-
PDF
- Split View
-
Views
-
Cite
Cite
George B. Schoeler, Stephen A. Manweiler, Douglas K. Bergman, Stephen K. Wikel, Influence of Repeated Infestations with Pathogen-Free Ixodes scapularis (Acari: Ixodidae) on In Vitro Lymphocyte Proliferation Responses of C3H/HeN Mice , Journal of Medical Entomology, Volume 37, Issue 6, 1 November 2000, Pages 885–892, https://doi.org/10.1603/0022-2585-37.6.885
- Share Icon Share
Abstract
In the United States, Ixodes scapularis Say has been implicated as the vector of at least three human pathogens. Tick induced modulation of host immunity is increasingly recognized as an important factor in successful transmission or establishment of tick-borne pathogens. This study was conducted to determine the effects of repeated infestations with pathogen-free I. scapularis nymphs on in vitro proliferative responses of splenic lymphocytes from C3H/HeN mice. Lymphocytes from repeatedly infested and uninfested mice were exposed to concanavalin A (Con A), Escherichia coli Castellini & Chalmers lipopolysaccharide (LPS), or I. scapularis salivary gland soluble proteins (SGSP), to determine if lymphocyte responses differed between tick-exposed and nonexposed mice. Female C3H/HeN mice were infested one to four times with pathogen-free I. scapularis nymphs, with a 14-d tick-free period between each exposure. After each infestation, tick biology parameters were measured and lymphocyte proliferative responses assessed. Acquired resistance to I. scapularis was not evident in mice subjected to tick feeding. Significant differences in the responses of lymphocytes exposed to I. scapularis SGSP were observed between infested and noninfested mice. In contrast, few differences between infested and noninfested mice were evident for lymphocytes exposed to Con A or LPS. Our results suggest that repeated exposure to I. scapularis nymphs does not affect Con A or LPS-induced proliferation of splenic lymphocytes, but significantly effects lymphocyte responses to tick salivary gland antigens.
Ixodes scapularis Say is the principal vector of Borrelia burgdorferi Johnson, Schmid, Hyde, Steigerwalt & Brenner in the eastern and midwestern United States (Lane et al. 1991). Lyme borreliosis, caused by infection with B. burgdorferi, is the most commonly reported arthropod-borne disease in the United States (CDC 1999). In addition to B. burgdorferi,I. scapularis is a vector of the human pathogens Babesia microti Poche (Spielman et al. 1985) and the causative agent of human granulocytic ehrlichiosis (Walker and Dumler 1996). Recently, a new tick-borne encephalitis-like virus was isolated from I. dammini (=I. scapularis [Oliver et al. 1993]) collected in the northeastern United States (Telford et al. 1997).
It is becoming increasing clear that interactions that occur at the tick-host-pathogen interface have profound implications for the transmission and establishment of tick-borne pathogens (Zeidner et al. 1997, Wikel 1999b). Disease-causing microorganisms from viruses to metazoan endoparasites are able to modulate host innate and specific acquired immune responses directed against them (Marrack and Kappler 1994, Kotwal 1996). The interactions between the feeding tick and the host immune system are additional factors that contribute to the complexity of the tick-host-pathogen relationship. Feeding ticks stimulate host immune regulatory and effector responses involving antigen-presenting cells, T lymphocytes, cytokines, homocytotrophic and circulating antibodies, complement, granulocytes, and other immunologically active molecules (Wikel 1982a, 1996, Brossard et al. 1991, Borsky et al. 1994). A result of these interactions is that many host-tick associations are characterized by the development of immunologically acquired resistance to tick infestation (Brossard et al. 1991, Wikel 1996, Brossard and Wikel 1997). Host acquired resistance to tick infestation is expressed by reduced engorgement weights, increased feeding periods, decreased ova production, inhibited molting, and tick mortality (Wikel 1996). However, acquired resistance does not develop in all tick-host relationships. BALB/c mice do not develop resistance to either I. scapularis nymphs (Schoeler et al. 1999) or Ixodes ricinus L. nymphs or larvae (Mbow et al. 1994, Christe et al. 1998). Even in the absence of acquired resistance to feeding, tick infestation does stimulate host immune responses (Borsky et al. 1994, Ganapamo et al. 1995, Schoeler et al. 1999).
