-
PDF
- Split View
-
Views
-
Cite
Cite
Michael J. Turell, Christopher N. Mores, David J. Dohm, Won-Ja Lee, Heung-Chul Kim, Terry A. Klein, Laboratory Transmission of Japanese Encephalitis, West Nile, and Getah Viruses by Mosquitoes (Diptera: Culicidae) Collected near Camp Greaves, Gyeonggi Province, Republic of Korea, 2003 , Journal of Medical Entomology, Volume 43, Issue 5, 1 September 2006, Pages 1076–1081, https://doi.org/10.1093/jmedent/43.5.1076
- Share Icon Share
Abstract
We conducted experimental studies to evaluate mosquitoes captured in Paju County, Gyeonggi Province, Republic of Korea, for their ability to transmit West Nile virus (family Flaviviridae, genus Flavivirus, WNV), Japanese encephalitis virus (family Flaviviridae, genus Flavivirus, JEV), and Getah virus (family Togaviridae, genus Alphavirus, GETV) under laboratory conditions. Both Culex pipiens pallens Coquillett and Culex tritaeniorhynchus Giles were highly susceptible to infection with WNV, with infection rates >65% when allowed to feed on chickens with viremias of ≈107 plaque-forming units (PFU) of virus/ml blood. In contrast, Cx. tritaeniorhynchus were significantly more susceptible to JEV or GETV (infection rate 100%) than were the Cx. p. pallens (infection rate 3% for JEV and 0% for GETV) captured in the same area when allowed to feed on chickens with viremias of ≈105 PFU of virus/ml blood. The detection of JEV in field-collected Cx. tritaeniorhynchus in Gyeonggi Province in 2000 and the demonstrated ability of this species to transmit this virus support the importance of the continued vaccination of Koreans against JEV and indicate a risk of infection for nonvaccinated individuals.
Japanese encephalitis virus (JEV) and West Nile virus (WNV) are both members of the JEV serogroup (family Flaviviridae, genus Flavivirus). Although most infections in humans with either of these viruses produce little or no clinical illness, infection with either of these viruses can cause life-threatening encephalitis (Burke and Leake 1988, Mackenzie et al. 2004). Japanese encephalitis virus is enzootic in Asia from western Nepal to Korea and Japan and has been responsible for outbreaks of encephalitis in humans, with thousands of cases being reported each year (Burke and Leake 1988, Sohn 2000, Vaughn and Hoke 1992). In the Republic of Korea (ROK), a mandatory vaccination program was initiated in the early 1980s, and this program has significantly decreased the numbers of human cases. The incidence of Japanese encephalitis was reduced from >18.5/100,000 people in 1964 to <0.02/100,000 people since 1985 (Sohn 2000). Although not responsible for as many deaths, WNV is known to occur over a wide geographic area. This virus is an emerging pathogen and was documented for the first time in the American continent in 1999 (CDC 1999, Lanciotti et al. 1999). Since 1999, infection with WNV has been responsible for >15,000 human infections and >500 human fatalities in North America (CDC 2002, 2003, 2004).
Both of these viruses are transmitted by mosquitoes and use birds as amplifying hosts (Burke and Leake 1988, Hayes 1989). Despite the small number of recent human cases of JE in the ROK, the detection of JEV in Culex tritaeniorhynchus Giles and Getah virus (family Togaviridae, genus Alphavirus, GETV) (a virus known to cause illness in horses and humans; Lundstrom 1999) in Aedes vexans (Meigen) mosquitoes captured in Gyeonggi Province, ROK, in 2000 (Turell et al. 2003), indicates a potential risk for human exposure to these viruses in the ROK. Although Cx. tritaeniorhynchus is the known vector of JEV throughout much of its range and is the expected vector in the ROK (Baik and Joo 1991), Cx. tritaeniorhynchus from Korea have not been tested for their ability to transmit JEV, WNV, or GETV. Numerous studies have shown that the ability of a mosquito species to transmit a particular virus can vary greatly for different geographical populations (Gubler and Rosen 1976, Hardy et al. 1976, Takahashi 1980). Similarly, although members of the genus Culex have been incriminated as vectors of WNV (Hayes 1989, Hubalek and Halouzka 1999), Korean Culex pipiens pallens Coquillett have never been evaluated for their ability to transmit this virus. Therefore, we evaluated Cx. tritaeniorhynchus and Cx. p. pallens captured in Gyeonggi Province, ROK, for their ability to transmit WNV, GETV, and JEV under laboratory conditions.
