to pools lacking fish (Ritchie and Laidlaw-Bell 1994). Although oviposition by mosquitoes was not measured, the abundance of immature mosquitoes in pools containing caged fish (Petranka and Fakhoury 1991) or water conditioned using fish (Angelon and Petranka 2002) was lower than in pools lacking fish. These findings suggest a reduction of mosquito oviposition by the detection of kairomones produced by fish; however, mechanical and visual cues of predator presence in prospective larval mosquito habitats also may allow ovipositing female mosquitoes to detect predation risk or changes in other factors associated with the presence of fish (e.g., predatory insect abundance or community composition) may influence the survival of immature mosquitoes. Van Dam and Walton (2008) recently showed in laboratory studies that the response of three mosquito species to the presence of fish exudates in oviposition sites was related to the likelihood of encountering fish in natural oviposition sites. Here, we examine the responses of egg-laying mosquitoes to the presence of fish by using the mosquitofish, Gambusia affinis (Baird and Girard), as a representative larvivore to test this hypothesis in the field.
Materials and Methods
The effect of fish on oviposition by Culex mosquitoes was tested in small and large oviposition sites at the University of California (UCR) Aquatic Research Facility, Riverside, CA. Field trials using small oviposition sites (wash tubs) were conducted during the vernal and autumnal periods of peak Culex egg laying activities. Experiments were carried out for 10 d starting on 18 June (spring study) and for 11 d starting on 26 September 2006 (autumn study). An experiment using comparatively large earthen mesocosms was carried out from 17 June through 13 July 2007. The collection and handling of mosquitofish was done under Protocols A-0405013-2 and A-20070008 approved by the Institutional Animal Care and Use Committee of the University of California-Riverside.
Small Oviposition Sites.
Four plastic wading pools (1.3 m in diameter by 0.15 m in depth) were used to condition water for the trials by using the wash tubs as oviposition sites. Forty G. affinis were held in a wading pool (the holding pool) and fed daily an artificial diet of fish flakes (Tetra Pond, Tetra Holding Inc., Blacksburg, VA). To reduce the chemical cues from prey consumed recently and increase the likelihood that chemical cues emanated from the fish directly, 30 adult G. affinis were then moved into a second wading pool (gut-clearing pool) to clear their digestive tracts. After starvation for 24 h, these fish were transferred to a third wading pool, the treatment pool, in which they were allowed to swim and release chemicals for 24 h in 100 liters of water. The source of water was an irrigation supply reservoir; mosquitofish were not present in the reservoir. Every 24 h thereafter, 10 fish were selected at random from the treatment pool and returned to the holding pool, 10 fish were transferred from the holding pool into the gut-clearing pool, and 10 fish were transferred from the gut-clearing pool to the treatment pool. A fourth wading pool with 100 liters of water was simultaneously set up as a control. A 20% water change occurred daily in the treatment pool and control pool. The wading pools were covered with 1.5-mm mesh fiberglass window screen to prevent mosquitoes from laying egg rafts on the water surface and to reduce the likelihood that chemicals emanating from predation on mosquitoes were present.
Mosquito oviposition responses to the two water treatments, control or fish-conditioned, were studied using 10-liter plastic wash tubs (36 by 31 by 13 cm [height]; Rubbermaid, Freeport, IL). By distributing water derived from individual treatment pools among replicate washtubs and rotating fish through the conditioning protocol, this experimental design ignores variation among replicate groups of fish and sacrifices our ability to generalize the results across groups of fish; yet, it reduces the sources of variation and emphasizes the power to detect a response by egg-laying mosquitoes. In the spring study, wash tubs were positioned in two groups of 10 wash tubs spaced 7 m apart. Wash tubs in each group were set out in a 5 by 2 array in which a pair of wash tubs in each row was separated by 0.5 m and wash tubs in the two columns were separated by 2 m. Water treatments were randomly assigned to wash tubs such that each water treatment was replicated five times per group. Ten liters of water from the treatment pool or control pool were added to each wash tub before dusk on 18 June. Ten grams of alfalfa pellets (Nutriphase Rabbit Formula, Pacific Coast Distributing Inc., Phoenix, AZ) was added to each wash tub to induce mosquito oviposition. On each consecutive day just before dusk, water level in each tub was adjusted to 10 liters by removing water to a predetermined level and then adding 1 liter of either control or treatment water to wash tubs.