Tick saliva contains many pharmacologically active compounds (Ribeiro 1995, Champagne and Valenzuela 1996, Sauer et al. 1996), some of which are involved in modulating host immune responses to tick feeding (Wikel et al. 1996, Wikel and Bergman 1997). Tick-mediated immunomodulation includes inhibiting complement components, impairing natural-killer cell function, inhibiting antibody production, modifying T-lymphocyte cytokine responses, and suppressing mitogen-induced in vitro proliferation of T lymphocytes (Wikel et al. 1996; Wikel and Bergman 1997; Schoeler et al. 1999, 2000).
T lymphocytes are critically important as regulators and effectors of host immune responses to ticks (Wikel 1996). Because ixodid ticks attach to and feed on hosts for a period that may range from days to weeks (Sonenshine 1991), it is not surprising that molecules introduced during tick feeding modulate T-cell responses of infested hosts. Infestation with Dermacentor andersoni Stiles, Rhipicephalus sanguineus Latreille, or I. ricinus modifies the in vitro proliferative responses of lymphocytes from repeatedly infested rabbits, guinea pigs, or laboratory mice (Wikel 1982b, Schorderet and Brossard 1993, Borsky et al. 1994, Dusbabek et al. 1995, Ganapamo et al. 1996, Ferreira and Silva 1999).
Dermacentor andersoni salivary gland extracts contain molecules that suppress Con A-stimulated proliferation of lymphocytes from normal BALB/c mice (Bergman et al. 1995, 1998) and whole tick extracts of I. ricinus significantly influence the in vitro proliferative responses of lymphocytes from normal or previously infested laboratory mice (Borsky et al. 1994, Dusbabek et al. 1995). Additionally, saliva from I. dammini (=I. scapularis) was shown to suppress in vitro lymphocyte responses of normal C57BL/6 and C3H/H mice (Urioste et al. 1994). However, the responses of lymphocytes from repeatedly infested mice were not determined. The objective of this study was to evaluate the effect of repeated infestations of C3H/HeN mice with pathogen-free I. scapularis on the in vitro responses of splenic lymphocytes to Con A, Escherichia coli Castellani & Chalmers LPS, and I. scapularis SGSP.
Materials and Methods
Mice
Female C3H/HeN mice (8–12 wk in age, weighing 20–25 g), were obtained from Charles River Laboratories, Wilmington, MA. Mice were housed at room temperature (≈22°C) and provided a commercial diet and water ad libitum.
Nymphal Ticks
Pathogen-free I. scapularis nymphs were obtained from a colony maintained in this laboratory. Ticks were held at 22°C and a photoperiod of 14:10 (L:D) h in 16-ml glass vials (Wheaton Glass, Millville, NJ) over a super-saturated potassium sulfate solution.
Infestation
Mice were infested by confining ticks to a capsule secured to the back of the mouse. The capsules consisted of 1.5-ml polypropylene microcentrifuge tubes, cut in half, and secured to the back of each mouse with a four to one mixture (wt:wt) of calophonium (rosin) (Sigma, St. Louis, MO) and beeswax. Capsules were applied to control animals in the same manner and the same number of times as for the tick-exposed mice. A new infestation site was used for each tick exposure.
Each group of experimental mice was infested from one to four times with 10 pathogen-free I. scapularis nymphs each. Infestation groups consisted of three tick-infested and three unexposed control mice. Each series of infestations was repeated twice. Nymphs were allowed to feed until fully engorged or for a maximum of 5 d. A 14-d tick-free period was maintained between each infestation.
Tick Biology
Tick biology parameters measured at the end of each infestation included the following: number of nymphs that successfully fed; engorgement weight; feeding duration; and viability, determined as the percentage of nymphs that successfully molted to adults. Weights of nymphs were recorded on the day that they detached, or were removed, from the host.
Preparation of Splenocytes
After each series of infestations, mouse spleens were removed under sterile conditions and splenocytes were prepared according to the methods of Ramachandra and Wikel (1992). Splenocyte suspensions were prepared and washed in sterile RPMI 1640 medium (Life Technologies, Gaithersburg, MD), supplemented with 2 mM glutamine, 100 U/ml penicillin, 100 μg/ml streptomycin, and heat-inactivated (56°C, 30 min) fetal bovine serum (Hyclone Laboratories, Logan, UT) at a final concentration of 10%. Splenocytes were suspended at a final concentration of 5.0 × 106 cells per milliliter.
