-
PDF
- Split View
-
Views
-
Cite
Cite
Roger W. Meola, Susan R. Dean, Govindan Bhaskaran, Effects of Juvenile Hormone on Eggs and Adults of the Cat Flea (Siphonaptera: Pulicidae), Journal of Medical Entomology, Volume 38, Issue 1, 1 January 2001, Pages 85–92, https://doi.org/10.1603/0022-2585-38.1.85
- Share Icon Share
Abstract
Juvenile hormone III plays a major role in regulating feeding and reproduction in the adult cat flea, Ctenocephalides felis (Bouché). Both blood consumption and egg production increased in a dose-dependent manner up to a maximum at 1,250 ppm when fleas were continuously exposed to concentrations up to 12,500 ppm juvenile hormone. Histological studies demonstrated that juvenile hormone III also stimulated cellular differentiation of salivary gland epithelia, midgut epithelia, and fat body cells, enhancing the ability of the adult flea to digest blood and synthesize vitellogenins for the maturing oocytes. In unfed fleas, exposure of adults to concentrations of ≥1,000 ppm juvenile hormone III applied to filter paper resulted in membrane lysis and destruction of salivary gland and midgut epithelial cells, fat body cells, and ovarian tissue. Unlike juvenile hormone mimics, which have potent ovicidal effects in fleas, juvenile hormone had little effect in preventing egg hatch; 58% of the eggs laid by fleas treated with 12,500 ppm juvenile hormone III hatched, and a concentration of 30,000 ppm was required to reduce hatch to 2% in untreated eggs exposed to treated filter paper for 2 h. Compared with the juvenile homone mimic pyriproxyfen, juvenile hormone III was less toxic to fed adult fleas. However, at a concentration of 12,500 ppm, juvenile hormone killed ≈45% of the adults and caused autolysis and yolk resorption in the developing oocytes. Thus, at high concentrations, juvenile hormone appears to have a pharmacological effect on fleas, which is highly unusual in insects.
Although insect growth regulators that mimic the action of juvenile hormone are used extensively to control the cat flea, Ctenocephalides felis (Bouché), little is known about the physiological effects of juvenile hormone in this insect. Rothschild et al. (1970) postulated that juvenile hormone is released from the corpora allata of blood-fed rabbit fleas Spilopsyllus cuniculi (Dale) in response to cortisol or estrogen secretion by the host, thereby initiating a chain of events leading to cellular differentiation of specific flea tissues. The tissues thought to be affected by juvenile hormone in S. cuniculi, the salivary glands, midgut epithelia, oocytes, fat body, and accessory glands, undergo cellular changes that directly enhance the flea’s ability to digest blood and reproduce (Rothschild et al. 1986). Silver et al. (1997) determined that juvenile hormone esterases are present in larval and adult tissues of the cat flea, which tends to confirm that juvenile hormone is indeed present in the flea and that it plays a role in cat flea reproduction. Further evidence of this effect is provided by work demonstrating that movement of sperm from the testes to the epididymis is stimulated in unfed adult male cat fleas exposed to juvenile hormone III or juvenile hormone mimics (Dean and Meola 1997).
Tissue differentiation during the course of bloodfeeding also has been observed in C. felis (Akin 1984) and Echidnophaga oshanini Wagn. (Vashchenok 1966). Histological changes observed after bloodfeeding commenced include the following: (1) transformation of cuboidal midgut epithelial cells into columnar cells that actively secrete digestive enzymes from clear vesicles in their apical ends; (2) enlargement of the salivary gland epithelial cells and their nuclei concurrent with secretion of larger droplets of salivary material; (3) expansion of fat body cells with a reduction in the number and size of lipid droplets and an accumulation of protein inclusions surrounding the nucleus; and (4) an increase in the size of resting stage oocytes and follicular epithelial cells as yolk is deposited in the oocytes and the chorion is formed. These cellular changes have not yet been definitively linked to host hormone or juvenile hormone secretion in the cat flea. In fact, exposure of adult cat fleas to pyriproxyfen, a juvenile hormone mimic, increases their mortality rate by causing degeneration of the aforementioned tissues (Meola et al. 1993, 1996).
