-
PDF
- Split View
-
Views
-
Cite
Cite
Ling-Yan Jiang, Yuan-Yuan Zhang, Zhen Li, Jian-Zhong Liu, Metabolic engineering of Corynebacterium glutamicum for increasing the production of l-ornithine by increasing NADPH availability, Journal of Industrial Microbiology and Biotechnology, Volume 40, Issue 10, 1 October 2013, Pages 1143–1151, https://doi.org/10.1007/s10295-013-1306-2
- Share Icon Share
Abstract
The experiments presented here were based on the conclusions of our previous proteomic analysis. Increasing the availability of glutamate by overexpression of the genes encoding enzymes in the l-ornithine biosynthesis pathway upstream of glutamate and disruption of speE, which encodes spermidine synthase, improved l-ornithine production by Corynebacterium glutamicum. Production of l-ornithine requires 2 moles of NADPH per mole of l-ornithine. Thus, the effect of NADPH availability on l-ornithine production was also investigated. Expression of Clostridium acetobutylicum gapC, which encodes NADP-dependent glyceraldehyde-3-phosphate dehydrogenase, and Bacillus subtilis rocG, which encodes NAD-dependent glutamate dehydrogenase, led to an increase of l-ornithine concentration caused by greater availability of NADPH. Quantitative real-time PCR analysis demonstrates that the increased levels of NADPH resulted from the expression of the gapC or rocG gene rather than that of genes (gnd, icd, and ppnK) involved in NADPH biosynthesis. The resulting strain, C. glutamicum ΔAPRE::rocG, produced 14.84 g l−1 of l-ornithine. This strategy of overexpression of gapC and rocG will be useful for improving production of target compounds using NADPH as reducing equivalent within their synthetic pathways.
Introduction
l-Ornithine, a non-essential amino acid and an important constituent of the urea cycle, is the precursor of other amino acids, such as citrulline and arginine. It is effective for the treatment and prophylaxis of liver diseases [24] and has been applied to promote wound healing [28]. Recently, it was demonstrated that l-ornithine supplementation increased serum levels of growth hormone and insulin-like growth factor-1 after strength-trained athletes engaged in heavy-resistance exercise [33]. Many studies have reported that high yields of l-ornithine can be produced from a citrulline- or arginine-requiring mutant of a coryneform bacterial strain generated by classical mutagenesis [3, 12, 15, 34]. Although this mutant produces a high yield of l-ornithine, cultures are unstable owing to reversion of the auxotrophic phenotype, which causes significant inhibition of the production of l-ornithine.
Several recent reports have described progress in the metabolic engineering of microorganisms used for l-ornithine production. Lee and Cho reported that an engineered Escherichia coli strain, W3110 (ΔargFΔargIΔargRΔproBΔspeF, P araB-arg214), produced 13.2 mg per gram of dry cell weight (DCW) of l-ornithine and that addition of glutamate to the culture favored l-ornithine production [18]. Hwang et al. reported that overexpression of argCJBD by C. glutamicum strain ATCC 13032 (ΔargFΔargRΔproB) resulted in the production of 16.49 mg g−1 DCW of l-ornithine. The concentration of l-ornithine in the culture medium was 179.14 mg l−1 [8]. Proline can be converted into l-ornithine by ornithine cyclodeaminase, which is a key enzyme responsible for enhancing l-ornithine production by C. glutamicum in proline-supplemented media [16]. Hwang and Cho reported that overexpression of the Ncgl1469 open reading frame, encoding N-acetylglutamate synthase activity, increased l-ornithine production in C. glutamicum by 39 % [6]. Recently, the same investigators deleted gntK, which encodes gluconate kinase, of C. glutamicum ATCC 13032 (ΔargFΔargR) to obtain C. glutamicum SJC8399, which produced 13.16 g l−1 of l-ornithine [7]. The l-ornithine-producing strain C. glutamicum ATCC 13032 (ΔargFΔargR) named ORN1 was constructed and shown to produce l-ornithine from arabinose when araBAD from E. coli was expressed [26]. Recently, this group also constructed the strain C. glutamicum ORN1 (pEKEx3-xylA Xc-xylB Cg) to effectively produce l-ornithine from xylose [23].
In a previous study [21], we reported the construction of a strain of C. glutamicum ATCC 13032 (ΔargFΔproBΔkgd) that produced up to 4.78 g l−1 of l-ornithine. Comparative proteomic analysis revealed the mechanism of l-ornithine overproduction by the engineered strain. The expression levels of 202 proteins varied significantly in C. glutamicum ATCC 13032 (ΔargFΔproBΔkgd) compared with those in the wild-type strain. Of these proteins, 52 proteins were identified. l-Ornithine overproduction in the engineered strain was related to the upregulation of the expression levels of enzymes involved in the l-ornithine biosynthesis pathway and downregulation of the expression levels of proteins involved in the pentose phosphate pathway. The expression levels of enzymes in ornithine biosynthesis (ArgCJBD) downstream of glutamate are much higher than that in the upstream pathway of glutamate. The results suggested possible strategies for further enhancing l-ornithine production. The present study is based on this proteomic analysis and describes our efforts to genetically engineer C. glutamicum to produce even higher levels of l-ornithine. Production of l-ornithine required 2 moles of NADPH per mole of l-ornithine. Thus, the effect of NADPH availability on l-ornithine production was also investigated.
Materials and methods
Microorganism and media
The bacterial strains used in this study are listed in Table 1. E. coli DH5α was used for plasmid construction. C. glutamicum ΔAP was used as the parental strain for generating mutants. Luria–Bertani (LB) was used to propagate E. coli and C. glutamicum for generating recombinant DNA. For l-ornithine production by C. glutamicum, the seed medium consisted of (per liter) 25 g glucose, 10 g yeast extract, 10 g corn steep liquor, 15 g (NH4)2SO4, 2.5 g MgSO4·7H2O, 1 g of KH2PO4, 0.5 g K2HPO4, 0.5 g Na2HPO4, and 10 g CaCO3. The fermentation medium [20] consisted of (per liter) 80.0 g glucose, 14.0 g yeast extract, 37.9 g (NH4)2SO4, 1.6 g MgSO4·7H2O, 1.0 g KH2PO4, 0.5 g K2HPO4, 0.5 g Na2HPO4, 20 mg FeSO4·7H2O, 20 mg MnSO4·4H2O, 2.0 g molasses, 5.0 g KAc, 5.0 g succinic acid, 10 g CaCO3, and 1 ml Tween 80 (added after 8 h of fermentation). The initial pH of the above media was adjusted to 7.0.