Salivary Gland Soluble Proteins (SGSP)
Adult female I. scapularis were fed on sheep for 4–6 d, as described by Patrick and Hair (1976). Ticks were washed sequentially with deionized water, 70% ethanol, 3% hydrogen peroxide, and deionized water to remove blood and other surface contaminants before dissection in sterile phosphate buffered saline (PBS, 18 mM monobasic potassium phosphate, 57 mM dibasic sodium phosphate, 75 mM sodium chloride, pH 7.2). Salivary glands were removed and frozen (−20°C) in sterile PBS.
To prepare SGSP, salivary glands were combined and homogenized in an ice-cold glass homogenizer. Particulate material was removed from the homogenate by centrifugation at 18,400 × g (13,500 rpm, JA-17 rotor, Beckman, Fullerton, CA) for 45 min at 5°C. The 18,400 × g supernatant was then centrifuged at ≈105,000 × g (50,000 rpm, TL100.3 rotor, Beckman) for 1 h at 5°C. The protein content of the supernatant (SGSP) was determined by microtiter plate bicinchoninic acid assay of Smith et al. (1985), (BCA Protein Assay, Pierce, Rockford, IL), using a bovine serum albumin standard. Salivary gland soluble protein was diluted to ≈200 μg/ml in PBS, filter-sterilized (sterile 0.22 μm centrifugal filter, Millipore), and frozen at −20°C until use.
Lymphocyte Proliferation Assay
Ixodes scapularis SGSP, Con A (Calbiochem, La Jolla, CA), and Escherichia coli LPS (Sigma) were compared to determine their effects on in vitro proliferation of repeatedly infested and control (uninfested) C3H/HeN mouse splenocytes, according to the methods of Bergman et al. (1995). Briefly, I. scapularis SGSP was diluted in sterile PBS to 2.0, 1.0, or 0.5 μg in 50 μl and Con A or LPS was diluted in sterile PBS to 0.5 or 0.1 μg in 50 μl. Fifty microliters of each dilution of SGSP, Con A, LPS, or sterile PBS was placed in triplicate into a sterile 96-well flat bottom, tissue culture microtiter plate (Corning, Corning NY). An equal volume of twofold concentrated RPMI 1640 medium, containing 200 U/ml penicillin, 200 μg/ml streptomycin, and 20% heat inactivated FBS, was then added to each well. Plates were incubated 24 h to assess sterility before adding murine splenocytes.
Splenocyte suspension (100 μl containing 5.0 × 105 cells) was added to each well and incubated at 37°C in a humidified 5% carbon dioxide atmosphere. After 56 h of incubation, 1 μCi of methyl-3H-thymidine with a specific activity of 248 GBq/mmol (NEN Life Science Products, Boston, MA) was added to each well, in a volume of 20 μl of RPMI 1640 medium lacking serum. After addition of radiolabeled thymidine, cells were cultured for an additional 18 h. After incubation, cellular nucleic acids were trapped on glass fiber filters using an automated cell harvester (Brandel, Gaithersburg, MD), and isotope incorporation determined by use of a liquid scintillation spectrophotometer (Beckman, Palo Alto CA). Each sample was assayed in triplicate. Mean counts per minute (±SE) were determined for each treatment.
Data Analysis
Kolmolgorov-Smirnov tests were used to compare tick feeding duration and weight after feeding between infestation groups. Chi-square tests were used to compare the proportion of ticks that successfully molted. The mean counts per minute of unstimulated cells and cells exposed to Con A, LPS, and SGSP from mice exposed to pathogen-free ticks and control mice not exposed to ticks were measured. Stimulation indices were calculated to normalize for the inherent variation observed from mouse to mouse and to present data as a ratio between mitogen or SGSP-exposed cells and unstimulated cells for each mouse. Stimulation indices for each mouse were calculated by dividing the mean count per minute of stimulated cells (Con A, LPS, or SGSP treated) by that of the mean count per minute of the associated unstimulated cells. The mean stimulation indices for each treatment (Con A, LPS, or SGSP dilution) within each exposure group (one, two, three, and four infestations) were calculated as the mean of the stimulation indices for the control and infested mice exposed to each treatment within each exposure group. For each tick-exposure group, counts per minute and stimulation indices of tick-exposed mice were compared with those from unexposed mice using the Kruskal–Wallis test (Steel et al. 1997).