The objectives of the current study were to determine the physiological and morphological effects of exposing cat flea eggs and adults to different concentrations of juvenile hormone III. Unfortunately, we were unable to use the classic techniques of allatectomy and juvenile hormone rescue in these experiments because the small size of the flea defeated our attempts to surgically remove the corpora allata. We were also unable to perform chemical allatectomies by exposing fleas to precocenes.
Materials and Methods
Laboratory Insects.
Fleas were obtained from a colony originally supplied by Zoecon (Dallas, TX) in 1987 and maintained in culture at Texas A&M University. Adult fleas for the colony were fed on five domestic cats housed in the Laboratory Animal Resources and Research facility on campus. Eggs collected daily were brought to our laboratory at the Center for Structural and Urban Entomology where they were placed on 30 g of larval rearing medium made from 6 g dried bovine blood, 23 g finely ground cat chow, and 200 mg powdered brewer’s yeast in 310 g of fine sand. Cocoons were sifted from the rearing medium and stored in plastic containers in an environmental chamber maintained at 25°C, 70–80% RH, and a photoperiod of 14:10 (L:D) h.
Exposure of Unfed Adults to Juvenile Hormone-Treated Paper.
Filter papers used to treat fleas in these tests were 7-cm-diameter MFS (MicroFiltrationSystems, Dublin, CA). Filter paper treatments were prepared from a 1-mg/ml isooctane solution of technical juvenile hormone III (Sigma, St. Louis, MO) by evaporating the solvent from given volumes of stock solution, redissolving them in 1 ml of acetone, and applying them to filter papers. Acetone control papers were treated with 1 ml technical grade acetone. Juvenile hormone was applied to filter papers at rates of: 1.1, 11, 55, and 110 μg/cm2, which corresponded to 100, 1,000, 5,000, and 10,000 ppm, respectively (weight of [AI]/weight of filter paper). Treated filter papers were dried in a fume hood for a minimum of 2 h to vaporize the acetone. To rule out the possibility that juvenile hormone is toxic to fleas because of its lipophilic properties, mineral oil controls (110 μg/cm2) were included with the treatments.
Fleas were collected when they were 7 d old (14 d after cocoon formation) and separated by vacuum aspiration into 35 replicate groups of ≈100 each. Each group of fleas was shaken into pint jars containing juvenile hormone III- or acetone-treated filter papers. Each treatment and control consisted of six replicate jars. Jars were covered with nylon mesh fabric and placed in an environmental chamber maintained at 25°C and 70–80% RH. The number of dead fleas was determined daily for the first five jars in each group. Twenty live fleas were taken from the sixth jar of each group on day 7 and fixed for histological study.
Exposure of Fed Adults to Juvenile Hormone-Treated Dog Hair.
Groups of 70, 7-d-old fleas (50 ♀, 20 ♂) were aspirated into each of 35 cages containing dog hair as a resting substrate. In each treatment, 5 ml of acetone or an acetone solution containing 1.25, 12.5, 125, 1,250 or 12,500 μg juvenile hormone was applied to 1 g of dog hair, resulting in treatment concentrations of 0, 1.25, 12.5, 125, 1,250, or 12,500 ppm. Mineral oil at the rate of 12,500 ppm was included as an additional control. Five replicate cages were used for each treatment. Adults were fed bovine blood using an artificial membrane system previously described by Pullen and Meola (1995). Blood used in these experiments was collected aseptically from a herd of donor cattle that were maintained without insecticide or antihelminthic treatments.
To ensure that fleas had access to fresh blood, clean reservoirs containing 7 ml of blood were placed on each cage daily. Fecal blood and eggs were removed daily from four cages in each treatment and control group over a period of 10 d. Fecal blood produced during this period was weighed, and counts were made of the number of eggs and dead fleas in each cage. On day 7, ≈20 eggs were removed from each cage and placed on moist squares of filter paper in plastic cups containing clean sand and larval diet. Cups were maintained in an environmental chamber at 25°C and 70–80% RH. After 5 d, eggs were examined with a microscope to observe percentage hatch of flea larvae. The number of fleas emerging as adults was determined after 4 wk. On day 7, ≈20 live fleas were collected from the fifth cage of each treatment and control group and fixed for paraffin sectioning.
Exposure of Eggs to Juvenile Hormone.