C. glutamicum strains and plasmids used in this study
Strain or plasmid . | Descriptiona . | Source . |
---|---|---|
C. glutamicum strains | ||
ATCC 13032 | Wild-type | ATCC |
ΔAP | ATCC 13032, ΔargF,ΔproB | 21 |
ΔAPE | ATCC 13032, ΔargF,ΔproB,ΔspeE | This study |
ΔAPER | ATCC 13032, ΔargF,ΔproB,ΔspeE,ΔargR | This study |
ΔAPRE::rocG | ATCC 13032, ΔargF,ΔproB,ΔargR, ΔspeE::Ptac-M-rocG | This study |
ΔAPER::gapC | ATCC 13032, ΔargF,ΔproB,ΔspeE, ΔargR::Ptac-M-gapC | |
ΔAPE::rocG-R::gapC | ATCC 13032, ΔargF,ΔproB,ΔspeE::Ptac-M-rocG, ΔargR::Ptac-M-gapC | |
Clostridium acetobutylicum | Wild type, CICC8011 | CICC |
Bacillus subtilis | Wild type, CICC10033 | CICC |
Escherichia coli DH5α | supE44, hsdR17, recA1, thi-1, endA1, lacZ, gyrA96, relA1 | Invitrogen |
Plasmid | ||
PMD18-T vector | TA cloning vector, Ampr | TaKaRa |
pK18mobsacB | sacB, lacZa, Kanr, mcs mobilizable vector, allows for selection of double crossover C. glutamicum | 25 |
pK-JL | K18mobsacB derivative, sacB under the control of the tac-M promoter, Kanr | This study |
pK18mobsacB-ΔspeE | pK18mobsacB with 2162 bp BamHI-SalI fragment containing internal deletion of 199 bp fragment of speE | This study |
pK18mobsacB-ΔargR | pK18mobsacB with 1574 bp BamHI-SalI fragment containing internal deletion of 506 bp fragment of argR | This study |
pK-ΔspeE::rocG | pK-JL with flanking fragment of speE internally inserted Ptac-M-rocG | This study |
pK-ΔargR::gapC | pK-JL with flanking fragment of argR internally inserted Ptac-M-gapC | This study |
pEC-XK99E | C. glutamicum/E. coli shuttle expression vector, Kanr | 13 |
pEC-pgi | pEC-XK99E derivative containing C. glutamicum pgi, Kanr | This study |
pEC-pfkA | pEC-XK99E derivative containing C. glutamicum pfkA, Kanr | This study |
pEC-gap | pEC-XK99E derivative containing C. glutamicum gap, Kanr | This study |
pEC-pyk | pEC-XK99E derivative containing C. glutamicum pyk, Kanr | This study |
pEC-pyc | pEC-XK99E derivative containing C. glutamicum pyc, Kanr | This study |
pEC-gltA | pEC-XK99E derivative containing C. glutamicum gltA, Kanr | This study |
pEC-gdh | pEC-XK99E derivative containing C. glutamicum gdh Kanr | This study |
pEC-gapC | pEC-XK99E derivative containing C. acetobutylicum gapC, Kanr | This study |
pEC-rocG | pEC-XK99E derivative containing B. subtilis rocG, Kanr | This study |
Strain or plasmid . | Descriptiona . | Source . |
---|---|---|
C. glutamicum strains | ||
ATCC 13032 | Wild-type | ATCC |
ΔAP | ATCC 13032, ΔargF,ΔproB | 21 |
ΔAPE | ATCC 13032, ΔargF,ΔproB,ΔspeE | This study |
ΔAPER | ATCC 13032, ΔargF,ΔproB,ΔspeE,ΔargR | This study |
ΔAPRE::rocG | ATCC 13032, ΔargF,ΔproB,ΔargR, ΔspeE::Ptac-M-rocG | This study |
ΔAPER::gapC | ATCC 13032, ΔargF,ΔproB,ΔspeE, ΔargR::Ptac-M-gapC | |
ΔAPE::rocG-R::gapC | ATCC 13032, ΔargF,ΔproB,ΔspeE::Ptac-M-rocG, ΔargR::Ptac-M-gapC | |
Clostridium acetobutylicum | Wild type, CICC8011 | CICC |
Bacillus subtilis | Wild type, CICC10033 | CICC |
Escherichia coli DH5α | supE44, hsdR17, recA1, thi-1, endA1, lacZ, gyrA96, relA1 | Invitrogen |
Plasmid | ||
PMD18-T vector | TA cloning vector, Ampr | TaKaRa |
pK18mobsacB | sacB, lacZa, Kanr, mcs mobilizable vector, allows for selection of double crossover C. glutamicum | 25 |
pK-JL | K18mobsacB derivative, sacB under the control of the tac-M promoter, Kanr | This study |
pK18mobsacB-ΔspeE | pK18mobsacB with 2162 bp BamHI-SalI fragment containing internal deletion of 199 bp fragment of speE | This study |
pK18mobsacB-ΔargR | pK18mobsacB with 1574 bp BamHI-SalI fragment containing internal deletion of 506 bp fragment of argR | This study |
pK-ΔspeE::rocG | pK-JL with flanking fragment of speE internally inserted Ptac-M-rocG | This study |
pK-ΔargR::gapC | pK-JL with flanking fragment of argR internally inserted Ptac-M-gapC | This study |
pEC-XK99E | C. glutamicum/E. coli shuttle expression vector, Kanr | 13 |
pEC-pgi | pEC-XK99E derivative containing C. glutamicum pgi, Kanr | This study |
pEC-pfkA | pEC-XK99E derivative containing C. glutamicum pfkA, Kanr | This study |
pEC-gap | pEC-XK99E derivative containing C. glutamicum gap, Kanr | This study |
pEC-pyk | pEC-XK99E derivative containing C. glutamicum pyk, Kanr | This study |
pEC-pyc | pEC-XK99E derivative containing C. glutamicum pyc, Kanr | This study |
pEC-gltA | pEC-XK99E derivative containing C. glutamicum gltA, Kanr | This study |
pEC-gdh | pEC-XK99E derivative containing C. glutamicum gdh Kanr | This study |
pEC-gapC | pEC-XK99E derivative containing C. acetobutylicum gapC, Kanr | This study |
pEC-rocG | pEC-XK99E derivative containing B. subtilis rocG, Kanr | This study |
a argF, ornithine carbamoyltransferase gene; proB, γ-glutamyl kinase gene; speE, spermidine synthase gene; argR, arginine repressor gene; rocG, NADH-dependent glutamate dehydrogenase gene; pgi, glucose-6-phosphate isomerase gene; pfkA, 6-phosphofructokinase gene; gap, NAD-dependent glyceraldehyde-3-phosphate dehydrogenase gene; gapC, NADP-dependent glyceraldehyde-3-phosphate dehydrogenase gene; pyk, pyruvate kinase gene; pyc, pyruvate carboxylase gene; gltA, citrate synthase gene; gdh, NADPH-dependent glutamate dehydrogenase; sacB, levansucrase gene; Ampr, ampicillin resistance; Kanr, kanamycin resistance
C. glutamicum strains and plasmids used in this study
Strain or plasmid . | Descriptiona . | Source . |
---|---|---|
C. glutamicum strains | ||
ATCC 13032 | Wild-type | ATCC |
ΔAP | ATCC 13032, ΔargF,ΔproB | 21 |
ΔAPE | ATCC 13032, ΔargF,ΔproB,ΔspeE | This study |
ΔAPER | ATCC 13032, ΔargF,ΔproB,ΔspeE,ΔargR | This study |
ΔAPRE::rocG | ATCC 13032, ΔargF,ΔproB,ΔargR, ΔspeE::Ptac-M-rocG | This study |
ΔAPER::gapC | ATCC 13032, ΔargF,ΔproB,ΔspeE, ΔargR::Ptac-M-gapC | |
ΔAPE::rocG-R::gapC | ATCC 13032, ΔargF,ΔproB,ΔspeE::Ptac-M-rocG, ΔargR::Ptac-M-gapC | |
Clostridium acetobutylicum | Wild type, CICC8011 | CICC |
Bacillus subtilis | Wild type, CICC10033 | CICC |
Escherichia coli DH5α | supE44, hsdR17, recA1, thi-1, endA1, lacZ, gyrA96, relA1 | Invitrogen |
Plasmid | ||
PMD18-T vector | TA cloning vector, Ampr | TaKaRa |
pK18mobsacB | sacB, lacZa, Kanr, mcs mobilizable vector, allows for selection of double crossover C. glutamicum | 25 |
