-
PDF
- Split View
-
Views
-
Cite
Cite
Silvia Galafassi, Annamaria Merico, Francesca Pizza, Linda Hellborg, Francesco Molinari, Jure Piškur, Concetta Compagno, Dekkera/Brettanomyces yeasts for ethanol production from renewable sources under oxygen-limited and low-pH conditions, Journal of Industrial Microbiology and Biotechnology, Volume 38, Issue 8, 1 August 2011, Pages 1079–1088, https://doi.org/10.1007/s10295-010-0885-4
- Share Icon Share
Abstract
Industrial fermentation of lignocellulosic hydrolysates to ethanol requires microorganisms able to utilise a broad range of carbon sources and generate ethanol at high yield and productivity. D. bruxellensis has recently been reported to contaminate commercial ethanol processes, where it competes with Saccharomyces cerevisiae [4, 26]. In this work Brettanomyces/Dekkera yeasts were studied to explore their potential to produce ethanol from renewable sources under conditions suitable for industrial processes, such as oxygen-limited and low-pH conditions. Over 50 strains were analysed for their ability to utilise a variety of carbon sources, and some strains grew on cellobiose and pentoses. Two strains of D. bruxellensis were able to produce ethanol at high yield (0.44 g g−1 glucose), comparable to those reported for S. cerevisiae. B. naardenensis was shown to be able to produce ethanol from xylose. To obtain ethanol from synthetic lignocellulosic hydrolysates we developed a two-step fermentation strategy: the first step under aerobic conditions for fast production of biomass from mixtures of hexoses and pentoses, followed by a second step under oxygen limitation to promote ethanol production. Under these conditions we obtained biomass and ethanol production on synthetic lignocellulosic hydrolysates, with ethanol yields ranging from 0.2 to 0.3 g g−1 sugar. Hexoses, xylose and arabinose were consumed at the end of the process, resulting in 13 g l−1 of ethanol, even in the presence of furfural. Our studies showed that Brettanomyces/Dekkera yeasts have clear potential for further development for industrial processes aimed at production of ethanol from renewable sources.
Introduction
Agricultural wastes and forest biomass represent attractive substrates for production of fuel ethanol. Hydrolysis of cellulose and hemicellulose produces a mixture of hexoses, as glucose, galactose and mannose, and pentoses, as xylose and arabinose. Fermentation of this mixture of sugars has been a challenge for strain development, because complete substrate utilisation is one of the prerequisites for economic feasibility of such processes in industrial applications. During the steps of pre-treatment and hydrolysis, low-molecular-weight organic acids, furans and aromatics are released, and these compounds are potent inhibitors of microbial metabolism [23, 24]. Industrial fermentation of lignocellulosic hydrolysates to ethanol therefore requires microorganisms which can utilise a broad range of carbon sources, producing ethanol at high yield and productivity, but also which are able to tolerate high ethanol concentrations and inhibitors.
Wild-type strains of Saccharomyces cerevisiae are well established for industrial ethanol production from sugar cane and starch-based plants, but they cannot utilise pentoses. Due to the large knowhow and availability of molecular tools, during recent years S. cerevisiae has been the preferred species for metabolic engineering aimed at introducing the ability to ferment pentose sugars [8, 32]. Recombinant xylose-fermenting strains of S. cerevisiae have recently been described to approach economically feasible ethanol production from undetoxified hydrolysates [21, 22]. These strains over-produce Pichia stipitis xylose reductase (XR) and xylitol dehydrogenase (XDH), and the non-oxidative pentose phosphate pathway (PPP) genes are over-expressed. Beside metabolic engineering strategies, also evolutionary engineering has been used to obtain S. cerevisiae strains possessing suitable characteristics for industrial employment [18]. Industrial recombinant arabinose-fermenting S. cerevisiae strains have been reported, too [14].
So far, only a few other yeasts have been characterised for their fermentative metabolism on pentose sugars, such as P. stipitis, Candida shehatae and Pachysolen tannophilus [1, 5, 7, 27, 30]. Pichia stipitis exhibits great ability to produce ethanol from xylose [11, 12], but it is aerobic and sensitive to organic acids as well as to other inhibitors. Adaptation strategies have been employed to increase its tolerance [11].
The Brettanomyces/Dekkera complex is composed of five species: Dekkera bruxellensis, D. anomala, Brettanomyces naardenensis, B. nanus and B. custersianus. A couple of Brettanomyces/Dekkera strains have been reported to be able to utilise xylose [25, 34]. D. bruxellensis is a frequent and increasing problem in the wine industry, causing economic losses in the wine sector [6]. Interestingly, D. bruxellensis has recently been reported even to contaminate distilleries, specially in continuous fermentation systems, where this yeast can compete with S. cerevisiae, due to its ability to grow under anaerobic conditions and at high ethanol concentrations [4, 26]. However, in general this group of yeasts has been only poorly studied so far [2, 31].
In this work a large screening of Dekkera/Brettanomyces strains was carried out for their ability to grow on a variety of sugars as carbon sources. Here we report on physiological and biochemical characterisation of D. bruxellensis and B. naardenensis strains under different growth conditions suitable for industrial fermentation processes, such as oxygen-limited and low-pH conditions. Finally, we describe a two-step batch process to produce biomass and ethanol from synthetic lignocellulosic hydrolysates.
Materials and methods
Yeast strains
The strains used throughout this work belong to the Dekkera/Brettanomyces group, isolated from beer, wine and soft drinks in various part of the world. Twenty-eight strains of Dekkera bruxellensis (CBS 72, CBS 73, CBS 74, CBS 75, CBS 78, CBS 96, CBS 98, CBS 1940, CBS 1941, CBS 1942, CBS 1943, CBS 2336, CBS 2499, CBS 2547, CBS 2796, CBS 2797, CBS 3025, CBS 4459, CBS 4602, CBS 6055, CBS 4481, CBS 8027, CBS 4482, CBS 4601, CBS 4914, CBS 5206, CBS 5512, CBS 5513), 15 strains of Dekkera anomala (CBS 76, CBS 77, CBS 1938, CBS 3026, CBS 4210, CBS 4211, CBS 4212, CBS 4460, CBS 4608, CBS 7250, CBS 4711, CBS 4712, CBS 7654, CBS 8138, CBS 8139), 4 strains of Brettanomyces naardenensis (CBS 6040, CBS6 108, CBS 6115, CBS 7540), 2 strains of Brettanomyces nanus (CBS 1945, CBS 1947) and 5 strains of Brettanomyces custersianus (CBS 4805, CBS 4806, CBS 5207, CBS 5208, CBS 8347), all obtained from the Centraalbureau Voor Schimmel Culture Collection, were tested. The strains were stored at −80°C in 15% v/v glycerol and inoculated on YPD agar plates (yeast extract 10 g l−1, Bacto-peptone 20 g l−1, glucose 20 g l−1, agar 20 g l−1).