The autumn field trial used the same water conditioning protocols as the spring experiment but only 2 liters of water was added to each wash tub. Because evaporative losses during the autumn trial were less than during the spring trial, only 0.5 liter of water was replaced daily in each wash tub. Water treatments were paired and wash tubs were placed at 10 locations throughout the UCR Aquatic Research Facility. The minimum distance between pairs of wash tubs was 12 m.
Egg rafts were collected each day just before dusk and isolated in 150-ml wax lined paper cups (Solo Cup Co., Highland Park, IL). Mosquito larvae were raised to the fourth instar by using a laboratory diet [ground rat chow and brewer's yeast, 3:1 (vol:vol)] and were identified to species using Meyer and Durso (1993).
Mesocosms.
Oviposition studies in summer 2007 used six earthen mesocosms (3.7 by 7.2 m) at the UCR Aquatic Research Facility. Water was again supplied from an irrigation supply reservoir that lacked fish on the Agricultural Experiment Station at UCR. Water depth in each mescosm was maintained at ≈0.3 m by a float valve. The mesocosms had been flooded for 36 d, drawn down, and dried for 2 d and then inundated again on 17 June. The influent flow rate for each mesocosm was calculated by measuring the time required to fill a graduated 350-ml cup (n = 3) between 1400 and 1430 hours on 20 June.
Approximately 530 mixed age G. affinis (mean standard length ± SD, 1.2 ± 0.4 cm; mean wet weight per mesocosm ± SD, 180 ± 1 g) were distributed among three cages (height by width by length, 0.45 by 0.45 by 0.65 m) spaced equidistantly along the long axis of each of three replicate mesocosms on 20 June. Cages were made of fiberglass window screen (mesh opening, 1.5 mm) affixed to pine furring strips (≈0.02 m in width by 0.04 m in depth). The fish were held in the cages for 72 h in a mesocosm containing a stock fish population before placing the cages into the experimental mesocosms, and food levels in the water were not supplemented during the experimental period. Empty cages were placed in the three fishless mesocosms. The total surface area occupied by the three cages was <3% of the total surface area of each mesocosm. Fish were removed from cages on 3 July.
Mosquito egg rafts were counted twice weekly in four 1-m transects along the perimeter of each mesocosm beginning on 22 June. A transect was taken on each side of a mesocosm. On each sampling date, the starting points for transects on each major compass direction were same for all mesocosms and were determined using a random number generator. Egg rafts laid up to 0.5 m from the edge of the mesocosm were included in the counts. Once per week ≤30 egg rafts were collected from each mesocosm and isolated in 150-ml wax lined paper cups. Mosquito larvae were fed the laboratory diet, raised to the fourth instar, and identified to species using keys in Meyer and Durso (1993).
The statistical significance of differences in mosquito oviposition between treatments summed across dates at each replicate location was assessed using a paired t-test for the autumn study and by using analysis of variance (ANOVA) for the factorial design of the spring study. The statistical significance of the treatment effects on mosquito oviposition for the mesocosm study was assessed using repeated measures multivariate ANOVA (SYSTAT version 9.0, SPSS Inc., Chicago, IL). The number of egg rafts collected was transformed (loge + 1) for the autumn wash tub study and the summer mesocosm study.
Results
Small Oviposition Sites.
Egg-laying Cx. quinquefasciatus did not respond significantly to the presence of fish chemicals in wash tub ovipositional sites in both field studies (spring study: F1,16 = 0.028; P = 0.870; autumn study: paired t-test, t9 = 0.970; P > 0.35). Mosquito oviposition did not differ significantly between the two groups of tubs in the spring trial (group effect: F1,16 = 0.028; P = 0.870; group × treatment: F1,16 = 0.372; P = 0.551); however, few egg rafts [mean number of egg rafts per tub, fish-conditioned water: 1.7 (n = 17); control: 1.6 (n = 14)] were laid during the study and the large confidence limits for the difference in the number of egg rafts laid between the treatments (95% confidence interval [CI]: -1.4, 1.6) are indicative of the low statistical power of this study. More than 80 egg rafts were collected in the two treatments during the autumn study (backtransformed mean number of egg rafts per tub, fish-conditioned water: 9.0 [n = 119]; control: 6.3 [n = 82]), and this study provided a more robust statistical test of the treatment effects (95% CI for difference between the treatments: 0.6, 3.3) than did the spring study. The majority of egg rafts collected in the spring and autumn field trials (91 and 98%, respectively) were laid by Cx. quinquefasciatus.