pK-JL | K18mobsacB derivative, sacB under the control of the tac-M promoter, Kanr | This study |
pK18mobsacB-ΔspeE | pK18mobsacB with 2162 bp BamHI-SalI fragment containing internal deletion of 199 bp fragment of speE | This study |
pK18mobsacB-ΔargR | pK18mobsacB with 1574 bp BamHI-SalI fragment containing internal deletion of 506 bp fragment of argR | This study |
pK-ΔspeE::rocG | pK-JL with flanking fragment of speE internally inserted Ptac-M-rocG | This study |
pK-ΔargR::gapC | pK-JL with flanking fragment of argR internally inserted Ptac-M-gapC | This study |
pEC-XK99E | C. glutamicum/E. coli shuttle expression vector, Kanr | 13 |
pEC-pgi | pEC-XK99E derivative containing C. glutamicum pgi, Kanr | This study |
pEC-pfkA | pEC-XK99E derivative containing C. glutamicum pfkA, Kanr | This study |
pEC-gap | pEC-XK99E derivative containing C. glutamicum gap, Kanr | This study |
pEC-pyk | pEC-XK99E derivative containing C. glutamicum pyk, Kanr | This study |
pEC-pyc | pEC-XK99E derivative containing C. glutamicum pyc, Kanr | This study |
pEC-gltA | pEC-XK99E derivative containing C. glutamicum gltA, Kanr | This study |
pEC-gdh | pEC-XK99E derivative containing C. glutamicum gdh Kanr | This study |
pEC-gapC | pEC-XK99E derivative containing C. acetobutylicum gapC, Kanr | This study |
pEC-rocG | pEC-XK99E derivative containing B. subtilis rocG, Kanr | This study |
Strain or plasmid . | Descriptiona . | Source . |
---|---|---|
C. glutamicum strains | ||
ATCC 13032 | Wild-type | ATCC |
ΔAP | ATCC 13032, ΔargF,ΔproB | 21 |
ΔAPE | ATCC 13032, ΔargF,ΔproB,ΔspeE | This study |
ΔAPER | ATCC 13032, ΔargF,ΔproB,ΔspeE,ΔargR | This study |
ΔAPRE::rocG | ATCC 13032, ΔargF,ΔproB,ΔargR, ΔspeE::Ptac-M-rocG | This study |
ΔAPER::gapC | ATCC 13032, ΔargF,ΔproB,ΔspeE, ΔargR::Ptac-M-gapC | |
ΔAPE::rocG-R::gapC | ATCC 13032, ΔargF,ΔproB,ΔspeE::Ptac-M-rocG, ΔargR::Ptac-M-gapC | |
Clostridium acetobutylicum | Wild type, CICC8011 | CICC |
Bacillus subtilis | Wild type, CICC10033 | CICC |
Escherichia coli DH5α | supE44, hsdR17, recA1, thi-1, endA1, lacZ, gyrA96, relA1 | Invitrogen |
Plasmid | ||
PMD18-T vector | TA cloning vector, Ampr | TaKaRa |
pK18mobsacB | sacB, lacZa, Kanr, mcs mobilizable vector, allows for selection of double crossover C. glutamicum | 25 |
pK-JL | K18mobsacB derivative, sacB under the control of the tac-M promoter, Kanr | This study |
pK18mobsacB-ΔspeE | pK18mobsacB with 2162 bp BamHI-SalI fragment containing internal deletion of 199 bp fragment of speE | This study |
pK18mobsacB-ΔargR | pK18mobsacB with 1574 bp BamHI-SalI fragment containing internal deletion of 506 bp fragment of argR | This study |
pK-ΔspeE::rocG | pK-JL with flanking fragment of speE internally inserted Ptac-M-rocG | This study |
pK-ΔargR::gapC | pK-JL with flanking fragment of argR internally inserted Ptac-M-gapC | This study |
pEC-XK99E | C. glutamicum/E. coli shuttle expression vector, Kanr | 13 |
pEC-pgi | pEC-XK99E derivative containing C. glutamicum pgi, Kanr | This study |
pEC-pfkA | pEC-XK99E derivative containing C. glutamicum pfkA, Kanr | This study |
pEC-gap | pEC-XK99E derivative containing C. glutamicum gap, Kanr | This study |
pEC-pyk | pEC-XK99E derivative containing C. glutamicum pyk, Kanr | This study |
pEC-pyc | pEC-XK99E derivative containing C. glutamicum pyc, Kanr | This study |
pEC-gltA | pEC-XK99E derivative containing C. glutamicum gltA, Kanr | This study |
pEC-gdh | pEC-XK99E derivative containing C. glutamicum gdh Kanr | This study |
pEC-gapC | pEC-XK99E derivative containing C. acetobutylicum gapC, Kanr | This study |
pEC-rocG | pEC-XK99E derivative containing B. subtilis rocG, Kanr | This study |
a argF, ornithine carbamoyltransferase gene; proB, γ-glutamyl kinase gene; speE, spermidine synthase gene; argR, arginine repressor gene; rocG, NADH-dependent glutamate dehydrogenase gene; pgi, glucose-6-phosphate isomerase gene; pfkA, 6-phosphofructokinase gene; gap, NAD-dependent glyceraldehyde-3-phosphate dehydrogenase gene; gapC, NADP-dependent glyceraldehyde-3-phosphate dehydrogenase gene; pyk, pyruvate kinase gene; pyc, pyruvate carboxylase gene; gltA, citrate synthase gene; gdh, NADPH-dependent glutamate dehydrogenase; sacB, levansucrase gene; Ampr, ampicillin resistance; Kanr, kanamycin resistance
Culture conditions
For l-ornithine fermentations, a 1.0-ml sample of the seed culture grown with shaking at 150 rpm at 30 °C for 12 h, was inoculated into 10 ml of the fermentation medium in a 100-ml flask, incubated at 30 °C and shaken at 150 rpm for 72 h. Kanamycin (50 μg ml−1 for E. coli and 25 μg ml−1 for C. glutamicum), chloramphenicol (20 μg ml−1 for E. coli and 10 μg ml−1 for C. glutamicum), or ampicillin (50 μg ml−1 for E. coli) were added to the medium as required.
Primers, plasmid construction, and gene knockouts
Plasmids constructed for this study are listed in Table 1. The chromosomal DNA of C. glutamicum was isolated as described by Eikmanns et al. [4]. The preparation of competent cells and electroporation of C. glutamicum was performed as described by Van der Rest et al. [31]. The mutant genotypes of C. glutamicum were confirmed using colony PCR.
Amplification of pgi, pfkA, gapA, pyk, pyc, gltA, and gdh from the genomic DNA of C. glutamicum ATCC 13032 employed the primers listed in Table 2. The products were ligated to pEC-XK99E DNA [13]. Clostridium acetobutylicum gapC was amplified from pB3gapC [5] using the primers gapCF and gapCR (Table 2) and ligated to pEC-XK99E to obtain pEC-gapC. B. subtilis rocG was amplified from genomic DNA using the primers rocGF and rocGR (Table 2) and ligated to pEC-XK99E to obtain pEC-rocG.
Primers used in this study
Primer . | Sequencea and purpose (5′–3′) . |
---|---|
sacBF | CGGCGACTAGTTGAGCTGTTGACAATTAATCATCGTGTGGTACCATGTGTGGAATTGTGAGCGGATAACAATTCCGCGGGTTCTTTAGGCCCGTAGTCT (SpeI, SacII), PCR for sacB |
sacBR | GCCGCGATATCTCTCGTGATGGCAGGTT (EcoRV), PCR for sacB |
pk18msF | GCGCCGATATCGTTCGTCTGGAAGGCAGTA (EcoRV), PCR for the backbone of pK18mobsacB except for sacB |
pk18msR | GCGCGACTAGTGCATGGGCATAAAGTTGC (SpeI), PCR for the backbone of pK18mobsacB except for sacB |