The Kruskal–Wallis test was used to compare counts per minute and stimulation indices between all four control mouse groups and all four tick-infestation groups, separately. When a Kruskal-Wallis test indicated a significant difference (P ≤ 0.05), six pair-wise comparisons (1 versus 2, 1 versus 3, 1 versus 4, 2 versus 3, 2 versus 4, 3 versus 4) were performed using a normalized rank distribution (Gibbons 1971). The significant (two-tailed) alpha (and corresponding Z-value) was calculated using the methods of Dunn to ensure an overall alpha not more than 0.05 per six comparisons (Marascuilo and Serlin 1988). The Spearman rank correlation was used to investigate how counts per minute and stimulation indices were related to feeding by ticks and repeated infestation by ticks (Gibbons 1971). In all statistical analyses, significance was assessed at the P ≤ 0.05 level.
Results
In vitro proliferative responses of lymphocytes from C3H/HeN mice repeatedly infested with pathogen-free I. scapularis nymphs were significantly different than responses of lymphocytes from uninfested mice when exposed to salivary gland soluble proteins (SGSP) derived from adult I. scapularis females. Significant differences between infested and uninfested mice were seen for virtually every infestation at all three dilutions of I. scapularis SGSP. In contrast to SGSP responsiveness, proliferation of lymphocytes from repeatedly infested and noninfested mice differed significantly only for cells from once-infested mice cultured with 0.1 μg per well of Con A, but not for cells cultured with 0.5 μg per well of Con A. No differences in proliferation were observed for lymphocytes from infested and noninfested mice exposed to either dilution of LPS.
Tick Biology Parameters
Few significant differences in tick biology parameters of I. scapularis nymphs were observed (Table 1). The mean number of I. scapularis nymphs recovered from mice ranged from 8.00 to 9.42, with an average of 8.81 ± 0.30 (mean ± SE) ticks recovered over the four infestations. Mean weights of replete I. scapularis nymphs ranged from 3.63 ± 0.10 mg (mean ± SE) for nymphs recovered after the third infestation to 2.89 ± 0.16 mg for nymphs recovered after the fourth infestation (Table 1). The mean replete weight of nymphs recovered after the fourth infestation was significantly less than that of nymphs recovered after the second and third infestations, but not significantly different than ticks recovered after the first infestation (Table 1). Mean feeding duration of I. scapularis nymphs recovered from C3H/HeN mice ranged from 4.3 d after the first infestation to 4.1 d after the third. Mean feeding duration of ticks after the first infestation was significantly higher than feeding duration of ticks fed after the second and third exposures, but not different from ticks fed after the fourth infestation (Table 1). These differences did not reveal a consistent pattern of increase or decrease in feeding duration (Table 1). No significant differences were seen in viability, measured as percent molt, of engorged nymphs recovered.
Replete nymphs recovered from mice, mean weight ± SE of recovered nymphs, mean number of days spent feeding, and percent nymphs successfully molting to adults for I. scapularis nymphs fed on C3H/HeN mice

Replete nymphs recovered from mice, mean weight ± SE of recovered nymphs, mean number of days spent feeding, and percent nymphs successfully molting to adults for I. scapularis nymphs fed on C3H/HeN mice

In vitro Lymphocyte Proliferation
No significant differences between lymphocyte responses of infested and control mice were observed for unstimulated cells (cells not exposed to Con A, LPS, or SGSP) from any of the four infestations (Table 2). Additionally, no significant differences were seen in responses of lymphocytes from noninfested and infested animals cultured with Con A (0.5 μg per well) or either dilution of LPS (0.5 or 0.1 μg per well). The only significant difference in lymphocyte responses of cells exposed to Con A or LPS was for cells from once-infested mice exposed to 0.1 μg per well of Con A (Table 2). In this case, the count per min ± SE was 17,907 ± 643 for noninfested mice as compared with 25,555 ± 787 for mice infested once with I. scapularis nymphs. This resulted in a 42.7% increase in counts per minute, a difference that is significant (Table 2).