Carbon source utilisation on silica plates
Since we found that some strains can utilise agar or agarose as a carbon source, silica plates were made, composed of silica 83 g l−1, potassium hydroxide 58 g l−1, phosphoric acid 33 g l−1, Yeast Nitrogen Base w/o amino acids 5.8 g l−1 and the carbon source added to final concentration of 17 g l−1. The carbon sources tested were pentoses (d-xylose, xylitol, l-arabinose), hexoses (d-glucose, d-galactose, d-mannose), disaccharides (sucrose, maltose, cellobiose) and polysaccharide (starch). Strains were grown overnight in rich YPD medium (glucose, 20 g l−1, peptone 20 g l−1, yeast extract, 10 g l−1) and washed twice before being spotted (about 200 cells) onto silica plates with various carbon sources and a negative control without any carbon source. They were grown at 25°C up to 6 weeks. Each experiment was repeated twice. Growth was checked twice a week. To confirm species designation under the experiments, the cells were checked under microscope, and any “suspicious” cells were analysed for the ribosomal DNA sequence D1/D2 domain of 26S RNA [17].
Batch cultivations
Batch cultivations were performed in shake flasks and in a Biostat-Q system bioreactor (B-Braun) with working volume of 0.8 l. The temperature was set at 30°C and the stirring speed at 500 rpm. The medium used was a defined synthetic medium [33] with some modifications in the final concentrations, being as follows: ammonium sulphate, 5.0 g l−1; potassium dihydrogen phosphate, 3.0 g l−1; magnesium sulphate heptahydrate, 0.5 g l−1; ethylenediamine tetraacetic acid (EDTA), 22.5 mg l−1; zinc sulphate heptahydrate, 6.75 mg l−1; manganese chloride tetrahydrate, 1.5 mg l−1; cobalt(II) chloride hexahydrate, 0.45 mg l−1; copper(II) sulphate pentahydrate, 0.45 mg l−1; disodium molybdate dihydrate, 0.60 mg l−1; calcium chloride dihydrate, 6.75 mg l−1; iron sulphate heptahydrate, 4.5 mg l−1; boric acid, 1.5 mg l−1; potassium iodide, 0.15 mg l−1; d-(−)-biotin, 0.08 mg l−1; calcium d-(+)-panthotenate, 1.5 mg l−1; nicotinic acid, 1.5 mg l−1; myo-inositol, 37.5 mg l−1; thiamine hydrochloride, 1.5 mg l−1; pyridoxol hydrochloride, 1.5 mg l−1; p-aminobenzoic acid, 0.3 mg l−1. For cultivation under oxygen-limited conditions the medium was supplemented with ergosterol, 10 mg l−1; Tween-80, 420 mg l−1; uracil 50 mg l−1. Different carbon sources were used: d-glucose, 20 g l−1; d-xylose, 20 g l−1.
Biomass obtained from an overnight culture (pre-inoculum) on YPD medium was washed twice and used to inoculate the fermenter at biomass concentration of around 0.05 g dry cell weight (DCW) l−1. Neither air nor nitrogen was supplied during the experiments under oxygen-limited conditions, and the stirring speed was set at 400 rpm. At the time of inoculum, the dissolved oxygen concentration was 100% of the air saturation, but it dropped to 0%, due to cell respiration during growth. During cultivation of Dekkera bruxellensis strains CBS 2499 and CBS 2796, the pH decreased from the initial value of 5 to a final value of about 2.5, whereas during fermentation of Brettanomyces naardenensis CBS 7540, pH was maintained at 5 by automatic addition of 2 M KOH.
Two-step cultivations
In the two-step batch cultivations the first step (to obtain the biomass) was performed under controlled aerobic conditions and started with 400 ml initial volume. In the experiments with mixtures of glucose and xylose the medium was the defined synthetic one described above but containing d-glucose 10 g l−1 and d-xylose, 40 g l−1 (1:4 ratio). In the experiments performed with synthetic softwood hydrolysate, the concentration of carbon sources was: d-glucose 16 g l−1, d-mannose 10 g l−1, d-xylose 7 g l−1, galactose 4 g l−1, l-arabinose 3 g l−1 and acetic acid 2.5 g −1 (as reported in [15]), with or without addition of furfural (Sigma) 1.4 g l−1 [20]. To obtain aerobic conditions in the first step, the stirring speed was kept at 800 rpm and the air flow at 1 l min−1 (2.5 vvm) until complete exhaustion of the initial carbon sources [dissolved oxygen tension (DOT) over 20% of the saturation value]. At the end of this step (biomass approximately 10 g DCW l−1), to induce fermentative metabolism, the air flow and the stirring speed were lowered (to 400 rpm), obtaining different dissolved oxygen concentrations. In particular, air flows of 0.007, 0.016 and 0.032 vvm generated oxygen transfer rates (OTR) of 0.08, 0.12 and 0.21 mgoxygen l−1 min−1, respectively. In this second step, to restore the initial concentration of nutrients, 400 ml of two times concentrated fresh medium was added to the fermentor. In the two-step processes performed with the feeding, after the first step under aerobic conditions, 400 ml fresh medium was introduced by a Masterflex L/S 751920 pump (Cole Parmer) to achieve feeding flows of 0.04 gxylose gcells −1 h−1 and 0.01 gglucose gcells −1 h−1 at the lower flow rate, and 0.16 gxylose gcells −1 h−1and 0.04 gglucose gcells −1 h−1, at the higher flow rate. During the processes, pH was maintained at the value of 5 by addition of 2 M KOH. All fermentations were performed in triplicate.
Biomass and metabolites quantification
Samples were withdrawn from the fermenter at appropriate intervals and used to monitor cell growth by measuring optical density at 660 nm using a spectrophotometer, after appropriate dilution. For dry weight determination, washed culture samples were filtered on a 0.45-μm glass microfiber GF/A filter (Whatman) and dried at 80°C for 24 h. The concentration of extracellular metabolites, such as glucose, ethanol, glycerol, acetate, galactose and arabinose, in the supernatants were determined by commercial enzymatic kits (Roche). Xylose and xylitol were quantified by high-performance liquid chromatography (HPLC). Two different columns were used: a Polyspher OA-HY (Merck), followed by an Aminex Hpx-87P (Bio-Rad). The system was operated at 80°C using water as the mobile phase at flow rate of 0.5 ml min−1 and pressure of 60 bar. All assays were performed in triplicate, and standard deviations varied between 1 and 5%.
Specific consumption rates of glucose and specific production rates of ethanol, glycerol and acetic acid were calculated during the exponential phase of growth under oxygen-limited condition. In the experiments performed with xylose or with sugar mixtures, specific consumption rates of glucose and xylose as well as specific production rates of ethanol were calculated during the fermentation phase as the amount of sugar utilised or ethanol produced by the average biomass present during the time interval considered. The yields of ethanol and other metabolites relative to the consumed carbon sources were calculated as the total amount of produced metabolites divided by the total amount of sugars utilised.