The remaining egg rafts collected in both experiments were laid by Cx. tarsalis. Egg rafts were collected from both water treatments, but the egg rafts laid by this species in the tubs were too rare to provide meaningful statistical tests of ovipositional preference.
Mesocosms.
The number of egg rafts laid on mesocosms containing caged fish was significantly less than for mesocosms lacking fish across the four dates when fish were present (F1,4 = 13.949; P < 0.025). From 22 June through 2 July, the trends for egg laying across dates were similar in both treatments (date × treatment: Pillai Trace = 0.782; F3,2 = 2.390; P > 0.30), and egg laying did not differ significantly among dates (date: Pillai Trace = 0.838; F3,2 = 3.445; P > 0.23). The number of egg rafts collected from mesocosms containing fish was reduced by 83.6% (SE = 2.9%; n = 4) compared with the number of egg rafts collected from mesocosms without mosquitofish (Fig. 1). On average, ≈12 egg rafts were collected from each mesocosm per week to determine the mosquito species; 36% (n = 102) of the egg rafts had hatched before collection and all of the egg rafts that hatched in the laboratory (n = 179) had been laid by Cx. tarsalis.
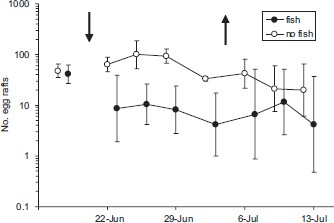
Egg rafts (backtransformed mean ± 95% CI) of Cx. tarsalis collected on four 1-m transects from replicate 28-m2 mesocosms containing caged G. affinis or lacking fish. The left arrow indicates the date that fish were added to cages and the right arrow indicates the date that fish were removed from cages. Data are offset horizontally to facilitate illustration.
Mosquito ovipositional activity before stocking fish and after removing the mosquitofish (Fig. 1) did not differ significantly between the mesocosms assigned to the two treatments (prestocking: F1,4 = 0.332; P > 0.59; after removal: F1,4 = 1.713; P > 0.26). After removing the fish, the trends for egg laying by Cx. tarsalis across dates were similar in both treatments (date × treatment: Pillai Trace = 0.534; F2,3 = 1.717; P > 0.31) and did not differ significantly among dates (date: Pillai Trace = 0.281; F2,3 = 0.585; P > 0.61).
The influent flow rate did not differ significantly between mesocosms assigned to the two treatments (mean ± SE, 34.1 ± 2.6 ml s-1, n = 6) (F1,4 = 0.182; P > 0.69). Water turnover time was estimated to be ≈3 d (2.8 ± 0.6 d; n = 6).
Discussion
Oviposition preferences for characteristics of aquatic habitats that lack fish, such as small size, semipermanence, and high levels of organic enrichment where hypoxic conditions are prevalent, may limit the coexistence of immature stages of some mosquito species and fish. Larvae and pupae of Cx. quinquefasciatus are typically found in organically enriched environments such as cisterns, underground drains, overflows from septic systems, dairy wastewater ponds, and in small containers such as cemetery urns containing decaying vegetation (Bohart and Washino 1978, Meyer and Durso 1993). These habitats typically lack fish. In nature, Cx. quinquefasciatus females did not reduce egg laying on organically enriched sites containing water conditioned with fish relative to sites enriched with organic matter and without fish exudates. The enhancement of Cx. quinquefasciatus oviposition from chemical cues derived from the decomposition of the alfalfa pellets might have superseded any potential negative effects on oviposition from fish kairomones in our field experiments. In laboratory studies, Cx. quinquefasciatus reduced oviposition by 25% onto cups containing water conditioned with high fish densities (0.5 fish per liter, without organic enrichment), but egg-laying females did respond consistently to the presence of fish exudates in oviposition cups (Van Dam and Walton 2008).