Counts per minute (CPM) ± SE of methyl-3H-thymidine incorporation into lymphocytes from C3H/HeN mice repeatedly infested with pathogen-free I. scapularis nymphs
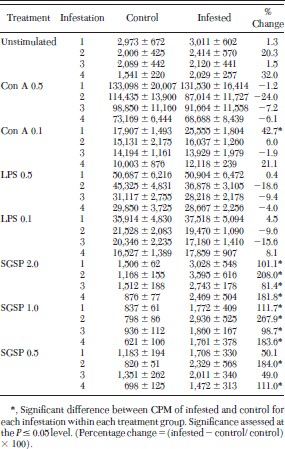
Counts per minute (CPM) ± SE of methyl-3H-thymidine incorporation into lymphocytes from C3H/HeN mice repeatedly infested with pathogen-free I. scapularis nymphs
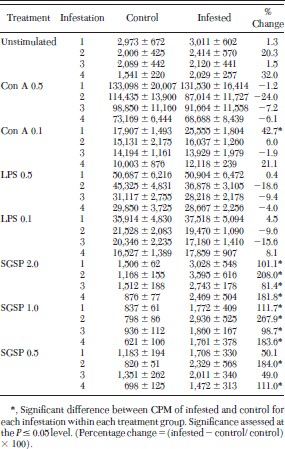
For lymphocytes cultured with each of the three dilutions of I. scapularis SGSP (2.0, 1.0, and 0.5 μg per well), significant differences in proliferative responses were seen for virtually all infestations and SGSP dilutions (Table 2). For example, lymphocytes cultured with 2.0 and 1.0 μg per well of SGSP, the counts per min ± SE of tick-infested mice were significantly higher for tick-infested mice than those of uninfested mice, for mice infested once, twice, three, and four times with pathogen-free I. scapularis nymphs (Table 2). For lymphocytes cultured with 0.5 μg per well of SGSP, counts per minute were higher for lymphocytes from infested mice, when compared with lymphocytes from uninfested mice for all infestations, the differences were only significant for mice infested twice (182.2%) and mice infested four times (108.5%, table 2). For the cells cultured with all dilutions of SGSP, the increase in proliferative responses ranged from 49% after the third infestation for cells cultured with 0.5 μg per well SGSP, to an increase of 267.9% for cells from twice-infested mice cultured with 1.0 μg per well SGSP.
The count per minute of unstimulated cells, and cells exposed to each dilution of mitogen or SGSP, differed between infestations for both control and infested mice within each infestation group. For example, the counts per min of unstimulated cells from uninfested mice ranged from 2,973 ± 672 (mean ± SE) for cells from control mice of the once-infested group to 1,541 ± 200 for cells from the four-times exposed group (Table 2). For mice exposed to tick feeding, counts per min of the 0.5 μg per well Con A-exposed cells ranged from 131,530 ± 16,414–68,688 ± 8,439 (table 2). Correlation between tick exposure (infested one, two, three, or four times) versus noninfested mice, and in vitro response of cells demonstrated that exposure to ticks did not significantly affect how cells responded to Con A or LPS (P > 0.05). In contrast, correlation between tick exposure and response to SGSP indicated that tick exposure significantly affected how cells responded to SGSP (P ≤ 0.05). However, the number of infestations was not correlated to the response of lymphocytes to SGSP (P > 0.05).
To determine how each treatment (Con A, LPS, or SGSP) affected the proliferation of lymphocytes, when compared with unstimulated cells (not exposed to mitogen or SGSP), and to normalize data by describing responses as a ratio between each treatment and the associated unstimulated cells within that treatment group, stimulation indices were calculated for cells cultured from repeatedly infested and uninfested mice. Stimulation indices for infested and noninfested mice for each dilution of Con A, LPS, or SGSP are presented in Table 3. No significant differences were seen between stimulation indices of cells from tick-infested or noninfested mice for either dilution of LPS or for the 0.1 μg per well of Con A or (Table 3). The only significant difference in stimulation indices for Con A was seen for cells cultured with 0.5 μg per well Con A after the second infestation (Table 3). In this case the difference between the tick-exposed and unexposed mice was −34.6% (Table 3). In contrast, significant differences were observed among stimulation indices of cells from tick-infested or noninfested mice for all three dilutions of SGSP (Table 3). For cells cultured with 1.0 μg per well of SGSP, the percentage difference in stimulation indices of infested mice were significantly higher than control mice for mice infested one, two, three, and four times (Table 3). For cells cultured with 2.0 and 0.5 μg per well of SGSP, stimulation indices of repeatedly infested mice were significantly higher than control mice for mice infested two, three, and four times. Stimulation indices from once-infested mice, exposed to either 2.0 or 0.5 μg per well of SGSP, were similarly higher than the stimulation indices of control mice. However, these differences were not significant (Table 3).