speEF5 | CGATGGATCCCGACCGCTACAAGGCATAA (BamHI), deletion of speE |
speER5 | GCGTCTAGAGCGGAAATAATGGCGAAA (XbaI), deletion of speE |
speEF3 | CGCTCTAGACGATTCTGCCTCTGGATTA (XbaI), deletion of speE |
speER3 | CGATGTCGACCACCATCTGCCCAACG (SalI), deletion of speE |
argRF5 | CGCTGGATCCTTTAAGCACGGCGTTATTT (BamHI), deletion of argR |
argRR5 | CGGTCTAGATGCGAGTCACGGGATTTA (XbaI), deletion of argR |
argRF3 | CGGTCTAGAGGTAAGGTATAACCCGAGTGT (XbaI), deletion of argR |
argRR3 | CGATGTCGACGACTTGATGCCCACGAGA (SalI), deletion of argR |
pgiF | GTAGGATCCAGGAGTTTTCATGGCGGAC (BamHI), PCR for pgi |
pgiR | GCGTCTAGAAGCGACTACCTATTTGCG (XbaI), PCR for pgi |
pfkF | CGAGTCGACAAGGAGGAAGACATGCGAATTGC (SalI), PCR for pfkA |
pfkR | CCGCTGCA GACTATCCAAACATTGCCTG (PstI), PCR for pfkA |
gapF | GGCGGTACCAGGAGACACAACATGACCATT (KpnI), PCR for gap |
gapR | CGGGGATCCATTAGAGCTTGGAAGCTACGAG (BamHI), PCR for gap |
pykF | CGAGAGCTCAAGGAGTAGGCTTATGGGCGT (SacI), PCR for pyk |
pykR | CCGGGTACCGATTAGAGCTTTGCAATCCT (KpnI), PCR for pyk |
pycF | CGCGAGCTCAAGGAGTACTCTAGTGTCGACTCACACAT (SacI), PCR for pyc |
pycR | CGCTCTAGATTAGGAAACGACGACGAT (XbaI), PCR for pyc |
gltF | GCCGAATTCAAGGAGAACAAATATGTTTGAAAG (EcoRI), PCR for gltA |
gltR | GTAGAGCTCTTTAGCGCTCCTCGCGAGGAACCAACT (SacI), PCR for gltA |
gdhF | CGCTCTAGAAAGGAGGAAATCATGACAGTTG (XbaI), PCR for gdh |
gdhR | CGGGTCGACTCTTAGATGACGCCCTGT (SalI), PCR for gdh |
gapCF | GCGGGTACCGGAGGTAGTTAGAATGGCAAAGATAGC (KpnI), PCR for gapC |
gapCR | CGCGGATCCCTATTTTGCTATT (BamHI), PCR for gapC |
rocF | CCGTCTAGAGGAGGGAAAAAGATGTCAGCAA (XbaI), PCR for rocG |
rocR | GGCGTCGACAAATTAGACCCATCCG (SalI), PCR for rocG |
ptac-rocGF | GCTCTAGATGAGCTGTTGACAATTAATCATCGTGTGGTACCATGTGTGGAATTGTGAGCGGATAACAATTGGAGGGAAAAAGATGTCAGCAA (XbaI), PCR for the PtacM-rocG fragment |
rocGR | GCTGATCCTCTAGAAAATTAGACCCATCCG (XbaI), PCR for the PtacM-rocG fragment |
ptac-gapCF | CGACTAGTTGAGCTGTTGACAATTAATCATCGTGTGGTACCATGTGTGGAATTGTGAGCGGATAACAATTAAGGAGGAGTTAGAATGGCAAAGATAGC (speI), PCR for the PtacM-gapC fragment |
gapCR2 | CGCTCTAGACTATTTTGCTATT(XbaI), PCR for the PtacM-gapC fragment |
zwfF | ACCCGCAGGATAAACGA, qRT-PCR for zwf |
zwfR | GCTAGATCATAAATGGC, qRT-PCR for zwf |
ppnkF | GTTTACCGACCGACTTGTG, qRT-PCR for ppnK |
ppnkR | GCTGACCTGGGATCTTTATT, qRT-PCR for ppnK |
icdF | AGGACCAGGGCTACGACAT, PCR for icd |
icdR | GCGGAACCCTTAACAGC, PCR for icd |
gndF | AACCGCAGCACTGACAAA, PCR for gnd |
gndR | CAGGGATGCTACGAACTCT, PCR for gnd |
16s-F | TCGATGCAACGCGAAGAAC, PCR for 16 sRNA |
16s-R | GAACCGACCACAAGGGAAAAC, PCR for 16sRNA |
Primer . | Sequencea and purpose (5′–3′) . |
---|---|
sacBF | CGGCGACTAGTTGAGCTGTTGACAATTAATCATCGTGTGGTACCATGTGTGGAATTGTGAGCGGATAACAATTCCGCGGGTTCTTTAGGCCCGTAGTCT (SpeI, SacII), PCR for sacB |
sacBR | GCCGCGATATCTCTCGTGATGGCAGGTT (EcoRV), PCR for sacB |
pk18msF | GCGCCGATATCGTTCGTCTGGAAGGCAGTA (EcoRV), PCR for the backbone of pK18mobsacB except for sacB |
pk18msR | GCGCGACTAGTGCATGGGCATAAAGTTGC (SpeI), PCR for the backbone of pK18mobsacB except for sacB |
speEF5 | CGATGGATCCCGACCGCTACAAGGCATAA (BamHI), deletion of speE |
speER5 | GCGTCTAGAGCGGAAATAATGGCGAAA (XbaI), deletion of speE |
speEF3 | CGCTCTAGACGATTCTGCCTCTGGATTA (XbaI), deletion of speE |
speER3 | CGATGTCGACCACCATCTGCCCAACG (SalI), deletion of speE |
argRF5 | CGCTGGATCCTTTAAGCACGGCGTTATTT (BamHI), deletion of argR |
argRR5 | CGGTCTAGATGCGAGTCACGGGATTTA (XbaI), deletion of argR |
argRF3 | CGGTCTAGAGGTAAGGTATAACCCGAGTGT (XbaI), deletion of argR |
argRR3 | CGATGTCGACGACTTGATGCCCACGAGA (SalI), deletion of argR |
pgiF | GTAGGATCCAGGAGTTTTCATGGCGGAC (BamHI), PCR for pgi |
pgiR | GCGTCTAGAAGCGACTACCTATTTGCG (XbaI), PCR for pgi |
pfkF | CGAGTCGACAAGGAGGAAGACATGCGAATTGC (SalI), PCR for pfkA |
pfkR | CCGCTGCA GACTATCCAAACATTGCCTG (PstI), PCR for pfkA |
gapF | GGCGGTACCAGGAGACACAACATGACCATT (KpnI), PCR for gap |
gapR | CGGGGATCCATTAGAGCTTGGAAGCTACGAG (BamHI), PCR for gap |
pykF | CGAGAGCTCAAGGAGTAGGCTTATGGGCGT (SacI), PCR for pyk |
pykR | CCGGGTACCGATTAGAGCTTTGCAATCCT (KpnI), PCR for pyk |
pycF | CGCGAGCTCAAGGAGTACTCTAGTGTCGACTCACACAT (SacI), PCR for pyc |
pycR | CGCTCTAGATTAGGAAACGACGACGAT (XbaI), PCR for pyc |
gltF | GCCGAATTCAAGGAGAACAAATATGTTTGAAAG (EcoRI), PCR for gltA |
gltR | GTAGAGCTCTTTAGCGCTCCTCGCGAGGAACCAACT (SacI), PCR for gltA |
gdhF | CGCTCTAGAAAGGAGGAAATCATGACAGTTG (XbaI), PCR for gdh |
gdhR | CGGGTCGACTCTTAGATGACGCCCTGT (SalI), PCR for gdh |
gapCF | GCGGGTACCGGAGGTAGTTAGAATGGCAAAGATAGC (KpnI), PCR for gapC |
gapCR | CGCGGATCCCTATTTTGCTATT (BamHI), PCR for gapC |
rocF | CCGTCTAGAGGAGGGAAAAAGATGTCAGCAA (XbaI), PCR for rocG |
rocR | GGCGTCGACAAATTAGACCCATCCG (SalI), PCR for rocG |
ptac-rocGF | GCTCTAGATGAGCTGTTGACAATTAATCATCGTGTGGTACCATGTGTGGAATTGTGAGCGGATAACAATTGGAGGGAAAAAGATGTCAGCAA (XbaI), PCR for the PtacM-rocG fragment |
rocGR | GCTGATCCTCTAGAAAATTAGACCCATCCG (XbaI), PCR for the PtacM-rocG fragment |
ptac-gapCF | CGACTAGTTGAGCTGTTGACAATTAATCATCGTGTGGTACCATGTGTGGAATTGTGAGCGGATAACAATTAAGGAGGAGTTAGAATGGCAAAGATAGC (speI), PCR for the PtacM-gapC fragment |
gapCR2 | CGCTCTAGACTATTTTGCTATT(XbaI), PCR for the PtacM-gapC fragment |
zwfF | ACCCGCAGGATAAACGA, qRT-PCR for zwf |
zwfR | GCTAGATCATAAATGGC, qRT-PCR for zwf |
ppnkF | GTTTACCGACCGACTTGTG, qRT-PCR for ppnK |
ppnkR | GCTGACCTGGGATCTTTATT, qRT-PCR for ppnK |
icdF | AGGACCAGGGCTACGACAT, PCR for icd |
icdR | GCGGAACCCTTAACAGC, PCR for icd |
gndF | AACCGCAGCACTGACAAA, PCR for gnd |
gndR | CAGGGATGCTACGAACTCT, PCR for gnd |
16s-F | TCGATGCAACGCGAAGAAC, PCR for 16 sRNA |
16s-R | GAACCGACCACAAGGGAAAAC, PCR for 16sRNA |
aRestriction enzyme cleavage sites are underlined
Primers used in this study
Primer . | Sequencea and purpose (5′–3′) . |
---|---|
sacBF | CGGCGACTAGTTGAGCTGTTGACAATTAATCATCGTGTGGTACCATGTGTGGAATTGTGAGCGGATAACAATTCCGCGGGTTCTTTAGGCCCGTAGTCT (SpeI, SacII), PCR for sacB |
sacBR | GCCGCGATATCTCTCGTGATGGCAGGTT (EcoRV), PCR for sacB |
pk18msF | GCGCCGATATCGTTCGTCTGGAAGGCAGTA (EcoRV), PCR for the backbone of pK18mobsacB except for sacB |
pk18msR | GCGCGACTAGTGCATGGGCATAAAGTTGC (SpeI), PCR for the backbone of pK18mobsacB except for sacB |
speEF5 | CGATGGATCCCGACCGCTACAAGGCATAA (BamHI), deletion of speE |
speER5 | GCGTCTAGAGCGGAAATAATGGCGAAA (XbaI), deletion of speE |