Enzyme activity assays
Cell extracts were prepared by extraction with acid-washed glass beads (Sigma), and the total amount of extracted proteins was quantified using the Biorad Protein Assay kit (Bio-Rad). Alcohol dehydrogenase (ADH), pyruvate decarboxylase (PDC), acetaldehyde dehydrogenase (AcDH), acetyl coenzyme A (CoA) synthetase (ACS) and glucose 6-phosphate dehydrogenase (G6PDH) were assayed according to Ref. [28]. Cell extracts for glycerol 3-phosphate dehydrogenase (GPDH) were desalted [3] prior to the assay, which was performed in TrED buffer (triethanolamine 10 mmol l−1, EDTA 1 mmol l−1, dithiothreitol 1 mmol l−1, pH 7.5), MgCl2 1 mmol l−1 and nicotinamide adenine dinucleotide (NADH) 0.1 mmol l−1. The reaction was started by addition of dihydroxyacetonephosphate at 0.67 mmol l−1. Xylose reductase (XR) and xylitol dehydrogenase (XDH) were assayed as described in Ref. [13], with the exception that the XR activity was assayed in the presence of 0.4 mmol NADH.
One unit (U) of enzyme activity was defined as 1 μmol substrate transformed per minute using an extinction coefficient for NADH of 6.22 l mmol−1 cm−1. Enzyme assays were performed in triplicate, and the obtained standard deviations varied between 3 and 12%.
Results and discussion
Screening for ability to grow on different sugars as carbon source
The ability to use a wide range of carbon sources represents a very interesting feature to develop suitable fermentative strategies for ethanol production from renewable sources. Fifty-four Dekkera/Brettanomyces strains were screened on solid media for their ability to grow on different sugars as carbon source: saccharose, cellobiose, maltose, lactose, starch, xylose, galactose and arabinose (Table 1). Surprisingly, we observed that several D. anomala strains, one strain of B. custersianus and one strain of B. nanus were even able to use agar as sole carbon source. To avoid any “contamination” for testing alternative carbon sources we then developed a plate assay by using silica support. In this way we could observe that all species grew on plates containing hexoses, disaccharides and starch, although with some individual variation due to strain specificity. Only B. naardenensis was able to utilise pentoses, such as d-xylose and d-arabinose. In conclusion, Dekkera/Brettanomyces yeasts can be cultivated on a wide variety of sugars.
Carbon utilisation, as tested on silica plates, of strains of Dekkera/Brettanomyces
CBS strains . | Xylose . | Xylitol . | Ara . | Glu . | Gal . | Man . | Sucr . | Mal . | Cellob . | Starch . | Agar . |
---|---|---|---|---|---|---|---|---|---|---|---|
B. custersianus | |||||||||||
4805 | − | − | − | + | + | + | + | + | + | − | + |
4806 | − | − | − | + | + | + | + | − | + | + | − |
5207, 8347 | − | − | − | + | + | + | + | + | + | − | − |
5208 | − | − | − | + | + | + | + | − | + | − | − |
B. naardenensis | |||||||||||
6040 | + | + | + | + | + | + | + | + | + | + | − |
6108 | − | − | − | + | − | + | + | + | + | + | − |
6115 | + | + | − | + | + | + | + | + | + | + | − |
7540 | + | + | − | + | + | − | + | − | + | + | − |
B. nanus | |||||||||||
1945 | − | − | − | + | + | + | + | + | + | + | − |
1947 | − | − | − | + | + | + | + | + | + | + | + |
D. anomala | |||||||||||
76, 3026 | − | − | − | + | + | + | + | + | + | + | − |
77, 4460, 7250 | − | − | − | + | + | + | + | + | + | − | − |
1938, 4211, 4212, 7654, 8138 | − | − | − | + | + | + | + | + | + | + | + |
4210, 8139 | − | − | − | + | + | + | + | + | + | − | + |
4608 | − | − | − | + | + | + | + | − | + | − | − |
4711 | − | − | − | + | + | − | + | + | + | − | − |
4712 | − | − | − | + | + | + | + | − | + | + | + |
D. bruxellensis | |||||||||||
72, 73, 75, 96, 98, 1940, 1941, 1942, 1943, 2499, 2547, 2796, 3025, 4482, 5206, 5512, 5513 | − | − | − | + | + | + | + | + | + | + | − |
74, 78 | − | − | − | + | + | + | + | + | − | − | − |
2336, 2797, 4459, 6055, 4481, 8027 | − | − | − | + | + | + | + | + | + | − | − |
4602 | − | − | − | + | − | + | + | − | − | + | − |
4601 | − | − | − | + | + | + | + | − | − | − | − |
4914 | − | − | − | + | + | + | + | − | + | + | − |
CBS strains . | Xylose . | Xylitol . | Ara . | Glu . | Gal . | Man . | Sucr . | Mal . | Cellob . | Starch . | Agar . |
---|---|---|---|---|---|---|---|---|---|---|---|
B. custersianus | |||||||||||
4805 | − | − | − | + | + | + | + | + | + | − | + |
4806 | − | − | − | + | + | + | + | − | + | + | − |
5207, 8347 | − | − | − | + | + | + | + | + | + | − | − |
5208 | − | − | − | + | + | + | + | − | + | − | − |
B. naardenensis | |||||||||||
6040 | + | + | + | + | + | + | + | + | + | + | − |
6108 | − | − | − | + | − | + | + | + | + | + | − |
6115 | + | + | − | + | + | + | + | + | + | + | − |
7540 | + | + | − | + | + | − | + | − | + | + | − |
B. nanus | |||||||||||
1945 | − | − | − | + | + | + | + | + | + | + | − |
1947 | − | − | − | + | + | + | + | + | + | + | + |
D. anomala | |||||||||||
76, 3026 | − | − | − | + | + | + | + | + | + | + | − |
77, 4460, 7250 | − | − | − | + | + | + | + | + | + | − | − |
1938, 4211, 4212, 7654, 8138 | − | − | − | + | + | + | + | + | + | + | + |
4210, 8139 | − | − | − | + | + | + | + | + | + | − | + |
4608 | − | − | − | + | + | + | + | − | + | − | − |
4711 | − | − | − | + | + | − | + | + | + | − | − |
4712 | − | − | − | + | + | + | + | − | + | + | + |
D. bruxellensis | |||||||||||