Materials and Methods
Mosquitoes
Adult female mosquitoes were captured in Mosquito Magnet traps (American Biophysics Corporation, North Kingstown, RI) in August 2003 in the vicinity of Camp Greaves, located west of Tongil-Chon, just south of the demilitarized zone (DMZ) (37° 54′ N, 126° 43′ E), Gyeonggi Province, ROK. Captured mosquitoes were transported to a biological safety level 3 laboratory (with HEPA-filtered exhaust air, treated sewage, and a 100% clothing change) at the United States Army Medical Research Institute of Infectious Diseases. They were then provided apple slices as a carbohydrate source and held at 26°C for 7–10 d until exposed to viremic chickens. In addition to the field-collected female mosquitoes, first generation progeny of these mosquitoes also were used in these studies. Voucher specimens were deposited at the National Museum of Natural History, Smithsonian Institution, Washington, DC, and their identity was confirmed by microsatellite genetic analysis (D. Fonseca, unpublished data).
Virus and Virus Assays
We used a strain of JEV (ROK-2.0028) and GETV (ROK-2.0017) that had been isolated from Cx. tritaeniorhynchus and Ae. vexans, respectively, captured near Camp Greaves in 2000 and passaged twice in African green monkey kidney (VERO) cells before use in this study (Turell et al. 2003). We also used a strain of WNV (crow 397–99) from the brain of a crow that died in the Bronx, NY, in September 1999 (Turell et al. 2000). This strain had been passaged twice in VERO cells before use in this study.
To determine infection status, specimens were serially diluted in diluent (10% heat-inactivated fetal bovine serum [FBS] in medium 199 with Earle's salts, NaHCO3, and antibiotics) and tested for the presence of virus on VERO cell monolayers by plaque assay. Procedures for plaque assay were similar to those described by Gargan et al. (1983) except that the overlay containing neutral red was added 2 d (WNV and GETV) or 4 d (JEV) after the initial assay. Plaques were counted the next day.
Vector Competence Studies
We previously determined that WNV- and JEV-inoculated 1-d-old Leghorn chickens, Gallus gallus L., developed viremias of ≈107 and 105 plaque-forming units (PFU)/ml, respectively, 2–3 d after infection (Turell et al. 2000, 2005). Preliminary studies with GETV indicated that 1-d-old Leghorn chickens also developed a viremia ≈105 PFU/ml 2–3 d after infection (M.J.T., unpublished data). Therefore, mosquitoes were allowed to feed on 2- to 4-d-old Leghorn chickens that had been inoculated with 102–3 PFU of JEV, GETV, or WNV 2–3 d earlier. Immediately after mosquito feeding, 0.1 ml of blood was obtained from the jugular vein of each chicken. This was added to 0.9 ml of heparinized diluent, and the blood suspensions were frozen at −70°C until tested for virus by plaque assay to determine the viremias at the time of mosquito feeding. After exposure to the viremic chickens, engorged mosquitoes were transferred to 3.8-liter screen-topped cardboard cages held at 26°C at a photoperiod of 16:8 (L:D) h. After an incubation period of ≥12 d, most mosquitoes were allowed to refeed on 1- to 2-d-old chickens either individually or in small groups to determine whether they could transmit virus by bite. Immediately after the transmission attempt, the mosquitoes were killed by freezing, identified to species, feeding status determined, and their legs and bodies triturated separately in 1 ml of diluent. Infection was determined by recovery of virus from the mosquito tissue suspension. If virus was recovered from its body, but not its legs, the mosquito was considered to have a nondisseminated infection limited to its midgut. In contrast, if virus was recovered from both the body and leg suspensions, the mosquito was considered to have a disseminated infection (Turell et al. 1984). We defined the infection and dissemination rates as the percentages of mosquitoes tested that contained virus in their body or legs, respectively. Chickens used in the transmission attempts were bled from the jugular vein 1 d (WNV) or 2 d (JEV of GETV) after mosquito feeding and the blood handled as described above. Recovery of virus from this blood indicated transmission. Because some of the mosquitoes were tested for transmission in small pools, it was not always possible to determine which mosquito in a pool actually transmitted virus by bite. Therefore, if more than one mosquito with a disseminated infection fed in a pool, data from that pool were not used to calculate the transmission rate, regardless of chicken viremia. Some of the mosquitoes also were tested for their ability to transmit virus to diluent in a capillary tube (Aitken 1977). Briefly, mosquitoes were chilled in a glass container in wet ice, their legs were removed and triturated for virus testing, their wings were removed, and the body was placed on its side on sticky tape. A glass capillary tube containing ≈10 μl of diluent (fortified to 50% heat-inactivated FBS) was placed so that the mosquito's proboscis was inserted into the diluent. Thirty minutes later, the diluent was expressed into 500 μl of diluent, and the mosquito's body was triturated for virus testing. The diluent, containing the expressed saliva, was tested in triplicate on six-well plates for the presence of virus. Infection and dissemination rates were compared by Fisher exact or chi-square (with Yates correction) tests at the 95% confidence level.