speEF3 | CGCTCTAGACGATTCTGCCTCTGGATTA (XbaI), deletion of speE |
speER3 | CGATGTCGACCACCATCTGCCCAACG (SalI), deletion of speE |
argRF5 | CGCTGGATCCTTTAAGCACGGCGTTATTT (BamHI), deletion of argR |
argRR5 | CGGTCTAGATGCGAGTCACGGGATTTA (XbaI), deletion of argR |
argRF3 | CGGTCTAGAGGTAAGGTATAACCCGAGTGT (XbaI), deletion of argR |
argRR3 | CGATGTCGACGACTTGATGCCCACGAGA (SalI), deletion of argR |
pgiF | GTAGGATCCAGGAGTTTTCATGGCGGAC (BamHI), PCR for pgi |
pgiR | GCGTCTAGAAGCGACTACCTATTTGCG (XbaI), PCR for pgi |
pfkF | CGAGTCGACAAGGAGGAAGACATGCGAATTGC (SalI), PCR for pfkA |
pfkR | CCGCTGCA GACTATCCAAACATTGCCTG (PstI), PCR for pfkA |
gapF | GGCGGTACCAGGAGACACAACATGACCATT (KpnI), PCR for gap |
gapR | CGGGGATCCATTAGAGCTTGGAAGCTACGAG (BamHI), PCR for gap |
pykF | CGAGAGCTCAAGGAGTAGGCTTATGGGCGT (SacI), PCR for pyk |
pykR | CCGGGTACCGATTAGAGCTTTGCAATCCT (KpnI), PCR for pyk |
pycF | CGCGAGCTCAAGGAGTACTCTAGTGTCGACTCACACAT (SacI), PCR for pyc |
pycR | CGCTCTAGATTAGGAAACGACGACGAT (XbaI), PCR for pyc |
gltF | GCCGAATTCAAGGAGAACAAATATGTTTGAAAG (EcoRI), PCR for gltA |
gltR | GTAGAGCTCTTTAGCGCTCCTCGCGAGGAACCAACT (SacI), PCR for gltA |
gdhF | CGCTCTAGAAAGGAGGAAATCATGACAGTTG (XbaI), PCR for gdh |
gdhR | CGGGTCGACTCTTAGATGACGCCCTGT (SalI), PCR for gdh |
gapCF | GCGGGTACCGGAGGTAGTTAGAATGGCAAAGATAGC (KpnI), PCR for gapC |
gapCR | CGCGGATCCCTATTTTGCTATT (BamHI), PCR for gapC |
rocF | CCGTCTAGAGGAGGGAAAAAGATGTCAGCAA (XbaI), PCR for rocG |
rocR | GGCGTCGACAAATTAGACCCATCCG (SalI), PCR for rocG |
ptac-rocGF | GCTCTAGATGAGCTGTTGACAATTAATCATCGTGTGGTACCATGTGTGGAATTGTGAGCGGATAACAATTGGAGGGAAAAAGATGTCAGCAA (XbaI), PCR for the PtacM-rocG fragment |
rocGR | GCTGATCCTCTAGAAAATTAGACCCATCCG (XbaI), PCR for the PtacM-rocG fragment |
ptac-gapCF | CGACTAGTTGAGCTGTTGACAATTAATCATCGTGTGGTACCATGTGTGGAATTGTGAGCGGATAACAATTAAGGAGGAGTTAGAATGGCAAAGATAGC (speI), PCR for the PtacM-gapC fragment |
gapCR2 | CGCTCTAGACTATTTTGCTATT(XbaI), PCR for the PtacM-gapC fragment |
zwfF | ACCCGCAGGATAAACGA, qRT-PCR for zwf |
zwfR | GCTAGATCATAAATGGC, qRT-PCR for zwf |
ppnkF | GTTTACCGACCGACTTGTG, qRT-PCR for ppnK |
ppnkR | GCTGACCTGGGATCTTTATT, qRT-PCR for ppnK |
icdF | AGGACCAGGGCTACGACAT, PCR for icd |
icdR | GCGGAACCCTTAACAGC, PCR for icd |
gndF | AACCGCAGCACTGACAAA, PCR for gnd |
gndR | CAGGGATGCTACGAACTCT, PCR for gnd |
16s-F | TCGATGCAACGCGAAGAAC, PCR for 16 sRNA |
16s-R | GAACCGACCACAAGGGAAAAC, PCR for 16sRNA |
Primer . | Sequencea and purpose (5′–3′) . |
---|---|
sacBF | CGGCGACTAGTTGAGCTGTTGACAATTAATCATCGTGTGGTACCATGTGTGGAATTGTGAGCGGATAACAATTCCGCGGGTTCTTTAGGCCCGTAGTCT (SpeI, SacII), PCR for sacB |
sacBR | GCCGCGATATCTCTCGTGATGGCAGGTT (EcoRV), PCR for sacB |
pk18msF | GCGCCGATATCGTTCGTCTGGAAGGCAGTA (EcoRV), PCR for the backbone of pK18mobsacB except for sacB |
pk18msR | GCGCGACTAGTGCATGGGCATAAAGTTGC (SpeI), PCR for the backbone of pK18mobsacB except for sacB |
speEF5 | CGATGGATCCCGACCGCTACAAGGCATAA (BamHI), deletion of speE |
speER5 | GCGTCTAGAGCGGAAATAATGGCGAAA (XbaI), deletion of speE |
speEF3 | CGCTCTAGACGATTCTGCCTCTGGATTA (XbaI), deletion of speE |
speER3 | CGATGTCGACCACCATCTGCCCAACG (SalI), deletion of speE |
argRF5 | CGCTGGATCCTTTAAGCACGGCGTTATTT (BamHI), deletion of argR |
argRR5 | CGGTCTAGATGCGAGTCACGGGATTTA (XbaI), deletion of argR |
argRF3 | CGGTCTAGAGGTAAGGTATAACCCGAGTGT (XbaI), deletion of argR |
argRR3 | CGATGTCGACGACTTGATGCCCACGAGA (SalI), deletion of argR |
pgiF | GTAGGATCCAGGAGTTTTCATGGCGGAC (BamHI), PCR for pgi |
pgiR | GCGTCTAGAAGCGACTACCTATTTGCG (XbaI), PCR for pgi |
pfkF | CGAGTCGACAAGGAGGAAGACATGCGAATTGC (SalI), PCR for pfkA |
pfkR | CCGCTGCA GACTATCCAAACATTGCCTG (PstI), PCR for pfkA |
gapF | GGCGGTACCAGGAGACACAACATGACCATT (KpnI), PCR for gap |
gapR | CGGGGATCCATTAGAGCTTGGAAGCTACGAG (BamHI), PCR for gap |
pykF | CGAGAGCTCAAGGAGTAGGCTTATGGGCGT (SacI), PCR for pyk |
pykR | CCGGGTACCGATTAGAGCTTTGCAATCCT (KpnI), PCR for pyk |
pycF | CGCGAGCTCAAGGAGTACTCTAGTGTCGACTCACACAT (SacI), PCR for pyc |
pycR | CGCTCTAGATTAGGAAACGACGACGAT (XbaI), PCR for pyc |
gltF | GCCGAATTCAAGGAGAACAAATATGTTTGAAAG (EcoRI), PCR for gltA |
gltR | GTAGAGCTCTTTAGCGCTCCTCGCGAGGAACCAACT (SacI), PCR for gltA |
gdhF | CGCTCTAGAAAGGAGGAAATCATGACAGTTG (XbaI), PCR for gdh |
gdhR | CGGGTCGACTCTTAGATGACGCCCTGT (SalI), PCR for gdh |
gapCF | GCGGGTACCGGAGGTAGTTAGAATGGCAAAGATAGC (KpnI), PCR for gapC |
gapCR | CGCGGATCCCTATTTTGCTATT (BamHI), PCR for gapC |
rocF | CCGTCTAGAGGAGGGAAAAAGATGTCAGCAA (XbaI), PCR for rocG |
rocR | GGCGTCGACAAATTAGACCCATCCG (SalI), PCR for rocG |
ptac-rocGF | GCTCTAGATGAGCTGTTGACAATTAATCATCGTGTGGTACCATGTGTGGAATTGTGAGCGGATAACAATTGGAGGGAAAAAGATGTCAGCAA (XbaI), PCR for the PtacM-rocG fragment |
rocGR | GCTGATCCTCTAGAAAATTAGACCCATCCG (XbaI), PCR for the PtacM-rocG fragment |
ptac-gapCF | CGACTAGTTGAGCTGTTGACAATTAATCATCGTGTGGTACCATGTGTGGAATTGTGAGCGGATAACAATTAAGGAGGAGTTAGAATGGCAAAGATAGC (speI), PCR for the PtacM-gapC fragment |
gapCR2 | CGCTCTAGACTATTTTGCTATT(XbaI), PCR for the PtacM-gapC fragment |
zwfF | ACCCGCAGGATAAACGA, qRT-PCR for zwf |
zwfR | GCTAGATCATAAATGGC, qRT-PCR for zwf |
ppnkF | GTTTACCGACCGACTTGTG, qRT-PCR for ppnK |
ppnkR | GCTGACCTGGGATCTTTATT, qRT-PCR for ppnK |
icdF | AGGACCAGGGCTACGACAT, PCR for icd |
icdR | GCGGAACCCTTAACAGC, PCR for icd |
gndF | AACCGCAGCACTGACAAA, PCR for gnd |
gndR | CAGGGATGCTACGAACTCT, PCR for gnd |
16s-F | TCGATGCAACGCGAAGAAC, PCR for 16 sRNA |
16s-R | GAACCGACCACAAGGGAAAAC, PCR for 16sRNA |
aRestriction enzyme cleavage sites are underlined
The suicide vector pK18mobsacB [25] was modified to improve its efficiency. The lethality of the expression of sacB depends on its level of expression in corynebacteria [9]. Therefore, a 1.85-kb DNA fragment containing the sacB cluster was amplified from pK18mobsacB [25] DNA using PCR and the primers sacBF and sacBR (Table 2). This converted the native promoter of the sacB cluster to the tac-M promoter, which is a strong promoter in corynebacteria [32]. The entire backbone of pK18mobsacB, except for sacB, was amplified using the primers pk18msF and pk18msR (Table 2). The two fragments were digested with SpeI and EcoRV and ligated together to form the inducible suicide vector pK-JL (5,570 bp).