72, 73, 75, 96, 98, 1940, 1941, 1942, 1943, 2499, 2547, 2796, 3025, 4482, 5206, 5512, 5513 | − | − | − | + | + | + | + | + | + | + | − |
74, 78 | − | − | − | + | + | + | + | + | − | − | − |
2336, 2797, 4459, 6055, 4481, 8027 | − | − | − | + | + | + | + | + | + | − | − |
4602 | − | − | − | + | − | + | + | − | − | + | − |
4601 | − | − | − | + | + | + | + | − | − | − | − |
4914 | − | − | − | + | + | + | + | − | + | + | − |
ara arabinose, glu glucose, gal galactose, man mannose, sucr sucrose, mal maltose, cellob cellobiose. Approximately 200 cells were spotted onto the plate: “+” means growth, and “−” means no growth after 6 weeks of incubation
Carbon utilisation, as tested on silica plates, of strains of Dekkera/Brettanomyces
CBS strains . | Xylose . | Xylitol . | Ara . | Glu . | Gal . | Man . | Sucr . | Mal . | Cellob . | Starch . | Agar . |
---|---|---|---|---|---|---|---|---|---|---|---|
B. custersianus | |||||||||||
4805 | − | − | − | + | + | + | + | + | + | − | + |
4806 | − | − | − | + | + | + | + | − | + | + | − |
5207, 8347 | − | − | − | + | + | + | + | + | + | − | − |
5208 | − | − | − | + | + | + | + | − | + | − | − |
B. naardenensis | |||||||||||
6040 | + | + | + | + | + | + | + | + | + | + | − |
6108 | − | − | − | + | − | + | + | + | + | + | − |
6115 | + | + | − | + | + | + | + | + | + | + | − |
7540 | + | + | − | + | + | − | + | − | + | + | − |
B. nanus | |||||||||||
1945 | − | − | − | + | + | + | + | + | + | + | − |
1947 | − | − | − | + | + | + | + | + | + | + | + |
D. anomala | |||||||||||
76, 3026 | − | − | − | + | + | + | + | + | + | + | − |
77, 4460, 7250 | − | − | − | + | + | + | + | + | + | − | − |
1938, 4211, 4212, 7654, 8138 | − | − | − | + | + | + | + | + | + | + | + |
4210, 8139 | − | − | − | + | + | + | + | + | + | − | + |
4608 | − | − | − | + | + | + | + | − | + | − | − |
4711 | − | − | − | + | + | − | + | + | + | − | − |
4712 | − | − | − | + | + | + | + | − | + | + | + |
D. bruxellensis | |||||||||||
72, 73, 75, 96, 98, 1940, 1941, 1942, 1943, 2499, 2547, 2796, 3025, 4482, 5206, 5512, 5513 | − | − | − | + | + | + | + | + | + | + | − |
74, 78 | − | − | − | + | + | + | + | + | − | − | − |
2336, 2797, 4459, 6055, 4481, 8027 | − | − | − | + | + | + | + | + | + | − | − |
4602 | − | − | − | + | − | + | + | − | − | + | − |
4601 | − | − | − | + | + | + | + | − | − | − | − |
4914 | − | − | − | + | + | + | + | − | + | + | − |
CBS strains . | Xylose . | Xylitol . | Ara . | Glu . | Gal . | Man . | Sucr . | Mal . | Cellob . | Starch . | Agar . |
---|---|---|---|---|---|---|---|---|---|---|---|
B. custersianus | |||||||||||
4805 | − | − | − | + | + | + | + | + | + | − | + |
4806 | − | − | − | + | + | + | + | − | + | + | − |
5207, 8347 | − | − | − | + | + | + | + | + | + | − | − |
5208 | − | − | − | + | + | + | + | − | + | − | − |
B. naardenensis | |||||||||||
6040 | + | + | + | + | + | + | + | + | + | + | − |
6108 | − | − | − | + | − | + | + | + | + | + | − |
6115 | + | + | − | + | + | + | + | + | + | + | − |
7540 | + | + | − | + | + | − | + | − | + | + | − |
B. nanus | |||||||||||
1945 | − | − | − | + | + | + | + | + | + | + | − |
1947 | − | − | − | + | + | + | + | + | + | + | + |
D. anomala | |||||||||||
76, 3026 | − | − | − | + | + | + | + | + | + | + | − |
77, 4460, 7250 | − | − | − | + | + | + | + | + | + | − | − |
1938, 4211, 4212, 7654, 8138 | − | − | − | + | + | + | + | + | + | + | + |
4210, 8139 | − | − | − | + | + | + | + | + | + | − | + |
4608 | − | − | − | + | + | + | + | − | + | − | − |
4711 | − | − | − | + | + | − | + | + | + | − | − |
4712 | − | − | − | + | + | + | + | − | + | + | + |
D. bruxellensis | |||||||||||
72, 73, 75, 96, 98, 1940, 1941, 1942, 1943, 2499, 2547, 2796, 3025, 4482, 5206, 5512, 5513 | − | − | − | + | + | + | + | + | + | + | − |
74, 78 | − | − | − | + | + | + | + | + | − | − | − |
2336, 2797, 4459, 6055, 4481, 8027 | − | − | − | + | + | + | + | + | + | − | − |
4602 | − | − | − | + | − | + | + | − | − | + | − |
4601 | − | − | − | + | + | + | + | − | − | − | − |
4914 | − | − | − | + | + | + | + | − | + | + | − |
ara arabinose, glu glucose, gal galactose, man mannose, sucr sucrose, mal maltose, cellob cellobiose. Approximately 200 cells were spotted onto the plate: “+” means growth, and “−” means no growth after 6 weeks of incubation
D. bruxellensis ethanol production under oxygen-limited and low-pH conditions
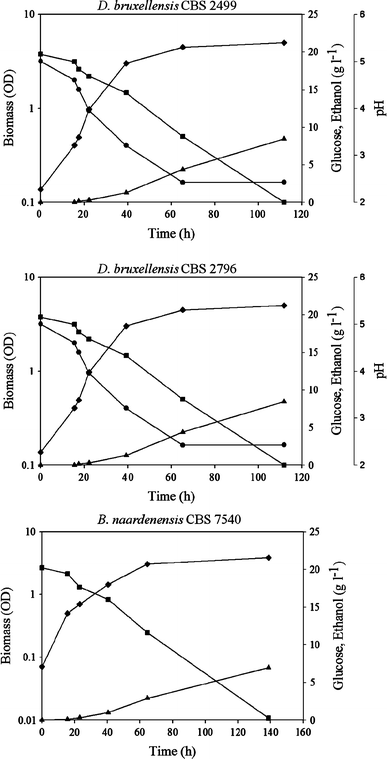
Kinetics of growth, glucose consumption and ethanol production of D. bruxellensis CBS 2499 (a), D. bruxellensis CBS 2796 (b) and B. naardenensis CBS 7540 (c) during batch fermentation under oxygen-limited conditions. Filled diamonds biomass, filled squares glucose, filled triangles ethanol, filled circles pH. Representative experiments are shown
Growth parameters of D. bruxellensis CBS 2499, D. bruxellensis CBS 2796 and B. naardenensis CBS 7540 strains in batch culture under conditions of oxygen limitation
. | D. bruxellensis . | B. naardenensis . | |
---|---|---|---|
CBS 2499 . | CBS 2796 . | CBS 7540 . | |
Specific growth rate (h−1) | 0.083 ± 0.006 | 0.11 ± 0.01 | 0.042 ± 0.005 |
Yields (mg gglucose −1) | |||
Biomass | 73 ± 4 | 80 ± 3 | 89 ± 7 |
Ethanol | 440 ± 30 | 430 ± 25 | 360 ± 30 |
Glycerol | 7.8 ± 0.7 | 5.2 ± 0.6 | 36 ± 3 |
Acetic acid | 1.3 ± 0.2 | 1.4 ± 0.2 | 4.3 ± 0.2 |
Production rates (mmol gcells −1 h−1) | |||