Stimulation indices ± SE of lymphocytes from C3H/ HeN mice repeatedly infested with pathogen-free I. scapularis nymphs
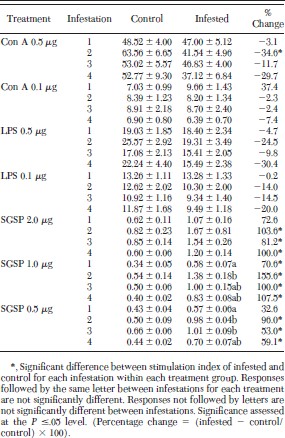
Stimulation indices ± SE of lymphocytes from C3H/ HeN mice repeatedly infested with pathogen-free I. scapularis nymphs
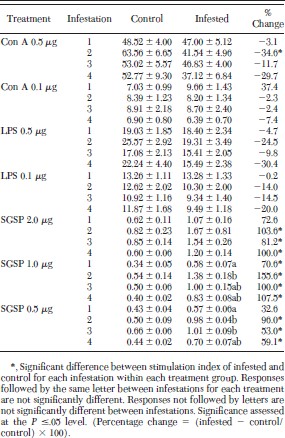
Differences in stimulation indices within each treatment (Con A, LPS, or SGSP) between infestation groups (infestations one, two, three, four) were not significant for uninfested control or infested mice cultured with Con A or LPS, or for control mice cultured with any dilution of SGSP (Table 3). In contrast, stimulation indices of cells from infested animals differed between infestation groups for cells cultured with 1.0 or 0.5 μg per well of SGSP, but not for cells cultured with 2.0 μg per well of SGSP. For example, stimulation indices of cells from infested mice, cultured with 1.0 μg per well SGSP, were significantly less for cells from once-infested when compared with twice-infested mice, but did not differ for mice infested three or four times (Table 3). Similarly, stimulation indices of cells from once-infested mice, cultured with 0.5 μg per well SGSP, were significantly less than stimulation indices from mice infested two or three times, but not different from mice infested four times with pathogen-free I. scapularis nymphs (Table 3).
For the stimulation indices calculated, correlation between tick exposure, infested (one, two, three, or four times) versus noninfested mice, and in vitro response of cells, demonstrated that exposure to ticks did not significantly affect how cells responded to Con A or LPS (P > 0.05). In contrast, correlation between tick exposure and response to SGSP indicated that tick exposure significantly affected how cells responded to SGSP (P ≤ 0.05). The number of times infested was not significantly correlated with how the cells responded to Con A, LPS, or SGSP (P > 0.05).
Discussion
No evidence of acquired resistance to I. scapularis was observed after any of the four infestations. In addition, few significant changes in lymphocyte proliferative responses were observed for Con A or LPS stimulated lymphocytes after infestation. In contrast, significant differences in lymphocyte responses to I. scapularis SGSP were observed between repeatedly infested and uninfested mice. I. scapularis SGSP suppressed the proliferation of lymphocytes from uninfested mice, while proliferation was significantly increased in mice infested with I. scapularis nymphs when compared with uninfested mice. This increase was evident after the initial infestation and continued throughout the four infestations with pathogen-free nymphs.