The disruption of genes was performed using the non-replicable integration vector pK18mobsacB or pK-JL, which allows for marker-free deletion of the target gene [25]. For the construction of pK18mobsacB-ΔspeE, the flanking sequences of speE were amplified from C. glutamicum ATCC 13032 using the primers speEF5/speER5 and speEF3/speER3. The product and pK18mobsacB DNAs were digested with BamHI/XbaI, XbaI/SalI, and BamHI/SalI, respectively, and then ligated to generate pK18mobsacB-ΔspeE. The construction of pK18mobsacB-ΔargR was similar, but the primers argRF5/argRR5 and argRF3/argRR3 were used. For the construction of pK-ΔspeE::rocG, the primers speEF5 and speER3 were used with pK18mobsacB-ΔspeE as template. The resulting fragment was ligated to the pMD18-T simple vector to generate pMD18-T-ΔspeE. The rocG fragment was amplified using primers ptac-rocGF/rocGR and pEG-rocG DNA as the template. The resulting fragment, Ptac-M-rocG, was digested with XbaI and inserted into the cognate site of pMD18-T-ΔspeE. The ΔspeE::Ptac-M-rocG fragment was cleaved from the resulting plasmid and was ligated to BamHI/SalI-digested pK-JL to obtain pK-ΔspeE::rocG. For the construction of pK-ΔargR::gapC, primers argR5 and argR3 were used with pK18mobsacB-ΔargR DNA as template. The resulting fragment was ligated to the pMD18-T simple vector to generate pMD18-T-ΔargR. The gapC fragment was amplified using the primers ptac-gapF and gapCR2 with pEG-gapC DNA as the template. The resulting fragment, Ptac-M-gapC, was digested with XbaI and inserted into the cognate site of pMD18-T-ΔargR. The ΔargR::Ptac-M-gapC fragment was cleaved from the resulting plasmid using BamHI/SalI and was ligated to BamHI/SalI-digested pK-JL DNA to obtain pK-ΔargR::gapC.
These nonreplicable integration vectors, including pK18mobsacB-ΔspeE, pK18mobsacB-ΔargR, pK-ΔspeE::rocG, and pK-ΔargR::gapC were used to transform C. glutamicum to disrupt the site-specific gene using the protocol described by Schäfer et al. [25].
Quantitative real-time PCR (qRT-PCR)
Total RNA from C. glutamicum grown for 54 h in shake flasks was isolated using an RNA extraction kit (Dongsheng Biotech, Guangzhou, China), following the manufacturer’s instructions. The first-strand cDNA was synthesized using an All-in-One™ First-Strand cDNA Synthesis Kit (GeneCopoeia, Guangzhou, China). qRT-PCR was performed using the All-in-One™ qPCR Mix kit (GeneCopoeia, Guangzhou, China) on an iCycler iQ5 Real-Time PCR system (Bio-Rad Laboratories, Richmond, CA, USA). One hundred nanograms of cDNA was used as template. The PCR conditions were as follows: 95 °C for 10 min, followed by 45 cycles of denaturation at 95 °C for 10 s, annealing at 60 °C for 20 s, and extension at 72 °C for 15 s. The primers for qRT-PCR are listed in Table 2. The 2−ΔΔCt quantification technique was used to analyze data [19]. The data were normalized to the level of expression of 16S rRNA.
NADPH assay
After aerobic cultivation of C. glutamicum on a rotary shaker (150 rpm) at 30 °C for 54 h, the cells were harvested by centrifugation and washed twice with water. Intracellular NADPH was extracted and quantified using the Enzychrom™ NADP+/NADPH Assay kit (BioAssay Systems, Hayward, CA), following the manufacturer’s instructions.
Enzyme assay
Two-milliliter cell cultures at 50 h were harvested by centrifugation, washed twice with water, and subjected to sonication in 0.4 ml of 50 mM Tris–Cl (pH 7.5) containing 20 % glycerol, 100 mM NaCl, 1 mM EDTA, and 1 mM phenylmethylsulfonyl fluoride. Then the resulting broken-cells suspension was centrifuged for 15 min at 4 °C and 14,000 rpm to obtain cell-free extracts. Glutamate dehydrogenase activity was determined spectrophotometrically by measuring the oxidation of NADPH (or NADH) at a wavelength of 340 nm and 25 °C, as described previously [1]. The standard reaction mixture contained 2.9 ml of 55 mM Tris–Cl (pH 7.5) containing 2 % glycerol, 10 mM NaCl, 100 mM NH4Cl, 10 mM 2-ketoglutarate, 0.2 mM NADPH (or NADH), and 100 μl crude extract. Reaction was initiated by the addition of the cell-free extracts. One unit of glutamate dehydrogenase activity was defined as the amount of enzyme that catalyzed the oxidation of 1 mmol NADPH (or NADH) in 1 min under the above conditions. Glyceraldehyde-3-phosphate dehydrogenase activity was determined spectrophotometrically by measuring the reduction of NAD (or NADP) at a wavelength of 340 nm and 25 °C, as described previously [22]. The standard reaction mixture contained 2.9 ml of 50 mM Tris–Cl (pH 8.5) containing 3 mM β-mercaptoethanol, 1 mM NADP, 1 mM dl-glyceraldehyde 3-phosphate, 1 mM H3PO4, and 100 μl crude extract. Reaction was initiated by the addition of the cell-free extracts. One unit of glyceraldehyde-3-phosphate dehydrogenase activity was defined as the amount of enzyme that catalyzed the reduction of 1 mmol NAD (or NADP) in 1 min under the above conditions.
Analysis of cell growth and ornithine
Cell growth was monitored by measuring the optical density of the culture at 600 nm (OD600) using a spectrophotometer (UV2450, Shimadzu Corporation, Japan) after adding 0.2 mol l−1 HCl to dissolve CaCO3. DCW was estimated according to the formula 1 OD600 = 0.28 g DCW l−1 [8]. l-Ornithine concentrations were determined by colorimetry using ninhydrin as described previously [2].
Statistical analysis
All experiments were conducted in triplicate, and data were averaged and presented as mean ± standard deviation (SD). One-way analysis of variance (ANOVA) followed by Tukey’s test was used to determine significant differences using OriginPro (version 7.5) software. Statistical significance was defined as p < 0.05.