In contrast to Cx. quinquefasciatus, Cx. tarsalis may have evolved a comparatively strong aversion to lay egg rafts on habitats containing fish. Cx. tarsalis larvae can be found in fishless aquatic habitats including rain barrels and hoofprints in corrals (Carpenter and La Casse 1955), but they are more commonly found in comparatively large water bodies such as ground pools, standing water associated with agricultural use such as poorly drained pastures and rice fields, and in the emergent vegetation of wetlands and sumps where fish predators are often found (Bohart and Washino 1978). Cx. tarsalis egg laying on mesocosms containing caged mosquitofish (≈0.07 mosquitofish per liter) was reduced by 84% relative to oviposition on control mesocosms. It is possible that egg laying by mosquitoes was deterred by the movements of fish within the cages, but we feel this effect was unlikely to have been significant. First, the total surface area of the three cages in each mesocosm was only 3% of the surface area of the mesocosm and the mosquitofish enclosed in the cages did not have direct access to egg-laying mosquitoes outside the cages, especially along the periphery of the mesocosms. Second, the trends for oviposition on the mesocoms are consistent with Cx. tarsalis ovipositional behavior in the laboratory (Van Dam and Walton 2008) where egg laying on water conditioned with fish was reduced nearly four-fold, even though the fish density (0.5 mosquitofish per liter) used to condition the water was much higher than in the mesocosms. Whereas moderate levels of organic enrichment enhance egg laying by gravid Cx. tarsalis (Isoe and Millar 1995, Du and Millar 1999), the strong and consistent reduction of oviposition in response to planktivorous fish suggests the importance of predation as a selective agent for oviposition site selection across the variety of developmental sites used by this species.
Differences in mosquito oviposition between the two treatments disappeared by 3 d after the fish were removed from earthen mesocosms and were consistent with mean turnover time of the water. However, the effect of predator kairomones on the behavior of sensitive organisms disappeared after 2-4 d (Petranka and Fakhoury 1991, Blaustein et al. 2005) or 7-8 d (Blaustein et al. 2004) in plastic tubs or wading pools without a continuous supply of water, probably due to degradation of the kairomones.
A general lack of morphological defenses and limited behavioral responses in larval and pupal mosquitoes against predators favors traits that reduce the temporal and spatial overlap of immature mosquitoes and their predators. The antipredator responses of immature mosquitoes to chemicals released by predators or damaged conspecifics include fleeing or reducing activity (Sih 1986, Kesavaraju et al. 2007, Ferrari et al. 2008), but the potential spatial separation of immature mosquitoes and their co-occurring predators is limited compared with the closely related phantom midges (Chaoborus). Lake-dwelling chaoborid species that co-occur with planktivorous fish show both decreased oviposition (Berendonk 1999) and altered spatial distribution in the water column when fish kairomones are present in the environment (Dawidowicz et al. 1990, Tjossem 1990, Berendonk and O'Brien 1996). Whereas vertical migration of some Chaoborus species permits coexistence with planktivorous fish in lakes, movement into the profundal zone is not possible for the immature stages of mosquitoes because they respire primarily using atmospheric oxygen and are restricted to shallow aquatic habitats. Traits that limit the temporal and spatial overlap between immature mosquitoes and their predators include rapid colonization of ephemeral, semipermanent, or hypereutrophic habitats where predators are rare (Walton et al. 1990, Chase and Knight 2003), rapid immature development especially in species commonly found in ground pools (Barrera and Medialdea 1996) where large populations of predators develop comparatively late in succession, and the propensity of mosquito larvae to be found in regions of habitats (i.e., dense emergent vegetation) with comparatively reduced risks of predation. The ability of some egg-laying mosquitoes to detect the presence of predators in oviposition sites is an important indirect effect of fish (Ritchie and Laidlaw-Bell 1994, this study) and insect (Chesson 1984; Blaustein et al. 2004, 2005) predators that influences the spatial distribution of mosquito species.
Acknowledgements
We thank R. T. Cardé, M. S. Mulla, B. A. Mullens, S. A. Juliano, the Medical-Veterinary Entomology discussion group, and two reviewers for comments on an early version of the manuscript. D. Beasley, J. Haug, N. Nguyen, and T. J. Walton provided invaluable assistance in the field. This work was supported by the Agricultural Experiment Station at UCR, Special Funds for Mosquito Research from the Division of Agriculture and Natural Resources of the University of California, the Coachella Valley Mosquito and Vector Control District, and USDA grant S-1029. A portion of this work was carried out in partial fulfillment of an M.S. from the Department of Entomology, University of California-Riverside (to A.R.V.).
References Cited