The host species has an impact on whether or not acquired resistance develops (Ribeiro 1989). Results of the tick biology parameters are consistent with previous studies that have reported that inbred laboratory mice do not develop acquired resistance to repeated infestations with ticks of the I. ricinus complex of species (Mbow et al. 1994,Wikel et al. 1997, Christe et al. 1998), including infestation with I. scapularis nymphs (Schoeler et al. 1999). Host-acquired resistance to tick infestation often has very profound affects on the tick, with significant reduction in engorgement weights and increased mortality of feeding ticks (Wikel 1982a, 1982b; Wikel 1996). Rabbits become progressively resistant when infested repeatedly with adult I. ricinus (Schorderet and Brossard 1993). Similarly, guinea pigs infested with I. scapularis develop resistance to successive infestations (Das et al. 1998). As a further example of the importance of the host species in the development of acquired resistance, dogs do not develop resistance to repeated infestations with R. sanguineus, whereas guinea pigs develop resistance with repeated exposures to the same species (Ferreira and Silva 1999).
The few significant differences in tick biology parameters seen in this study are not consistent with the changes associated with host acquired resistance to tick infestation (DenHollander and Allen 1985, Schorderet and Brossard 1993, Das et al. 1998). Tick feeding can be influenced by the age of ticks, environmental factors, season, and sex ratios of infesting nymphs (Gladney and Drummond 1970, Sweatman 1970, Balashov 1972, Branagan 1974, Christe et al. 1998), or by mouse strain infested (Randolph 1979). Regardless, differences in tick biology parameters observed in this study did not indicate the development of acquired resistance to infestation.
Although acquired resistance to I. scapularis was not evident in repeatedly infested mice, differences were seen in proliferative responses of splenic lymphocytes from mice exposed to feeding ticks, when compared with uninfested control mice. Specifically, lymphocytes from repeatedly infested mice, exposed to each of three dilutions of I. scapularis SGSP, showed significantly increased proliferative responses. These differences were significant after each infestation for the 2.0 μg per well and 1.0 μg per well dilution of SGSP, and after the second and fourth infestations for the 0.5 μg per well dilution of SGSP. Although the differences after the first and third infestations were not significant for this dilution, the trend was similar to that of cells exposed to the other dilutions of SGSP.
In contrast, the proliferative responses of cells from infested and noninfested mice, cultured with Con A or LPS, were significantly different only after the first infestation for cells cultured with 0.1 μg per well Con A. No other significant differences were observed in cells exposed to Con A or LPS after any of the infestations, suggesting that prior exposure to feeding I. scapularis nymphs does not affect proliferative responses of splenic lymphocytes exposed to these mitogens.
Correlation analysis revealed that tick exposure did not affect how cells responded to either Con A or LPS (P > 0.10). Correlation analyses between tick exposure and proliferative responses demonstrated that exposure to ticks significantly affected how cells responded to SGSP (P < 0.0001), whereas the number of tick exposures did not affect the magnitude of the response to SGSP (P > 0.10). Changes in in vitro proliferative response to SGSP were independent of how many times mice were infested. Furthermore, the results of the comparisons of stimulation indices of uninfested and repeatedly infested mice are consistent with comparisons based on counts per minute for cells cultured with Con A, LPS or SGSP.
The results of this study demonstrate that proliferative responses of lymphocytes from C3H/HeN mice, exposed to the mitogens Con A or LPS, are not affected by infestation with I. scapularis. However, exposure to I. scapularis feeding significantly affects the proliferative response of lymphocytes exposed to I. scapularis SGSP. One infestation is sufficient to significantly increase the proliferative responses of cells cultured with SGSP, and the magnitude of the SGSP induced response observed after the first exposure does not differ significantly from the magnitude of responses observed after the second, third, and fourth infestation.
Differences were observed in the counts per minute between uninfested mice for each of the four infestations, for each of the mitogens or SGSP. In a similar manner, differences were observed between infested mice for each mitogen and SGSP for each of the four infestations (Table 2). These differences were not unexpected, because mice from successive infestations were of different ages when lymphocytes were prepared and cultured, and it is well established that changes in both functional and phenotypic profiles of T lymphocytes occur in rodents of different ages (Linton et al. 1997).