Results and discussion
Effect of expression of genes involved in glutamate biosynthesis on the production of l-ornithine
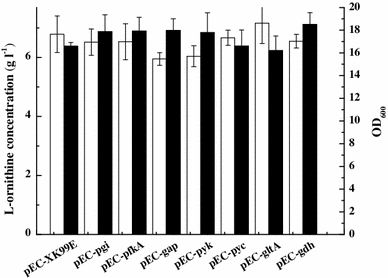
Effect of overexpression of genes encoding enzymes in the upstream pathway of glutamate biosynthesis on l-ornithine production in C. glutamicum ΔAP. OD600 (white bars); l-ornithine concentration (black bars). Cells were cultured in the fermentation medium 25 μg ml−1 Kan and 0.5 mM IPTG at 30 °C for 72 h. Data are mean ± SD for three replicates
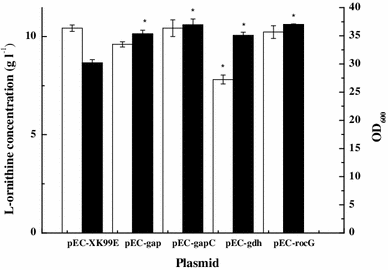
Effect of the overexpression of genes encoding enzymes requiring NADPH on l-ornithine production by C. glutamicum ΔAP. OD600 (white bars); l-ornithine concentration (black bars). Cells were cultured in the fermentation medium 25 μg ml−1 Kan and 0.5 mM IPTG at 30 °C for 72 h. Data are mean ± SD for three replicates. *Significant different at P < 0.01 compared with corresponding controls
Glyceraldehyde-3-phosphate dehydrogenase, catalyzing oxidation of D-glyceraldehyde-3-phosphate into 1,3-diphosphoglycerate, is a key enzyme of glycolysis and plays a crucial role in catabolic carbohydrate metabolism. Overexpression of NAD-dependent glyceraldehyde-3-phosphate dehydrogenase can drive more metabolic flux of carbon into the Embden–Meyerhof–Parnas (EMP) pathway, hence improving the internal glutamate pool. Overexpression of gdh in C. glutamicum resulted in an increased internal glutamate pool [11]. The increased internal glutamate pool resulted in enhanced l-ornithine production.
C. acetobutylicum gapC encodes NADP-dependent glyceraldehyde-3-phosphate dehydrogenase. The GapC catalyzes glyceraldehyde-3-phosphate and NADP to form 3-phospho-d-glyceroyl phosphate and NADPH. Overexpression of the gapC gene results in an increase of the intracellular NADPH concentration. Overexpression of C. acetobutylicum gapC increases the production of CoQ10 [5]. The overexpression of C. acetobutylicum gapC together with the knockout of the endogenous gapA gene improves lycopene and ε-caprolactone production [22]. B. subtilis rocG encodes an NAD-dependent glutamate dehydrogenase that converts 2-oxoglutarate to glutamate in the presence of NADH. The expression of rocG provides more NADPH for l-ornithine production as an outcome of the consumption of 2-oxoglutarate by the NADPH-independent reaction.
Figure 2 also shows that overexpression of the gdh gene caused significant inhibition of growth. Kholy et al. [11] reported the same result. C. glutamicum overexpressing the gdh gene showed somewhat slower growth on glucose and acetate medium than that of the wild type. The overexpression of the other three genes did not inhibit cell growth. Thus, the genes gapC and rocG were selected for further study.
Gene deletion strategy to increase l-ornithine production
Our previous proteome analysis [20] demonstrated that spermidine synthase encoded by speE was upregulated in the l-ornithine-producing strain C. glutamicum compared with wild type. We reasoned that this upregulation might result in the degradation of l-ornithine. Therefore, we deleted speE from C. glutamicum ΔAP to generate C. glutamicum ΔAPE. Deletion of speE enhanced the production of l-ornithine. Strain ΔAPE produced 10.87 ± 0.37 g l−1 of l-ornithine, which is 12.0 % higher than that of C. glutamicum ΔAP (9.71 ± 0.18 g l−1; Table 3).
l-Ornithine production by the engineered strains
Strain . | OD600 . | l-Ornithine (g l−1) . | NADPH (μmol g−1) . |
---|---|---|---|
ΔAP | 26.23 ± 0.51 | 9.71 ± 0.18 | 2.13 ± 0.16 |
ΔAPE | 25.53 ± 0.65 | 10.87 ± 0.37 | 2.00 ± 0.42 |
ΔAPER | 13.65 ± 0.28 | 12.12 ± 0.57 | 3.04 ± 0.21 |
ΔAPER::gapC | 13.10 ± 0.21 | 13.23 ± 0.54 | 11.46 ± 0.63 |
ΔAPRE::rocG | 11.65 ± 0.99 | 14.84 ± 0.57 | 11.37 ± 0.16 |
ΔAPE::rocG-R::gapC | 12.50 ± 0.85 | 14.20 ± 0.71 | 11.46 ± 0.71 |
Strain . | OD600 . | l-Ornithine (g l−1) . | NADPH (μmol g−1) . |
---|---|---|---|
ΔAP | 26.23 ± 0.51 | 9.71 ± 0.18 | 2.13 ± 0.16 |
ΔAPE | 25.53 ± 0.65 | 10.87 ± 0.37 | 2.00 ± 0.42 |
ΔAPER | 13.65 ± 0.28 | 12.12 ± 0.57 | 3.04 ± 0.21 |
ΔAPER::gapC | 13.10 ± 0.21 | 13.23 ± 0.54 | 11.46 ± 0.63 |
ΔAPRE::rocG | 11.65 ± 0.99 | 14.84 ± 0.57 | 11.37 ± 0.16 |
ΔAPE::rocG-R::gapC | 12.50 ± 0.85 | 14.20 ± 0.71 | 11.46 ± 0.71 |
l-Ornithine production by the engineered strains
Strain . | OD600 . | l-Ornithine (g l−1) . | NADPH (μmol g−1) . |
---|---|---|---|
ΔAP | 26.23 ± 0.51 | 9.71 ± 0.18 | 2.13 ± 0.16 |
ΔAPE | 25.53 ± 0.65 | 10.87 ± 0.37 | 2.00 ± 0.42 |
ΔAPER | 13.65 ± 0.28 | 12.12 ± 0.57 | 3.04 ± 0.21 |
ΔAPER::gapC | 13.10 ± 0.21 | 13.23 ± 0.54 | 11.46 ± 0.63 |
ΔAPRE::rocG | 11.65 ± 0.99 | 14.84 ± 0.57 | 11.37 ± 0.16 |
ΔAPE::rocG-R::gapC | 12.50 ± 0.85 | 14.20 ± 0.71 | 11.46 ± 0.71 |
Strain . | OD600 . | l-Ornithine (g l−1) . | NADPH (μmol g−1) . |
---|---|---|---|
ΔAP | 26.23 ± 0.51 | 9.71 ± 0.18 | 2.13 ± 0.16 |
ΔAPE | 25.53 ± 0.65 | 10.87 ± 0.37 | 2.00 ± 0.42 |
ΔAPER | 13.65 ± 0.28 | 12.12 ± 0.57 | 3.04 ± 0.21 |
ΔAPER::gapC | 13.10 ± 0.21 | 13.23 ± 0.54 | 11.46 ± 0.63 |
ΔAPRE::rocG | 11.65 ± 0.99 | 14.84 ± 0.57 | 11.37 ± 0.16 |
ΔAPE::rocG-R::gapC | 12.50 ± 0.85 | 14.20 ± 0.71 | 11.46 ± 0.71 |
The expression of the arg operon that controls the l-ornithine biosynthetic pathway is regulated by the arginine repressor (ArgR). Further, the DNA-binding affinity of ArgR for the upstream of the argB gene plays an important role in the biosynthesis of l-ornithine biosynthesis by C. glutamicum [17]. Deletion of argR is another strategy for enhancing the level of expression of the arg operon. Thus, we deleted the argR gene of the C. glutamicum ΔAPE to generate C. glutamicum ΔAPER. C. glutamicum ΔAPER produced 12.12 ± 0.57 g l−1 of l-ornithine (Table 3), which was 11.5 % higher than that of C. glutamicum ΔAPE.