Research was conducted in compliance with the Animal Welfare Act and other federal statutes and regulations relating to animals and experiments involving animals and adheres to principles stated in the Guide for the Care and Use of Laboratory Animals (National Research Council, 1996). The facility where this research was conducted is fully accredited by the Association for the Assessment and Accreditation of Laboratory Animal Care International.
Results and Discussion
Viremias in the 11 chickens used to expose Cx. p. pallens to WNV (mean ± SD = 106.8 ± 0.2 PFU/ml, range 106.3–7.0) were similar to those in the three chickens used to expose Cx. tritaeniorhynchus to WNV (106.8 ± 0.2 PFU/ml, range 106.6–7.0). Likewise, viremia levels in chickens used to expose Cx. p. pallens to JEV and GETV were similar to those in the chickens used to expose Cx. tritaeniorhynchus. However, for each of these viruses, viremia levels could be defined as either low, 104.3 ± 0.2 PFU/ml, or high, 105.2 ± 0.3 PFU/ml.
West Nile Virus
After feeding on chickens with a mean viremia of 106.8 PFU/ml, both Cx. tritaeniorhynchus and Cx. p. pallens were highly susceptible to infection with WNV. However, infection rates were significantly higher in Cx. tritaeniorhynchus (100%; n = 13) than in Cx. p. pallens (66%; n = 134) (Fisher exact test; P < 0.01) (Table 1).
Infection and dissemination rates for mosquitoes orally exposed to West Nile virus
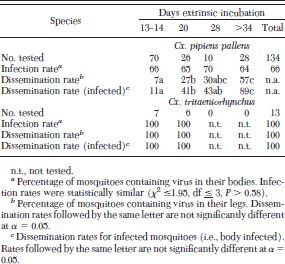
Infection and dissemination rates for mosquitoes orally exposed to West Nile virus
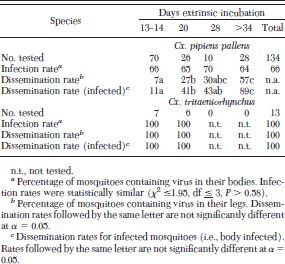
Although infection rates remained relatively constant over time in Cx. p. pallens, virus dissemination rates increased with increasing extrinsic incubation (Table 1). Only five (7%) of 70 mosquitoes tested 13 or 14 d after the infectious meal had a disseminated infection compared with 16 (57%) of 28 mosquitoes tested on or after 28 d. This difference was even more apparent if only infected mosquitoes were considered, with dissemination rates of 11 and 89%, for infected mosquitoes tested on days 13–14 and ≥28, respectively. In contrast, all 13 Cx. tritaeniorhynchus, including the seven tested on day 13 or 14, had a disseminated infection.
Mosquitoes were tested for their ability to transmit WNV either in vivo to naïve 1-d-old chickens or in vitro by the capillary tube method. All six Cx. p. pallens and the single Cx. tritaeniorhynchus with a disseminated infection that fed on a naïve chicken transmitted WNV by bite. When their proboscis was inserted into diluent in a capillary tube, we detected WNV from the expectorate of 12 (86%) of 14 Cx. p. pallens, but not from any of four Cx. tritaeniorhynchus, including one mosquito that had transmitted WNV by bite to a chicken (Table 2). The reduced transmission efficiency for WNV detected with artificial methods (e.g., capillary tube or hanging drop method for detecting transmission) compared with direct animal feeding for Cx. tritaeniorhynchus also was reported by Akhter et al. (1982). They found that 64 (93%) of 69 Cx. tritaeniorhynchus with a disseminated infection transmitted WNV when fed on a suckling mouse but that only 77 (61%) of 126 transmitted WNV to a hanging drop. This difference was significant (χ2 = 20.7, df = 1, P < 0.001) and may explain our inability to detect transmission of WNV by Cx. tritaeniorhynchus by the capillary method.