In contrast to our results, infestation with other tick species has been shown to significantly affect mitogen-stimulated in vitro lymphocyte proliferative responses. Concanavilin A-stimulated proliferative responses of lymphocytes from guinea pigs, infested with D. andersoni, were reduced when compared with noninfested animals, and the reduction was greater during initial infestations than during subsequent infestations (Wikel 1982b). Lymph node cells of C3H/HeJ mice infested one and four times with two male and two female R. sanguineus showed a decrease of 77.9% and 82.4%, respectively, in proliferative responses to Con A when compared with cells from uninfested animals (Ferreira and Silva 1999). In contrast to lymph node cells, splenocytes from the same mice showed no significant change in proliferative responses when compared with uninfested control animals (Ferreira and Silva 1999). The Con A-stimulated proliferative response of spleen lymphocytes from BALB/c mice repeatedly infested with I. ricinus was unchanged after the primary and secondary infestations, and then increased above those from noninfested mice after the third and fourth infestations (Dusbabek et al. 1995). In contrast, B-cell responses to LPS and pokeweed mitogen were suppressed after each of four infestations (Dusbabek et al. 1995). In a similar study, Borsky et al. (1994) reported reduced responsiveness of splenocytes to Con A after a primary infestation with I. ricinus nymphs. Responsiveness increased to levels above control value after the second infestation, and then decreased again after the third exposure (Borsky et al. 1994). Finally, T cells collected from lymph nodes of I. ricinus infested BALB/c mice at 9 d postinfestation had a suppressed in vitro response to Con A. Our results do not show a change in splenic lymphocyte responsiveness to Con A or LPS between infested and uninfested mice after repeated exposures to I. scapularis nymphs.
Concanavalin A stimulates T-lymphocyte proliferation in vitro (Sharon 1983). Saliva or salivary gland extracts from various tick species contain molecules that influence Con A-stimulated lymphocyte proliferation (Urioste et al. 1994; Bergman et al. 1995, 1998; Ferreira and Silva 1999). Splenocytes from C57BL/6 and C3H/H mice, incubated in the presence of dilutions of I. dammini (=I. scapularis) saliva, had significantly decreased proliferative responses to Con A (Urioste et al. 1994). Proliferative responses were suppressed up to 70–80%, when compared with that of cells not exposed to tick saliva (Urioste et al. 1994). Salivary gland extracts prepared daily from feeding D. andersoni females suppressed in vitro lymphocyte responsiveness to Con A and enhanced proliferation in the presence of LPS for lymphocytes from uninfested mice (Ramachandra and Wikel 1992) and cattle (Ramachandra and Wikel 1995). Subsequent studies identified a 36-kDa soluble protein from D. andersoni salivary glands to be responsible for suppression of Con A-induced lymphocyte proliferation (Bergman et al. 1998).
Our results, in agreement with those of other studies, demonstrate that lymphocytes from tick-exposed animals respond differently to tick-derived antigens, when compared with lymphocytes from uninfested control animals. For example, D. andersoni salivary gland extract enhanced in vitro lymphocyte proliferation of lymphocytes from tick-resistant guinea pigs when compared with noninfested animals (Wikel et al. 1978). Lymphocytes from rabbits or BALB/c mice, cultured in the presence of salivary gland or integumental extracts, had significantly enhanced responsiveness (Schorderet and Brossard 1993, Borsky et al. 1994). Similar results were reported by Dusbabek et al. (1995) for I. ricinus infested BALB/c mice (Dusbabek et al. 1995). Results of these and the current study demonstrate that prior exposure to tick salivary or whole tick extract antigens significantly stimulates the antigen-specific in vitro proliferative responses of lymphocytes.
T lymphocytes have both immunoregulatory and effector functions that are essential for maintaining the integrity of the immune system; therefore, it is not surprising that ticks have evolved substances to modulate T-lymphocyte responses (Wikel 1999a, 1999b). Modifying T-lymphocyte responses could affect host immune responses not only to the feeding tick, but to tick-transmitted infectious agents as well. Studies are currently underway to identify those salivary gland molecules that are responsible for modulating T-lymphocyte proliferative responses to I. scapularis.
Acknowledgements
The authors thank R. W. Barker and J. W. Dillwith (Oklahoma State University) for critically reviewing the manuscript. This study was supported in part by a grant from the Centers for Disease Control and Prevention, U.S. Public Health Service (S.K.W.), and Oklahoma Agricultural Experiment Station Project OKL02174 (S.K.W.). Approved for publication by the director, Oklahoma Agricultural Experiment Station.
References Cited
Author notes
This study was conducted according to the guidelines of the Institutional Animal Care and Use Committee, Oklahoma State University.