Effect of NADPH availability
To reduce the metabolic burden caused by plasmid replication, the genes gapC or rocG were integrated into the chromosome of C. glutamicum ΔAPER. The individual chromosomal integration of the gapC gene led to an increase in the concentration of l-ornithine from 12.12 ± 0.57 to 13.23 ± 0.54 g l−1 (P < 0.01; Table 3). The individual chromosomal integration of the rocG gene also led to an increase of l-ornithine concentration compared with C. glutamicum ΔAPER (14.84 ± 0.57 g l−1 vs 12.12 ± 0.57 g l−1; P < 0.01; Table 3). Moreover, these modifications resulted in a significant increase in the concentration of intracellular NADPH. However, the simultaneous integration of gapC and rocG genes did not further increase the intracellular concentrations of l-ornithine and NADPH. In order to understand the mechanism of l-ornithine production, we determined the transcriptional level of gapC and rocG, and their corresponding enzyme activities. The data are shown in Table 4. The individual chromosomal integration of the gapC gene led to a reduction of Gap enzyme activity, and increase of total glyceraldehyde-3-phosphate dehydrogenase activities. The expression of GapC enzyme resulted in the evaluated NADPH concentration. The individual chromosomal integration of the rocG gene led to a reduction of Gdh enzyme activity, and increase of total glutamate dehydrogenase activities, which can provide more NADPH for l-ornithine biosynthesis, and thereby improving l-ornithine production. The data shown in Table 4 also demonstrate that the level of transcription of the gapC gene and GapC activity in C. glutamicum ΔAPE::rocG-R::gapC was significantly different from that of C. glutamicum ΔAPER::gapC. However, the level of transcription of the rocG gene and RocG activity in C. glutamicum ΔAPE::rocG-R::gapC was not significantly different from that of C. glutamicum ΔAPRE::rocG.
Transcriptional levels of genes and specific activity of enzymes in the engineered strains
Strain . | Relative mRNA expressiona . | Specific glyceraldehyde-3-phosphate dehydrogenase activity (U mg−1 protein)a . | Specific glutamate dehydrogenase activity (U mg−1 protein)a . | |||||
---|---|---|---|---|---|---|---|---|
gapC . | rocG . | Gap . | GapC . | Total . | Gdh . | RocG . | Total . | |
ΔAPER | 205.2 | 205.2 | 8,077.7 | 8,077.7 | ||||
ΔAPER::gapC | 1.4 | 122.9 | 468.1 | 591.0 | ||||
ΔAPRE::rocG | 1.4 | 6,772.0 | 4,785.5 | 11,557.5 | ||||
ΔAPE::rocG-R::gapC | 1.6 | 1.4 | 106.8 | 516.2 | 623.0 | 6,774.9 | 4,800.7 | 11,575.6 |
Strain . | Relative mRNA expressiona . | Specific glyceraldehyde-3-phosphate dehydrogenase activity (U mg−1 protein)a . | Specific glutamate dehydrogenase activity (U mg−1 protein)a . | |||||
---|---|---|---|---|---|---|---|---|
gapC . | rocG . | Gap . | GapC . | Total . | Gdh . | RocG . | Total . | |
ΔAPER | 205.2 | 205.2 | 8,077.7 | 8,077.7 | ||||
ΔAPER::gapC | 1.4 | 122.9 | 468.1 | 591.0 | ||||
ΔAPRE::rocG | 1.4 | 6,772.0 | 4,785.5 | 11,557.5 | ||||
ΔAPE::rocG-R::gapC | 1.6 | 1.4 | 106.8 | 516.2 | 623.0 | 6,774.9 | 4,800.7 | 11,575.6 |
aValues are averages based on the results obtained with at least three independent experiments and the standard deviations were consistently <10 %
Transcriptional levels of genes and specific activity of enzymes in the engineered strains
Strain . | Relative mRNA expressiona . | Specific glyceraldehyde-3-phosphate dehydrogenase activity (U mg−1 protein)a . | Specific glutamate dehydrogenase activity (U mg−1 protein)a . | |||||
---|---|---|---|---|---|---|---|---|
gapC . | rocG . | Gap . | GapC . | Total . | Gdh . | RocG . | Total . | |
ΔAPER | 205.2 | 205.2 | 8,077.7 | 8,077.7 | ||||
ΔAPER::gapC | 1.4 | 122.9 | 468.1 | 591.0 | ||||
ΔAPRE::rocG | 1.4 | 6,772.0 | 4,785.5 | 11,557.5 | ||||
ΔAPE::rocG-R::gapC | 1.6 | 1.4 | 106.8 | 516.2 | 623.0 | 6,774.9 | 4,800.7 | 11,575.6 |
Strain . | Relative mRNA expressiona . | Specific glyceraldehyde-3-phosphate dehydrogenase activity (U mg−1 protein)a . | Specific glutamate dehydrogenase activity (U mg−1 protein)a . | |||||
---|---|---|---|---|---|---|---|---|
gapC . | rocG . | Gap . | GapC . | Total . | Gdh . | RocG . | Total . | |
ΔAPER | 205.2 | 205.2 | 8,077.7 | 8,077.7 | ||||
ΔAPER::gapC | 1.4 | 122.9 | 468.1 | 591.0 | ||||
ΔAPRE::rocG | 1.4 | 6,772.0 | 4,785.5 | 11,557.5 | ||||
ΔAPE::rocG-R::gapC | 1.6 | 1.4 | 106.8 | 516.2 | 623.0 | 6,774.9 | 4,800.7 | 11,575.6 |
aValues are averages based on the results obtained with at least three independent experiments and the standard deviations were consistently <10 %
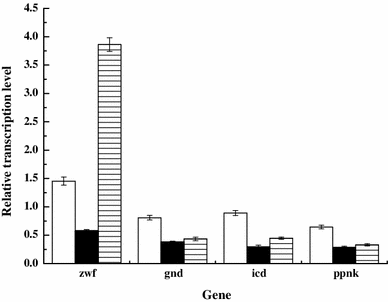
Levels of transcription of genes involved in NADPH biosynthesis in C. glutamicum ΔAPER::gapC, ΔAPRE::rocG, and ΔAPE::rocG-R::gapC compared with those in the parental strain, C. glutamicum ΔAPER. ΔAPER::gapC (white bars), ΔAPRE::rocG (black bars), and ΔAPE::rocG-R::gapC (hatched bars)
The present study demonstrates that the availability of NADPH and the production of l-ornithine are tightly linked. Production of l-ornithine by C. glutamicum requires 2 moles of NADPH per mole of l-ornithine. Other strategies have been developed to improve the availability of NADPH. The inactivation of the gene encoding gluconate kinase (gntK) was shown to lead to a 51.8 % increase in intracellular NADPH concentration and a 49.9 % increase in l-ornithine production [7]. They also demonstrated that excess NADPH is not necessarily rate-limiting, but is required for increased l-ornithine production in C. glutamicum. Overexpression of E. coli pntAB, which encodes a membrane-bound transhydrogenase, enhances the availability of NADPH and thus increases the levels of l-lysine in C. glutamicum [10]. Replacement of the endogenous NAD-dependent glyceraldehyde-3-phosphate dehydrogenase with the NADP-dependent glyceraldehyde-3-phosphate dehydrogenase from Streptococcus mutans also increases the availability of NADPH and l-lysine in C. glutamicum [30]. Overexpression of nadk, which encodes NAD kinase, increases the NADPH/NADP ratio, which in turn enhances thymidine biosynthesis in E. coli [14]. Simultaneous chromosomal overexpression of transhydrogenase (pntAB) and NAD kinase (yfjB) genes had an effect on increasing NADPH supply and improving anaerobic isobutanol production [27]. These strategies, which are being explored in our laboratory, may be useful for further improving the production of l-ornithine by C. glutamicum ΔAPRE::rocG.
In conclusion, we implemented strategies to enhance l-ornithine production by C. glutamicum that were suggested by the results of our previous proteomic analysis [21]. Overexpression of C. acetobutylicum gapC and B. subtilis rocG resulted in increased production of l-ornithine, because these genetic manipulations provided increased levels of NADPH for l-ornithine biosynthesis. The increased levels of NADPH resulted from the expression of the gapC or rocG gene rather than that of the genes (gnd, icd, and ppnK) involved in NADPH biosynthesis. To the best of our knowledge, this is the first report on the expression of gapC and rocG to enhance the production of l-ornithine by C. glutamicum.
Acknowledgments
We are grateful to the National Natural Science Foundation of China (grant nos. 30970089, 20876181, 21276289) and the Natural Science Foundation of Guangdong Province (nos. 9351027501000003, S2011010001396) for their financial support.