Eggs were exposed to juvenile hormone on filter papers according to procedures similar to those used in the adult studies. Juvenile hormone was applied to filter papers at rates of: 0 (acetone control), 1.1, 11, 55, 110, and 220 μg/cm2, which corresponds to 0, 150, 1,500, 7,500, 15,000, and 30,000 ppm, respectively. A 220-μg/cm2 mineral oil control was also included. Five replicate papers were prepared for each concentration.
Eggs were collected from untreated fleas, aged 24 h at 25°C and 70–80% RH, and divided into 35 groups of ≈20 each. One group of eggs was placed on each treated filter paper for 2 h before it was transferred with a moist paintbrush to a dampened, untreated square of filter paper. Each square was placed in a cup containing larval rearing medium and held for 5 d before determining percentage egg hatch; the number of fleas emerging as adults was determined after 4 wk.
Preparation of Fleas for Histology.
Adult fleas, which had their legs severed to facilitate entry of fixative into tissues, were submersed in a combo fixative (eight parts absolute ethanol, one part formalin, one part chloroform, 10 drops DMSO, and 0.5 g picric acid/100 ml solution) for at least 24 h before processing. Fleas were dehydrated in 70 and 95% ethanol for 1 h in each solution and three changes of 100% ethanol for 1 h each and infiltrated with Hemo-De (a xylene substitute, Fisher, Pittsburg, PA) for two changes of 30 min each, equal parts of Hemo-De and paraffin for 1 h at 60°C and one change of paraffin overnight at 60°C. Specimens were then placed in fresh paraffin at a vacuum of 10 torr for 30 min and embedded in paraffin for sectioning. Paraffin sections were cut at 7 μm and stained using the Mallory-Heidenhain procedure of Cason (1950).
Statistics.
Means were compared with the control by using a 2-sample, unpaired t-test (P > 0.05).
Results
Juvenile Hormone Treatment of Unfed Fleas.
Mortality of unfed fleas exposed to filter papers treated with juvenile hormone III at concentrations ranging from 100 to 10,000 ppm increased in a dose-dependent manner between day 5 and 10 (Fig. 1). After 5 d, mortality of fleas among all but the 100 ppm treatment groups and mineral oil control was significantly greater than that of the acetone controls (mineral oil control: t = 1.313, df = 8, P > 0.05; 100 ppm: t = 2.03, df = 8, P > 0.05; 1,000 ppm: t = 6.59, df = 8, P < 0.05; 5,000 ppm: t = 8.47, df = 8, P < 0.05; 10,000 ppm: t = 20.08, df = 8, P < 0.05).
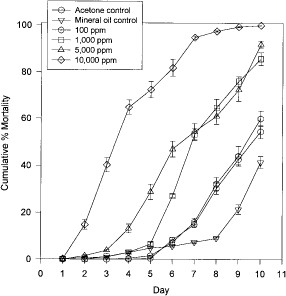
Mean cumulative percentage mortality of unfed adult fleas maintained on acetone or juvenile hormone IIItreated filter paper.
Histological sections of 7-d-old unfed fleas treated with at least 11 μg/cm2 (1,000 ppm) juvenile hormone III showed degeneration of midgut and salivary gland epithelial cells, fat body cells, and ovarian tissue in contrast to the normal cellular structure found in control fleas (Fig. 2). Higher magnification micrographs revealed that the midgut epithelial cells of control fleas had partially differentiated from the flat, cuboidal cells seen in newly emerged fleas (Fig. 3 A) to columnar cells seen at 7 d (Fig. 3B). In comparison, the midgut epithelia of treated fleas atrophied (Fig. 3C), resulting in expansion of the midgut as shown in Fig. 2B. Evidence of cellular degeneration also was observed in the salivary glands. The salivary epithelial cells of the controls (Fig. 4 A) were rounded with scalloped edges and distinct nuclei compared with salivary glands of treated fleas in which the epithelial cells were flattened and had indistinct boundaries with disrupted nuclear membranes (Fig. 4B). Comparison of fat body cells of control fleas (Fig. 5 A) that contain lipid vacuoles and well-defined nuclear and cytoplasmic membranes with fat body cells of treated fleas (Fig. 5B) showed that the nuclear and cytoplasmic membranes of treated fat body cells had undergone autolysis resulting in cellular degeneration and depletion of lipid droplets.