Ethanol | 2.83 ± 0.03 | 5.3 ± 0.3 | 3.07 ± 0.15 |
Glycerol | 0.022 ± 0.002 | 0.01 ± 0.006 | 0.25 ± 0.02 |
Acetic acid | 0.025 ± 0.002 | 0.021 ± 0.001 | 0.047 ± 0.005 |
Glucose consumption rate (mmol gcells −1 h−1) | 2.50 ± 0.18 | 3.99 ± 0.32 | 2.99 ± 0.24 |
. | D. bruxellensis . | B. naardenensis . | |
---|---|---|---|
CBS 2499 . | CBS 2796 . | CBS 7540 . | |
Specific growth rate (h−1) | 0.083 ± 0.006 | 0.11 ± 0.01 | 0.042 ± 0.005 |
Yields (mg gglucose −1) | |||
Biomass | 73 ± 4 | 80 ± 3 | 89 ± 7 |
Ethanol | 440 ± 30 | 430 ± 25 | 360 ± 30 |
Glycerol | 7.8 ± 0.7 | 5.2 ± 0.6 | 36 ± 3 |
Acetic acid | 1.3 ± 0.2 | 1.4 ± 0.2 | 4.3 ± 0.2 |
Production rates (mmol gcells −1 h−1) | |||
Ethanol | 2.83 ± 0.03 | 5.3 ± 0.3 | 3.07 ± 0.15 |
Glycerol | 0.022 ± 0.002 | 0.01 ± 0.006 | 0.25 ± 0.02 |
Acetic acid | 0.025 ± 0.002 | 0.021 ± 0.001 | 0.047 ± 0.005 |
Glucose consumption rate (mmol gcells −1 h−1) | 2.50 ± 0.18 | 3.99 ± 0.32 | 2.99 ± 0.24 |
Growth parameters of D. bruxellensis CBS 2499, D. bruxellensis CBS 2796 and B. naardenensis CBS 7540 strains in batch culture under conditions of oxygen limitation
. | D. bruxellensis . | B. naardenensis . | |
---|---|---|---|
CBS 2499 . | CBS 2796 . | CBS 7540 . | |
Specific growth rate (h−1) | 0.083 ± 0.006 | 0.11 ± 0.01 | 0.042 ± 0.005 |
Yields (mg gglucose −1) | |||
Biomass | 73 ± 4 | 80 ± 3 | 89 ± 7 |
Ethanol | 440 ± 30 | 430 ± 25 | 360 ± 30 |
Glycerol | 7.8 ± 0.7 | 5.2 ± 0.6 | 36 ± 3 |
Acetic acid | 1.3 ± 0.2 | 1.4 ± 0.2 | 4.3 ± 0.2 |
Production rates (mmol gcells −1 h−1) | |||
Ethanol | 2.83 ± 0.03 | 5.3 ± 0.3 | 3.07 ± 0.15 |
Glycerol | 0.022 ± 0.002 | 0.01 ± 0.006 | 0.25 ± 0.02 |
Acetic acid | 0.025 ± 0.002 | 0.021 ± 0.001 | 0.047 ± 0.005 |
Glucose consumption rate (mmol gcells −1 h−1) | 2.50 ± 0.18 | 3.99 ± 0.32 | 2.99 ± 0.24 |
. | D. bruxellensis . | B. naardenensis . | |
---|---|---|---|
CBS 2499 . | CBS 2796 . | CBS 7540 . | |
Specific growth rate (h−1) | 0.083 ± 0.006 | 0.11 ± 0.01 | 0.042 ± 0.005 |
Yields (mg gglucose −1) | |||
Biomass | 73 ± 4 | 80 ± 3 | 89 ± 7 |
Ethanol | 440 ± 30 | 430 ± 25 | 360 ± 30 |
Glycerol | 7.8 ± 0.7 | 5.2 ± 0.6 | 36 ± 3 |
Acetic acid | 1.3 ± 0.2 | 1.4 ± 0.2 | 4.3 ± 0.2 |
Production rates (mmol gcells −1 h−1) | |||
Ethanol | 2.83 ± 0.03 | 5.3 ± 0.3 | 3.07 ± 0.15 |
Glycerol | 0.022 ± 0.002 | 0.01 ± 0.006 | 0.25 ± 0.02 |
Acetic acid | 0.025 ± 0.002 | 0.021 ± 0.001 | 0.047 ± 0.005 |
Glucose consumption rate (mmol gcells −1 h−1) | 2.50 ± 0.18 | 3.99 ± 0.32 | 2.99 ± 0.24 |
B. naardenensis ethanol production under oxygen-limited conditions
To date, neither cultivation under oxygen-limited conditions nor ethanol production by B. naardenensis have been reported in literature. To study the fermentative growth of B. naardenensis, four strains that have previously been tested on plates were cultivated in liquid on glucose and on xylose under aerobic conditions, to determine their specific growth rates. Strain CBS 7540 exhibited the fastest rates (0.3 h−1 on glucose and 0.23 h−1 on xylose) and for this reason was selected to be studied in more detail for its ability to produce ethanol.
Under conditions of oxygen limitation, B. naardenensis was able to grow on synthetic medium containing glucose as carbon source (Fig. 1c, Table 2), but at a lower growth rate than D. bruxellensis, even though their glucose consumption rates were similar. B. naardenensis produced ethanol at a specific production rate similar to that determined in D. bruxellensis cultures, but with lower yield (Table 2). In addition, B. naardenensis showed higher production of glycerol and acetic acid than D. bruxellensis (Table 2).
B. naardenensis failed to grow on xylose under oxygen-limited conditions (data not shown).
Ethanol production from xylose by a two-step batch process
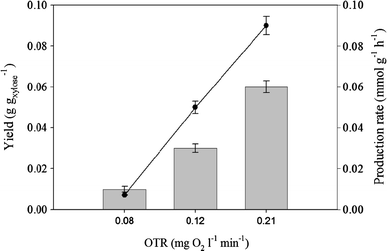
Ethanol production rates and yields in batch fermentations of B. naardenensis CBS 7540 on xylose (20 g l−1) and at different levels of oxygenation (OTR). Grey bars yield, filled circles ethanol production rate
Analysis of enzymatic activities involved in glucose and xylose fermentation
The main enzymatic activities involved in sugar metabolism and in the fermentative pathway were analysed in D. bruxellensis and in B. naardenensis under different growth conditions. We found that biochemical and physiological observations supported each other (Table 3). D. bruxellensis has been reported to produce ethanol under aerobic conditions, thus behaving like a Crabtree-positive yeast [31]. We could detect activities of the main fermentative enzymes, pyruvate decarboxylase (PDC) and alcohol dehydrogenase (ADH), under aerobic conditions as well as under conditions of oxygen limitation, and they were found to be unaffected by the dissolved oxygen concentration, as expected for a Crabtree-positive yeast (Table 3). Acetaldehyde dehydrogenase (ACDH), involved in the production of acetic acid, was detected at a lower level under oxygen-limited conditions, in agreement with the concomitant low production of acetic acid. Undetectable activities of acetyl CoA synthetase (ACS) were found in both aerobic and oxygen-limited conditions. Glycerol 3-phosphate dehydrogenase activity (GPDH), involved in the production of glycerol, was detected only during the growth under oxygen-limited conditions, accordingly to the production of this metabolite.