Transmission rates for mosquitoes with a disseminated infection after oral exposure to West Nile, Japanese encephalitis, or Getah virus
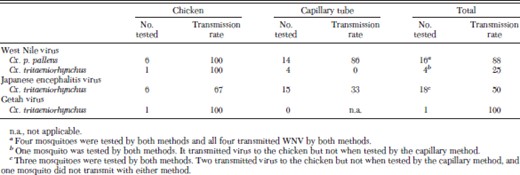
Transmission rates for mosquitoes with a disseminated infection after oral exposure to West Nile, Japanese encephalitis, or Getah virus
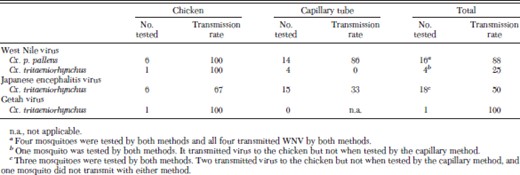
The Cx. p. pallens were competent laboratory vectors of WNV, with vector competence levels similar to those observed for North American Cx. p. pipiens (Turell et al. 2001). Cx. tritaeniorhynchus were extremely susceptible to infection with WNV, with all mosquitoes developing a disseminated infection and the only mosquito refeeding on a naïve chicken transmitting virus by bite. This is consistent with earlier reports on the ability of Cx. tritaeniorhynchus from Pakistan to transmit WNV (Akhter et al. 1982). The range of WNV has been expanding for several years, extending west into the Americas and northeast into southern Russia and many of the former Soviet Republics (Mackenzie et al. 2004). It is therefore possible that WNV may be introduced into the ROK by any of the following methods: infected mosquitoes transported in an airplane, an infected human traveling to the ROK who develops a moderate viremia, an infected bird or other animal brought into the ROK, or a migrating viremic bird. Should WNV be introduced, either Cx. p. pallens or Cx. tritaeniorhynchus would be efficient vectors and could transmit this virus in the ROK.
Getah Virus
Although none of the 40 Cx. p. pallens that fed on chickens with a mean viremia of 104.4 PFU/ml became infected, all three Cx. tritaeniorhynchus that fed on these chickens became infected (Table 3). In addition, we did not detect virus in any of 30 Cx. p. pallens that fed on chickens with a mean viremia of 105.3 PFU/ml, indicating that Cx. p. pallens is essentially refractory to infection with GETV. In contrast, all three Cx. tritaeniorhynchus not only became infected, but all three developed a disseminated infection and the only mosquito that took a bloodmeal on a naïve chicken transmitted GETV by bite. Although the sample size is too small to make a definitive statement about its ability to transmit GETV, Cx. tritaeniorhynchus seems to be an efficient laboratory vector of this virus.
Infection and dissemination rates for mosquitoes orally exposed to Getah virus
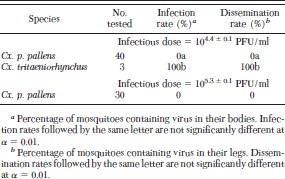
Infection and dissemination rates for mosquitoes orally exposed to Getah virus
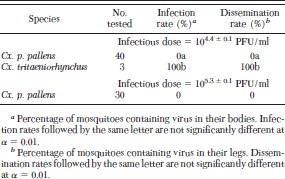
Japanese Encephalitis Virus
Only two (6%) of 32 Cx. p. pallens became infected after they had fed on chickens with a mean viremia of 105.2 PFU/ml. In contrast, all 24 (100%) Cx. tritaeniorhynchus became infected after feeding on chickens with this or a lower viremia (Table 4). Although two Cx. p. pallens became infected with JEV, they contained titers of only 102.1 and 102.2 PFU, and neither of these developed a disseminated infection. In contrast, eight (80%) of the 10 Cx. tritaeniorhynchus that fed on chickens with a mean viremia of 104.3, and 13 (93%) of the 14 Cx. tritaeniorhynchus that fed on chickens with a mean viremia of 105.2 developed a disseminated infection. Virus titers in the three Cx. tritaeniorhynchus with nondisseminated infections ranged from 104.1 to 104.6 PFU, whereas those in Cx. tritaeniorhynchus with disseminated infections were all ≥104.9 PFU.