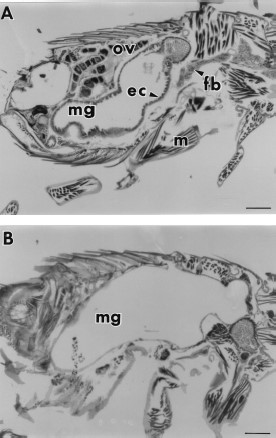
(A) An unfed 7-d-old control flea with abundant fat body (fb), midgut (mg) with epithelial cells (ec), muscles (m), and ovarian tissue (ov). (B) An unfed 7-d-old flea maintained on paper treated with 110 μg/cm2 (10,000 ppm) juvenile hormone III showing an expanded midgut with degenerated midgut epithelia, fat body and ovarian tissue. Bar = 169 μm.
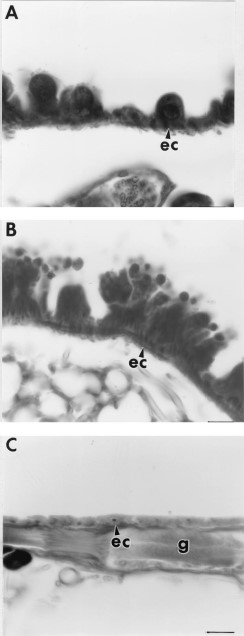
(A) Cuboidal midgut epithelial cells (ec) of a newly emerged flea. (B) Developing columnar midgut epithelial cells of a 7-d-old unfed control flea. (C) Remnants of degenerated midgut epithelial cells in a 7-d-old unfed flea that had been exposed to 110 μg/cm2 juvenile hormone III. g = ganglion. Bar = 16.9 μm.
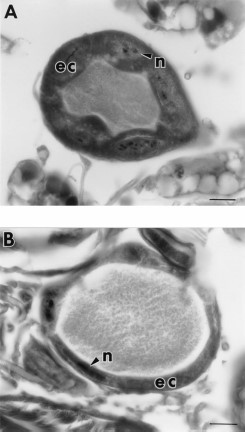
(A) Salivary gland epithelial cells (ec) of a 7-d-old unfed control flea showing scalloped membranes and large rounded nuclei (n). (B) Salivary gland epithelial cells of a 7-d-old unfed flea exposed to 110 μg/cm2 juvenile hormone III showing thin flattened epithelial cells and nuclei. Bar = 16.9 μm.
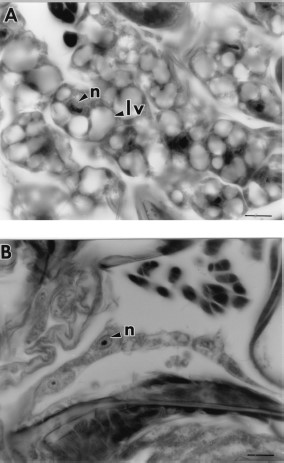
(A) Fat body cells of a 7-d-old unfed control flea with large lipid vacuoles (lv) and nucleus (n). (B) Fat body cells of a 7-d-old unfed flea exposed to 110 μg/cm2 juvenile hormone III that had degenerated and were depleted of lipid vacuoles. Bar = 16.9 μm.
Juvenile Hormone Treatment of Blood-Fed Fleas.
When blood-fed fleas were maintained on juvenile hormone III-treated dog hair in concentrations ranging from 1.25 to 12,500 ppm (Fig. 6), mortality was significantly greater in the 12,500 ppm group after 1 d than in the acetone controls (12,500 ppm: t = 19.85, df = 8, P < 0.05). In contrast, no significant mortality occurred in the mineral oil controls or in fleas treated with 1.25 or 125 ppm juvenile hormone III (mineral oil: t = 0.730, df = 8, P > 0.05; 1.25 ppm: t = 0.5, df = 8, P > 0.05; 125 ppm: t = 0.5, df = 8, P > 0.05). However, mortality was significantly lower in the 12.5 ppm (days 1–3 and 5–10) and 1,250 ppm (days 2,3 and 5) groups (12.5 ppm: t = -3.1, df = 8, P < 0.05; 1,250 ppm: t = -3.1, df = 8, P < 0.05).
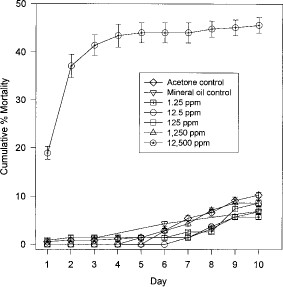
Mean cumulative percentage mortality of blood fed adult fleas maintained on juvenile hormone III-treated hair.