Activity of D. bruxellensis CBS 2499 and B. naardenensis CBS 7540 enzymes involved in glucose and xylose fermentation
Enzyme . | D. bruxellensis CBS 2499 . | B. naardenensis CBS 7540 . | ||||
---|---|---|---|---|---|---|
Glucose . | Glucose . | Xylose . | ||||
+O2 . | O2 lim . | +O2 . | O2 lim . | +O2 . | O2 lim . | |
HXK | 0.58 ± 0.01 | 0.79 ± 0.05 | 0.37 ± 0.03 | 1.71 ± 0.04 | – | – |
G6PDH | 0.21 ± 0.09 | 0.32 ± 0.04 | 0.65 ± 0.01 | 1.35 ± 0.005 | 0.60 ± 0.04 | 0.45 ± 0.04 |
PDC | 0.26 ± 0.01 | 0.22 ± 0.06 | 0.02 ± 0.005 | 1.19 ± 0.02 | 0.10 ± 0.0025 | 0.29 ± 0.01 |
ADH | 4,19 ± 0.11 | 4.40 ± 0.52 | 0.05 ± 0.007 | 1.72 ± 0.03 | 0.31 ± 0.01 | 1.45 ± 0.04 |
ACDH | 0.31 ± 0.02 | 0.06 ± 0.003 | 0.003 ± 0.001 | ND | 0.05 ± 0.002 | 0.04 ± 0.004 |
ACS | ND | ND | 0.133 ± 0.04 | 0.031 ± 0.004 | – | – |
GPD | ND | 0.034 ± 0.004 | ND | ND | ND | ND |
XR-NADH | – | – | ND | ND | ND | ND |
XR-NADPH | – | – | 0.018 ± 0.004 | 0.011 ± 0.006 | 0.07 ± 0.003 | 0.113 ± 0.003 |
XDH | – | – | ND | ND | 0.23 ± 0.01 | 0.26 ± 0.03 |
Enzyme . | D. bruxellensis CBS 2499 . | B. naardenensis CBS 7540 . | ||||
---|---|---|---|---|---|---|
Glucose . | Glucose . | Xylose . | ||||
+O2 . | O2 lim . | +O2 . | O2 lim . | +O2 . | O2 lim . | |
HXK | 0.58 ± 0.01 | 0.79 ± 0.05 | 0.37 ± 0.03 | 1.71 ± 0.04 | – | – |
G6PDH | 0.21 ± 0.09 | 0.32 ± 0.04 | 0.65 ± 0.01 | 1.35 ± 0.005 | 0.60 ± 0.04 | 0.45 ± 0.04 |
PDC | 0.26 ± 0.01 | 0.22 ± 0.06 | 0.02 ± 0.005 | 1.19 ± 0.02 | 0.10 ± 0.0025 | 0.29 ± 0.01 |
ADH | 4,19 ± 0.11 | 4.40 ± 0.52 | 0.05 ± 0.007 | 1.72 ± 0.03 | 0.31 ± 0.01 | 1.45 ± 0.04 |
ACDH | 0.31 ± 0.02 | 0.06 ± 0.003 | 0.003 ± 0.001 | ND | 0.05 ± 0.002 | 0.04 ± 0.004 |
ACS | ND | ND | 0.133 ± 0.04 | 0.031 ± 0.004 | – | – |
GPD | ND | 0.034 ± 0.004 | ND | ND | ND | ND |
XR-NADH | – | – | ND | ND | ND | ND |
XR-NADPH | – | – | 0.018 ± 0.004 | 0.011 ± 0.006 | 0.07 ± 0.003 | 0.113 ± 0.003 |
XDH | – | – | ND | ND | 0.23 ± 0.01 | 0.26 ± 0.03 |
Cell extracts were taken during the exponential phase of growth under aerobic (+O2) and under oxygen-limited (O2 lim) conditions
ADH alcohol dehydrogenase, ACDH acetaldehyde dehydrogenase, ACS acetyl CoA synthetase, G6PDH glucose 6-phosphate dehydrogenase, GPD glycerol 3-phosphate dehydrogenase, HXK hexokinase, PDC pyruvate decarboxylase, XR-NADH xylose reductase NADH-dependent, XR-NADPH xylose reductase NADPH-dependent, XDH xylitol dehydrogenase, ND not detectable, “–” not measured
Activity of D. bruxellensis CBS 2499 and B. naardenensis CBS 7540 enzymes involved in glucose and xylose fermentation
Enzyme . | D. bruxellensis CBS 2499 . | B. naardenensis CBS 7540 . | ||||
---|---|---|---|---|---|---|
Glucose . | Glucose . | Xylose . | ||||
+O2 . | O2 lim . | +O2 . | O2 lim . | +O2 . | O2 lim . | |
HXK | 0.58 ± 0.01 | 0.79 ± 0.05 | 0.37 ± 0.03 | 1.71 ± 0.04 | – | – |
G6PDH | 0.21 ± 0.09 | 0.32 ± 0.04 | 0.65 ± 0.01 | 1.35 ± 0.005 | 0.60 ± 0.04 | 0.45 ± 0.04 |
PDC | 0.26 ± 0.01 | 0.22 ± 0.06 | 0.02 ± 0.005 | 1.19 ± 0.02 | 0.10 ± 0.0025 | 0.29 ± 0.01 |
ADH | 4,19 ± 0.11 | 4.40 ± 0.52 | 0.05 ± 0.007 | 1.72 ± 0.03 | 0.31 ± 0.01 | 1.45 ± 0.04 |
ACDH | 0.31 ± 0.02 | 0.06 ± 0.003 | 0.003 ± 0.001 | ND | 0.05 ± 0.002 | 0.04 ± 0.004 |
ACS | ND | ND | 0.133 ± 0.04 | 0.031 ± 0.004 | – | – |
GPD | ND | 0.034 ± 0.004 | ND | ND | ND | ND |
XR-NADH | – | – | ND | ND | ND | ND |
XR-NADPH | – | – | 0.018 ± 0.004 | 0.011 ± 0.006 | 0.07 ± 0.003 | 0.113 ± 0.003 |
XDH | – | – | ND | ND | 0.23 ± 0.01 | 0.26 ± 0.03 |
Enzyme . | D. bruxellensis CBS 2499 . | B. naardenensis CBS 7540 . | ||||
---|---|---|---|---|---|---|
Glucose . | Glucose . | Xylose . | ||||
+O2 . | O2 lim . | +O2 . | O2 lim . | +O2 . | O2 lim . | |
HXK | 0.58 ± 0.01 | 0.79 ± 0.05 | 0.37 ± 0.03 | 1.71 ± 0.04 | – | – |
G6PDH | 0.21 ± 0.09 | 0.32 ± 0.04 | 0.65 ± 0.01 | 1.35 ± 0.005 | 0.60 ± 0.04 | 0.45 ± 0.04 |
PDC | 0.26 ± 0.01 | 0.22 ± 0.06 | 0.02 ± 0.005 | 1.19 ± 0.02 | 0.10 ± 0.0025 | 0.29 ± 0.01 |
ADH | 4,19 ± 0.11 | 4.40 ± 0.52 | 0.05 ± 0.007 | 1.72 ± 0.03 | 0.31 ± 0.01 | 1.45 ± 0.04 |
ACDH | 0.31 ± 0.02 | 0.06 ± 0.003 | 0.003 ± 0.001 | ND | 0.05 ± 0.002 | 0.04 ± 0.004 |
ACS | ND | ND | 0.133 ± 0.04 | 0.031 ± 0.004 | – | – |
GPD | ND | 0.034 ± 0.004 | ND | ND | ND | ND |
XR-NADH | – | – | ND | ND | ND | ND |
XR-NADPH | – | – | 0.018 ± 0.004 | 0.011 ± 0.006 | 0.07 ± 0.003 | 0.113 ± 0.003 |
XDH | – | – | ND | ND | 0.23 ± 0.01 | 0.26 ± 0.03 |
Cell extracts were taken during the exponential phase of growth under aerobic (+O2) and under oxygen-limited (O2 lim) conditions
ADH alcohol dehydrogenase, ACDH acetaldehyde dehydrogenase, ACS acetyl CoA synthetase, G6PDH glucose 6-phosphate dehydrogenase, GPD glycerol 3-phosphate dehydrogenase, HXK hexokinase, PDC pyruvate decarboxylase, XR-NADH xylose reductase NADH-dependent, XR-NADPH xylose reductase NADPH-dependent, XDH xylitol dehydrogenase, ND not detectable, “–” not measured
In B. naardenensis, on the contrary, PDC and ADH were strongly induced at reduced oxygen supply (Table 3), as reported for Crabtree-negative yeasts [35]. Very low activity of ACDH was detected under aerobic conditions on glucose, but higher levels were observed on xylose. This could affect the redox balance during xylose fermentation (see paragraph below), due to the presence of NAD-dependent acetaldehyde dehydrogenase activity [13, 29]. GPDH was undetectable even under conditions of oxygen limitation. Interestingly, the detected activity of glucose 6-phosphate dehydrogenase (G6PDH) in B. naardenensis was higher than in D. bruxellensis, as well as the activity of ACS.