Infection and dissemination rates for mosquitoes orally exposed to Japanese encephalitis virus
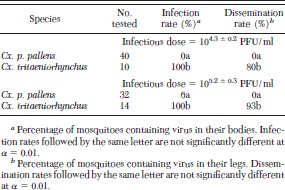
Infection and dissemination rates for mosquitoes orally exposed to Japanese encephalitis virus
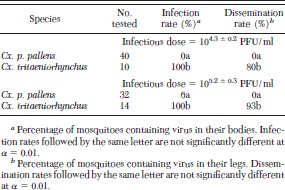
Mosquitoes were tested for their ability to transmit JEV either to naïve 1-d-old chickens or via the capillary tube method. None of the Cx. p. pallens developed a disseminated infection, and as expected, none of 13 mosquitoes tested transmitted virus. Nine (50%) of 18 Cx. tritaeniorhynchus with a disseminated infection that either fed on a naïve chicken or expectorated saliva into a capillary tube transmitted JEV by bite (four [67%] of six feeding on a chicken and five [33%] of 15 expectorating into a capillary tube) (Table 2). This included three mosquitoes tested by both methods. Although we did not detect virus in any of the saliva samples from these three mosquitoes, two (67%) transmitted JEV to 1-d-old chickens. Again, transmission rates for mosquitoes with a disseminated infection were lower for mosquitoes tested with the capillary method than for those allowed to feed on a susceptible vertebrate host. It is possible that the amount of virus expectorated into a capillary tube was less than the level of detection for plaque assay for some of the female mosquitoes. This may have been exacerbated because saliva is used to locate a blood vessel (Ribeiro et al. 1984), and more saliva may be expectorated into a vertebrate host while seeking to locate a blood vessel than into a capillary tube, where the mosquito has "found blood" almost instantly. Also, the medium used to collect the saliva (e.g., oil or aqueous) can affect the amount of virus detected (Colton et al. 2005). Therefore, studies that use an aqueous medium (e.g., diluent fortified with FBS), as we did in the current study, may underestimate the amount of virus secreted. Therefore, the capillary method may be a more conservative but a less sensitive method for determining the ability of a mosquito to transmit virus by bite. If a mosquito transmits virus during capillary feeding, it would almost certainly have transmitted virus to a susceptible vertebrate host. However, the converse may not be true, and not all mosquitoes that successfully transmit an infectious dose to a vertebrate host would transmit detectable virus when tested by the capillary tube method.
Although, Huang (1982) reported nine isolations of JEV from Cx. p. pallens in China, they concluded on epidemiological grounds that this species was probably not an important vector in China. In addition, Lee et al. (1969) isolated JEV from a pool of Cx. p. pallens captured in the ROK. However, based on our results, the Cx. p. pallens from the ROK would not be important in transmitting JEV because of the presence of both a midgut infection and midgut escape barrier (Kramer et al. 1981). In contrast, based on the high level of vector competence in our study, its known role in other countries in Asia (Mitamura et al. 1937, Huang 1982, Burke and Leake 1988, Rosen et al. 1989, Vaughn and Hoke 1992), the numerous isolations of JEV from this species captured in the ROK in 2000 (Turell et al. 2003), and its continued presence in large numbers (Burkett et al. 2002), Cx. tritaeniorhynchus should be considered to be the principal vector of JEV in the ROK. As the recent isolations of JEV indicate, JEV is still actively being transmitted in the ROK. The lack of human cases is probably due to an effective vaccination program. As illustrated by the cases of JE in foreign visitors to Hong Kong and Shanghai in 2004 (Poerschke 2004, Gingrich 2005), nonvaccinated foreign travelers to areas where JEV is being transmitted may be at risk of infection and disease, even if disease is not occurring in the local population due to a vaccination program (Borwein 2005).
Acknowledgements
We thank Edward C. Huycke, Commander, 18th Medical Command, and Patrick T. Stackpole, Commander, Camp Greaves, for support in the evaluation of mosquito fauna for arboviruses. We thank Wan Y. Kim for logistical support and as an interpreter, and Joan Eitzen, Zia Mehr, Anthony Schuster, and others from CHPPM-Pacific for support. We are grateful for the assistance of Edwin Huertas, Hee-Choon Lee, Myong Wa Yi, and others from the office of the Deputy Chief of Staff, Force Health Protection, 18th Medical Command, for logistical and excellent technical assistance. We thank Hae-Wol Cho (Korean Center for Disease Control and Prevention, National Institute of Health) for assistance. We also thank M. O'Guinn, J. Kondig, and K. Kenyon for critically reading the manuscript and providing editorial comment. We thank J. Pecor (Biosystematics Unit, Department of Entomology, Walter Reed Army Institute of Research) for identifying mosquitoes and D. Fonseca (Academy of Natural Sciences, Philadelphia, PA) for molecular confirmation of their identity. Finally, we acknowledge the trap donations from the American Biophysics Corporation and Environmental Products and Research. Funding for this project was provided by the Department of Defense, Global Emerging Infections Surveillance and Response System (DoD-GEIS), Walter Reed Army Institute of Research, and the 18th Medical Command, Eighth U.S. Army, Republic of Korea. This research was performed while C.N.M. held a National Research Council Research Associateship Award at USAMRIID.
The mention of trade names or commercial products does not constitute endorsement or recommendation for use by the Department of Defense or the Korean National Institute of Health. Opinions, interpretations, conclusions, and recommendations are those of the authors and are not necessarily endorsed by the U.S. Army.
References Cited