Examination of histological sections of 7-d-old blood-fed fleas treated with 0, 1.25, 12.5, and 125 ppm juvenile hormone III revealed that these fleas were similar to each other in appearance (not pictured). No evidence of degeneration of midgut or salivary gland epithelial cells or fat body cells was observed. Sections of 7-d-old blood-fed fleas treated with 1,250 or 12,500 ppm juvenile hormone III were also similar in appearance to untreated fleas (Fig. 7 A), except that many of the oocytes appeared to be degenerating (Fig. 7B). The nuclei of these oocytes appeared to be undergoing autolysis and the yolk granules were being digested and reabsorbed. Comparison of oocytes from control (Fig. 8 A) versus treated fleas demonstrated that the follicular epithelium also was degenerating (Fig. 8B). Fleas treated with all concentrations of juvenile hormone III appeared to have more oocytes maturing at one time in the ovarioles than did controls.
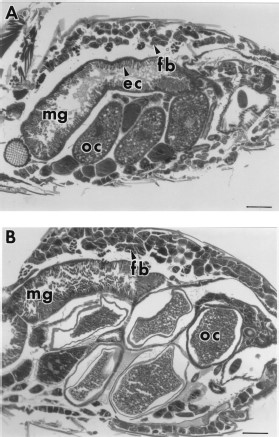
(A) A 7-d-old blood-fed control flea showing the midgut (mg) with well-developed epithelial cells (ec), developing oocytes (oc), and fat body (fb). (B) A 7-d-old blood-fed flea exposed to 12,500 ppm juvenile hormone IIItreated hair is similar in appearance to the control except that the oocytes are degenerating. Bar = 169 μm.
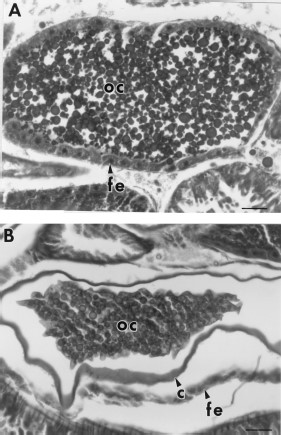
(A) Oocyte (oc) of a 7-d-old blood-fed control flea which contains abundant yolk and a well-developed follicular epithelium (fe) (nucleus not visible in this section). (B) Oocyte of a 7-d-old blood-fed flea exposed to 12,500 ppm juvenile hormone III-treated hair showing reabsorption of yolk and a degenerating follicular epithelium. c = chorion. Bar = 37.6 μm.
Fleas maintained on juvenile hormone III-treated hair in the artificial membrane system produced significantly more fecal blood and, presumably, fed more than control fleas over a period of 10 d (Table 1). When fleas were treated with concentrations of juvenile hormone III ranging from 1.25 to 1,250 ppm, fecal blood production increased in a dose-dependent manner; fleas treated with 1,250 ppm produced almost three times as much fecal blood as controls. Fecal blood production in the 12,500 ppm group, although significantly greater than that of the controls, was less than in the 125 and 1,250 ppm groups, probably because of greater mortality in the 12,500 ppm group.