Xylose utilisation in B. naardenensis was found to be dependent on the activity of a specific nicotinamide adenine dinucleotide phosphate (NADPH)-dependent xylose reductase (XR) activity and on the activity of a NAD-dependent xylitol dehydrogenase (XDH) (Table 3). We observed an 8–10-fold lower level of XR and a twofold lower level of XDH in comparison with those reported for P. stipitis [36]. Furthermore, XR was found to be specific only for NADPH (Table 3), thereby not participating in re-oxidation of NADH produced by XDH. This could cause a cofactor imbalance during xylose fermentation, resulting in xylitol accumulation.
Fermentation of synthetic lignocellulosic hydrolysates
The composition of the sugar fraction in lignocellulosic hydrolysates is dependent on the raw material [9]. We first carried out a fermentation process by using a mixture of glucose and xylose with the typical 1:4 ratio obtained in hardwood hydrolysates. Also in this case we used the two-step process described above, with the first phase for fast biomass production in batch mode and under controlled aerobic conditions on the glucose/xylose mixture. When the final biomass was reached and both sugars consumed, the conditions of oxygen limitation were applied (OTR 0.12 mgoxygen l−1 min−1) to induce ethanol production. In this process we tested also the possibility of feeding the biomass during the ethanol production phase by using the same mixture containing glucose and xylose at 1:4 ratio employed for the biomass production phase (for details see “Materials and methods” section). Also in this case no further increase of biomass was observed in the second phase under oxygen-limited conditions, as expected. We analysed processes at different feeding rates (Table 4). Due to the presence of glucose in the feed, a higher ethanol yield than that observed in the process on xylose only (see paragraph above and Fig. 2 for comparison) was obtained. In addition, by increasing the feeding rate, higher ethanol yield and productivity were obtained (Table 4), but this resulted in xylitol accumulation (data not shown). This fact could indicate that xylose fermentation under oxygen-limited conditions causes a redox imbalance, probably due to the different cofactor utilisation by the two enzymes (XR and XDH) involved in the first steps of xylose metabolism (Table 3). Recombinant pentose-fermenting S. cerevisiae strains often experience such a redox imbalance, leading to undesired xylitol accumulation [16, 36]. Several strategies to reduce xylitol formation have been explored, and one of the most successful has been the expression of xylose isomerase from Piromyces sp., which converts xylose to xylulose without any cofactor involvement [19].
Ethanol yield and productivity of B. naardenensis CBS 7540 in batch fermentations fed by mixtures of glucose and xylose (1:4) at different feeding flow rates
Feeding flow . | Ethanol yield . | Ethanol production rate . | |
---|---|---|---|
Xylose . | Glucose . | ||
gxylose gcells −1 h−1 . | gglucose gcells −1 h−1 . | g gsugars −1 . | mmolsugars gcells −1 h−1 . |
0.04 | 0.01 | 0.20 ± 0.01 | 0.13 ± 0.01 |
0.16 | 0.04 | 0.25 ± 0.02 | 0.38 ± 0.02 |
Feeding flow . | Ethanol yield . | Ethanol production rate . | |
---|---|---|---|
Xylose . | Glucose . | ||
gxylose gcells −1 h−1 . | gglucose gcells −1 h−1 . | g gsugars −1 . | mmolsugars gcells −1 h−1 . |
0.04 | 0.01 | 0.20 ± 0.01 | 0.13 ± 0.01 |
0.16 | 0.04 | 0.25 ± 0.02 | 0.38 ± 0.02 |
Ethanol yield and productivity of B. naardenensis CBS 7540 in batch fermentations fed by mixtures of glucose and xylose (1:4) at different feeding flow rates
Feeding flow . | Ethanol yield . | Ethanol production rate . | |
---|---|---|---|
Xylose . | Glucose . | ||
gxylose gcells −1 h−1 . | gglucose gcells −1 h−1 . | g gsugars −1 . | mmolsugars gcells −1 h−1 . |
0.04 | 0.01 | 0.20 ± 0.01 | 0.13 ± 0.01 |
0.16 | 0.04 | 0.25 ± 0.02 | 0.38 ± 0.02 |
Feeding flow . | Ethanol yield . | Ethanol production rate . | |
---|---|---|---|
Xylose . | Glucose . | ||
gxylose gcells −1 h−1 . | gglucose gcells −1 h−1 . | g gsugars −1 . | mmolsugars gcells −1 h−1 . |
0.04 | 0.01 | 0.20 ± 0.01 | 0.13 ± 0.01 |
0.16 | 0.04 | 0.25 ± 0.02 | 0.38 ± 0.02 |
Softwood hydrolysates yield a high proportion of hexose sugars, mainly mannose and glucose. To approach the real conditions occurring when this kind of hydrolysate is utilised, the fermentation process was performed by using a mixture containing hexoses and pentoses at the same concentrations as in undetoxified spruce hydrolysate [15]. Again, we ran the process in batch mode in two steps: the first step, for fast biomass production, on the sugars mixture under controlled aerobic conditions; and the second step, for ethanol production, under oxygen-limited conditions (OTR 0.12 mgoxygen l−1 min−1). In this experiment no feeding was applied during the second step. Table 5 summarises the calculated fermentation parameters. All hexoses and xylose, and half of the initial amount of arabinose, were consumed at the end of the process, leading to production of 13 g l−1 of ethanol, which represented the highest yield and productivity obtained. Also in this case production of glycerol, acetic acid and xylitol occurred. Undetoxified hydrolysates are known to contain inhibitory compounds such as furfurals. We therefore investigated the resistance of B. naardenensis to this compound. We observed that, in the presence of furfural (0.8 g l−1), the growth rate of B. naardenensis strain CBS 7540 showed a 40% reduction (data not shown). Addition of furfural to the synthetic lignocellulosic hydrolysate affected also the fermentation process by decreasing the specific rate of ethanol production, but the final ethanol yield remained almost the same (Table 5).