Daily fecal blood production (mg/flea) ± SD by fleas maintained on juvenile hormone III-treated dog hair and fed with an artificial membrane system
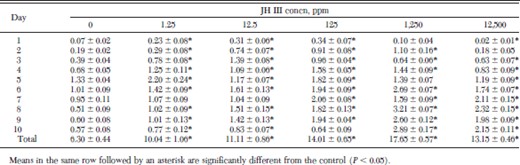
Daily fecal blood production (mg/flea) ± SD by fleas maintained on juvenile hormone III-treated dog hair and fed with an artificial membrane system
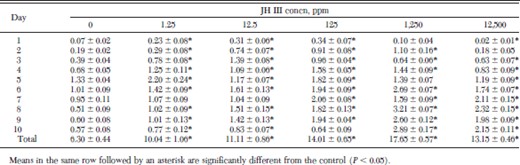
Fleas maintained on juvenile hormone III-treated hair in the artificial membrane system produced significantly more eggs than control fleas over a 10-d period (Table 2). When fleas were treated with concentrations of juvenile hormone III ranging from 1.25 to 1,250 ppm, egg production increased in a dose-dependent manner. Fleas treated with 1,250 ppm juvenile hormone III produced more than six times as many eggs as the controls over a 10-d period. Egg production in the 12,500-ppm group, although significantly greater than in the controls, was less than in the 1,250-ppm group, probably as a result of greater mortality in this group. Less than 10% of the eggs produced by fleas treated with concentrations of 0, 1.25, 12.5, or 125 ppm juvenile hormone III were devoid of yolk or collapsed. In contrast, significantly more collapsed eggs were produced by fleas treated with 1,250 ppm (15–20%) or 12,500 ppm (20–40%) juvenile hormone III (1.25 ppm: t = 0.347, df = 8, P > 0.05; 12.5 ppm: t = 0.343, df = 8, P > 0.05; 125 ppm: t = 0.39, df = 8, P > 0.05; 1,250 ppm: t = 2.96, df = 8, P < 0.05; 12,500 ppm: t = 7.72, df = 8, P < 0.05).
Mean number of eggs/♀ ± SD produced by blood-fed fleas maintained on juvenile hormone III-treated hair
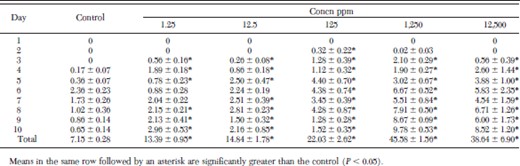
Mean number of eggs/♀ ± SD produced by blood-fed fleas maintained on juvenile hormone III-treated hair
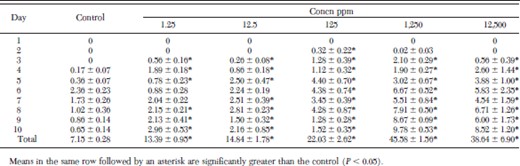
Treatment of adult fleas with juvenile hormone III had little effect on the ability of their eggs to hatch or progeny to complete development to the adult stage (Table 3). A treatment of 12,500 ppm juvenile hormone III was required to significantly reduce percentage egg hatch and development to the adult stage in progeny fleas.
Egg hatch and adult emergence from eggs laid by juvenile hormone III-treated fleas
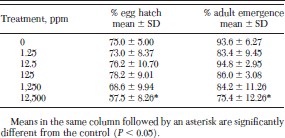
Egg hatch and adult emergence from eggs laid by juvenile hormone III-treated fleas
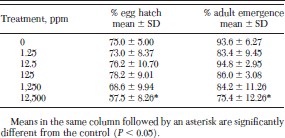
Juvenile Hormone Treatment of Eggs from Untreated Fleas.
A concentration of 1,500 ppm juvenile hormone III was required to significantly reduce percentage egg hatch and 7,500 ppm was required to significantly reduce percentage adult emergence in eggs from untreated fleas treated with juvenile hormone III (Table 4). A concentration of 30,000 ppm reduced percentage egg hatch almost to zero and completely inhibited adult emergence.
Egg hatch and adult emergence of eggs collected from untreated fleas and aged 24 h before exposure to juvenile hormone III-treated filter papers for 2 h
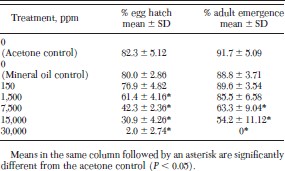
Egg hatch and adult emergence of eggs collected from untreated fleas and aged 24 h before exposure to juvenile hormone III-treated filter papers for 2 h
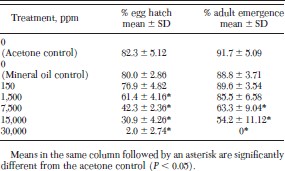
Discussion
Our results suggest that juvenile hormone III plays a major role in regulating reproduction in the adult cat flea by initiating the differentiation of tissues needed for feeding and digestion of blood and the processing of nutrients for vitellogenin synthesis. Fleas exposed to increasing concentrations of juvenile hormone III ingested increasing amounts of blood (based on amounts of fecal blood) and produced greater numbers of eggs over a 10-d period. Consequently, fleas appear to be similar to many other adult insects in which juvenile hormone causes an increase in fecundity by increasing yolk protein synthesis by the fat body and uptake of vitellogenin by the maturing oocytes (Wyatt and Davey 1996, Wyatt 1988). Because the adult cat flea is a blood-feeding insect, cellular differentiation of the salivary gland and midgut epithelia enables the flea to imbibe blood and digest it more rapidly, enhancing vitellogenin synthesis.
Because both untreated and juvenile hormone III-treated fleas underwent differentiation of the same tissues after blood feeding, it appears that juvenile hormone was produced endogenously by the adult cat flea in response to bloodfeeding or some other stimulus. Possibly, small amounts of juvenile hormone III were also produced by unfed fleas in view of the partial differentiation of midgut cells that we observed in fleas 7 d after eclosion. Although we did not observe cellular differentiation in tissues of blood-fed fleas as a series of graded steps in response to increasing concentrations of juvenile hormone III, we were able to observe an increase in the numbers of maturing oocytes in the ovarioles. Possibly, electron microscopy studies of midgut and salivary gland epithelia and fat body cells would yield qualitative and quantitative differences in mitochondria, endoplasmic reticulum, golgi, and other cellular organelles that would correlate cellular activity with juvenile hormone III concentration.
Although exposure of blood-fed fleas to juvenile hormone III concentrations ranging from 1.25 to 1,250 ppm increased feeding and fecundity, exposure of blood-fed fleas to 12,500 ppm and unfed fleas to at least 1,000 ppm resulted in increased mortality. The only histological evidence of tissue degeneration that we observed in fleas fed 12,500 ppm of juvenile hormone was autolysis and yolk resorption in the oocytes. In contrast, unfed fleas treated with at least 1,000 ppm juvenile hormone III died in response to the breakdown of cellular membranes and tissue destruction in the midgut and salivary gland epithelia, fat body cells, and ovarian tissue. The histopathological effects of juvenile hormone were identical to the effects observed in pyriproxyfen-treated fleas (Meola et al. 1993, 1996). Therefore, high concentrations of juvenile hormone appeared to cause pharmacological effects in unfed cat fleas. The concentration of juvenile hormone that is lethal to blood-fed fleas may be greater than the concentration lethal to unfed fleas because blood-fed fleas have greater levels of juvenile hormone esterases present in their midguts (Silver et al. 1997), and, thus, may be able to metabolize excess juvenile hormone more efficiently than unfed fleas. Other researchers have reported that juvenile hormone alters membrane enzyme activity and permeability (Barber et al. 1981, Wyatt and Davey 1996). This may explain why physiological concentrations of juvenile hormone III promote cellular differentiation and yolk uptake by oocytes, whereas pharmacological concentrations cause membrane destruction and lysis of cells.
Pyriproxyfen is far more toxic to fleas than juvenile hormone III, possibly because juvenile hormone esterases do not degrade pyriproxyfen as they do juvenile hormone (Campbell et al. 1998, Graf 1999). This may explain why pyriproxyfen causes high mortality of adults when unfed fleas are continuously exposed to 0.1 ppm or bloodfed fleas to 12.5 ppm pyriproxyfen (Meola et al. 1996). Pyriproxyfen, in addition to being more toxic than juvenile hormone III to adult fleas, is also more toxic to eggs. Less than 5% of the eggs laid by fleas treated with 12.5 ppm pyriproxyfen hatched (S.R.D., unpublished data) versus 58% of those laid by fleas treated with 12,500 ppm juvenile hormone III. Likewise, when eggs laid by untreated fleas were aged for 24 h and held on filter papers treated with 100 ppm pyriproxyfen for 2 h, ≈9% hatched (Meola et al. 1993) versus 31% of those treated with 15,000 ppm juvenile hormone III. Considering these differences, pyriproxyfen may be more than a juvenile hormone mimic, because it is much more potent in its insecticidal effects on insects than other compounds such as methoprene, which is a structural analog of juvenile hormone.
Acknowledgements
We acknowledge the help of Janice Readio in reviewing the manuscript. This research was funded by Texas Agricultural Experiment Station Project 6526.
In conducting the research described in this report, the investigators adhered to the “Guide for the Care and Use of Laboratory Animals,” as promulgated by the Committee on Care and Use of Laboratory Animals of the Institute of Laboratory Animal Resources, National Research Council. The facilities are fully accredited by the American Association of Laboratory Animal Care.
References Cited