Ethanol yield and productivity during B. naardenensis CBS 7540 fermentation of a synthetic softwood hydrolysate-like medium (with or without addition of furfural, 1.4 g l−1)
Strain and medium . | Ethanol . | Yield . | ||||
---|---|---|---|---|---|---|
Specific productivity (mmol gcells −1 h−1) . | Volumetric productivity (g l−1 h−1) . | Ethanol (g gsugars −1) . | Acetate (g gsugars −1) . | Glycerol (g gsugars −1) . | Xylitol (g gpentoses −1) . | |
B. naardenensis CBS 7540 | ||||||
Softwood hydrolysate | 0.73 ± 0.09 | 0.135 ± 0.016 | 0.31 ± 0.02 | 0.063 ± 0.004 | 0.034 ± 0.002 | 0.42 ± 0.03 |
Softwood hydrolysate with furfural | 0.42 ± 0.04 | 0.10 ± 0.015 | 0.28 ± 0.02 | 0.086 ± 0.006 | 0.033 ± 0.003 | 0.43 ± 0.02 |
S. cerevisiae TMB 3400a | ||||||
Softwood hydrolysate with furfural | 0.43 ± 0.26 | – | 0.41 ± 0.02 | – | 0.035 ± 0.006 | 0 |
Strain and medium . | Ethanol . | Yield . | ||||
---|---|---|---|---|---|---|
Specific productivity (mmol gcells −1 h−1) . | Volumetric productivity (g l−1 h−1) . | Ethanol (g gsugars −1) . | Acetate (g gsugars −1) . | Glycerol (g gsugars −1) . | Xylitol (g gpentoses −1) . | |
B. naardenensis CBS 7540 | ||||||
Softwood hydrolysate | 0.73 ± 0.09 | 0.135 ± 0.016 | 0.31 ± 0.02 | 0.063 ± 0.004 | 0.034 ± 0.002 | 0.42 ± 0.03 |
Softwood hydrolysate with furfural | 0.42 ± 0.04 | 0.10 ± 0.015 | 0.28 ± 0.02 | 0.086 ± 0.006 | 0.033 ± 0.003 | 0.43 ± 0.02 |
S. cerevisiae TMB 3400a | ||||||
Softwood hydrolysate with furfural | 0.43 ± 0.26 | – | 0.41 ± 0.02 | – | 0.035 ± 0.006 | 0 |
S. cerevisiae data are from literature [15]
ND not detectable, “–” not measured
Ethanol yield and productivity during B. naardenensis CBS 7540 fermentation of a synthetic softwood hydrolysate-like medium (with or without addition of furfural, 1.4 g l−1)
Strain and medium . | Ethanol . | Yield . | ||||
---|---|---|---|---|---|---|
Specific productivity (mmol gcells −1 h−1) . | Volumetric productivity (g l−1 h−1) . | Ethanol (g gsugars −1) . | Acetate (g gsugars −1) . | Glycerol (g gsugars −1) . | Xylitol (g gpentoses −1) . | |
B. naardenensis CBS 7540 | ||||||
Softwood hydrolysate | 0.73 ± 0.09 | 0.135 ± 0.016 | 0.31 ± 0.02 | 0.063 ± 0.004 | 0.034 ± 0.002 | 0.42 ± 0.03 |
Softwood hydrolysate with furfural | 0.42 ± 0.04 | 0.10 ± 0.015 | 0.28 ± 0.02 | 0.086 ± 0.006 | 0.033 ± 0.003 | 0.43 ± 0.02 |
S. cerevisiae TMB 3400a | ||||||
Softwood hydrolysate with furfural | 0.43 ± 0.26 | – | 0.41 ± 0.02 | – | 0.035 ± 0.006 | 0 |
Strain and medium . | Ethanol . | Yield . | ||||
---|---|---|---|---|---|---|
Specific productivity (mmol gcells −1 h−1) . | Volumetric productivity (g l−1 h−1) . | Ethanol (g gsugars −1) . | Acetate (g gsugars −1) . | Glycerol (g gsugars −1) . | Xylitol (g gpentoses −1) . | |
B. naardenensis CBS 7540 | ||||||
Softwood hydrolysate | 0.73 ± 0.09 | 0.135 ± 0.016 | 0.31 ± 0.02 | 0.063 ± 0.004 | 0.034 ± 0.002 | 0.42 ± 0.03 |
Softwood hydrolysate with furfural | 0.42 ± 0.04 | 0.10 ± 0.015 | 0.28 ± 0.02 | 0.086 ± 0.006 | 0.033 ± 0.003 | 0.43 ± 0.02 |
S. cerevisiae TMB 3400a | ||||||
Softwood hydrolysate with furfural | 0.43 ± 0.26 | – | 0.41 ± 0.02 | – | 0.035 ± 0.006 | 0 |
S. cerevisiae data are from literature [15]
ND not detectable, “–” not measured
Conclusions
In this work we demonstrated that Dekkera/Brettanomyces yeasts are able to use a wide range of carbon sources, even pentoses. Under conditions suitable for industrial processes, such as under oxygen limitation and low-pH conditions, D. bruxellensis produced ethanol with yields comparable to those of S. cerevisiae (0.44 g g−1 glucose). If we compare the ethanol productivities, it is clear that D. bruxellensis cannot compete with S. cerevisiae in batch processes, but it can in continuous fermentation systems. Notably, D. bruxellensis has been found as a major contaminant in industrial continuous fermentation processes. In addition, for the first time we show that B. naardenensis is able to produce ethanol from glucose and xylose under oxygen-limited conditions. A batch process in two steps was exploited to obtain ethanol from synthetic lignocellulosic hydrolysates. Comparison between B. naardenensis and a metabolically engineered S. cerevisiae strain (Table 5) shows that B. naardenensis exhibits lower ethanol yield but similar ethanol specific productivity. Therefore, our studies demonstrate that Brettanomyces/Dekkera yeasts have clear potential for further development for industrial production of ethanol from renewable sources.
Use of optimised fermentation strategies as well as understanding of the metabolic control and development of genetically modified strains could further improve the basic abilities of Dekkera/Brettanomyces yeasts and subsequently promote their utilisation as new industrial species. A partial genome sequence of Dekkera bruxellensis is now available [10, 37], opening the possibility for genetic manipulation of this yeast. Also some other approaches, such as evolutionary engineering, could be used to increase the ethanol yield.
Acknowledgments
This work was partially funded by INTAS (ref. no. 05-1000005-7730) to C.C. and J.P. J.P. and L.H. thank the Futura and Tryggers Foundation for their funding support.