-
PDF
- Split View
-
Views
-
Cite
Cite
Elcio Ribeiro Borges, Nei Pereira, Succinic acid production from sugarcane bagasse hemicellulose hydrolysate by Actinobacillus succinogenes, Journal of Industrial Microbiology and Biotechnology, Volume 38, Issue 8, 1 August 2011, Pages 1001–1011, https://doi.org/10.1007/s10295-010-0874-7
- Share Icon Share
Abstract
Succinic acid, a four-carbon diacid, has been the focus of many research projects aimed at developing more economically viable methods of fermenting sugar-containing natural materials. Succinic acid fermentation processes also consume CO2, thereby potentially contributing to reductions in CO2 emissions. Succinic acid could also become a commodity used as an intermediate in the chemical synthesis and manufacture of synthetic resins and biodegradable polymers. Much attention has been given recently to the use of microorganisms to produce succinic acid as an alternative to chemical synthesis. We have attempted to maximize succinic acid production by Actinobacillus succinogenes using an experimental design methodology for optimizing the concentrations of the medium components. The first experiment consisted of a 24−1 fractional factorial design, and the second entailed a Central Composite Rotational Design so as to achieve optimal conditions. The optimal concentrations of nutrients predicted by the model were: NaHCO3, 10.0 g l−1; MgSO4, 3.0 g l−1; yeast extract, 2.0 g l−1; KH2PO4. 5.0 g l−1; these were experimentally validated. Under the best conversion conditions, as determined by statistical analysis, the production of succinic acid was carried out in an instrumented bioreactor using sugarcane bagasse hemicellulose hydrolysate, yielding a concentration of 22.5 g l−1.
This article is based on a presentation at the 32nd Symposium on Biotechnology for Fuels and Chemicals.
Electronic supplementary material
The online version of this article (doi:10.1007/s10295-010-0874-7) contains supplementary material, which is available to authorized users.
Introduction
Succinic acid is currently produced from crude oil by catalytic hydrogenation of maleic anhydride to succinic anhydride and subsequent hydration or by the direct catalytic hydrogenation of maleic acid [29]. Population growth and the associated demand for energy and goods coupled with more restrictive environmental regulations and growing concern about carbon emissions from the burning of fossil fuels have intensified the search for renewable energy feedstocks to substitute or complement fossil fuel sources [21, 23]. Sugarcane bagasse, a lignocellulosic material composed of 50% cellulose, 25% hemicellulose and 25% lignin, is one of the most important agricultural residues produced in Brazil, amounting to approximately 250 kg per ton sugarcane [28]. A diluted acid pretreatment of sugarcane bagasse generates a liquid phase (hemicellulose hydrolysate) composed mainly of xylose, which can, in turn, be used as a substrate for biotechnological and chemical processes [7, 11]. The use of a renewable agricultural feedstock for the production of succinic acid reduces the need to draw upon limited oil reserves, thus diversifying a biorefinery’s potential product portfolio [17]. The utilization of such feedstocks within the concept of biorefinery has been successfully investigated for the fermentative production of a range of products, including lactic acid, ethanol, polyhydroxybutyrate (PHB) and succinic acid [6].
Succinic acid, also known as amber acid or butanedioic acid, is a dicarboxylic acid with the molecular formula C4H6O4. It can be used as a precursor for the production of many chemicals for use in the agricultural, food processing and pharmaceutical industries [for example, as surfactants, detergents, adipic acid, 1,4 butanediol, tetrahydrofuran, N-methyl pyrrolidinone, 2-pyrrolidinone, succinate salts, gamma-butyrolactone, various green solvents, biodegradable polymers, such as polybutyrate succinate (PBS) and ingredients to stimulate animal and plant growth] [22, 29]. Several research teams are working on the development of industrial-level fermentation processes for succinic acid production using strains of Anaerobiospirillum succiniciproducens, Actinobacillus succinogenes, Mannheimia succiniciproducens [14, 24, 30] and Corynebacterium glutamicum and recombinant Escherichia coli strains [3, 19]. A. succinogenes is able to produce a relatively large amount of succinic acid from a broad range of carbon sources, such as arabinose, cellobiose, fructose, galactose, glucose, lactose, maltose, mannitol, mannose, sorbitol, sucrose, xylose or salicin under anaerobic conditions [8]. This microbial species is a facultatively anaerobic, Gram-negative bacterium that naturally produces high concentrations of succinate as a fermentation end product in addition to formate, acetate and ethanol [8, 26]. CO2 functions as an electron acceptor and alters the flux of phosphoenolpyruvate (PEP), which induces the metabolism of the substrate to pyruvate and lactate/ethanol at low CO2 levels and succinate at high CO2 levels [26].
The composition of culture medium significantly affects the yield of any fermentation product. Physiological and nutritional factors, such as the initial sugar concentration, complex nitrogen sources, inoculum size and cell acclimatization, carbonate ion concentrations, magnesium, pH and the temperature of the growth medium, are reported to be the most critical factors affecting both cell growth and succinic acid production [1, 14].
Objective
The ultimate aim of the study reported here was to develop a cheap and suitable medium for succinic acid production from the hemicellulose hydrolysate of sugarcane bagasse in a batchwise culture of A. succinogenes. To this end, we studied the influence of the medium components (NaHCO3, Yeast Extract, K2HPO4, MgSO4) and their interaction on succinic acid production using an experimental design strategy.
Materials and methods
Microorganism and inoculum preparation
The strain of A. succinogenes CIP 106512 was obtained from the Pasteur Institute. The culture stock was maintained on Trypticase Soy Agar (TSA) slants at 4°C. The inoculum was grown in a liquid medium composed of xylose, 20 g l−1; yeast extract, 2.0 g l−1; MgSO4, 1.0 g l−1; NaHCO3, 12.0 g l−1; K2HPO4, 1.5 g l−1. The cultures were shaken at 150 rpm and 37°C in an orbital shaking incubator for 24 h, which was the time required for the microorganisms to enter the exponential growth phase. After growth, the cells needed for each fermentation assay were separated from the media by centrifugation at 8,000 rpm and 10°C for 20 min.
Fermentation assays in flasks

Schematic representation of CO2 distribution in shake flasks for medium optimization in succinic acid fermentation
The results were analyzed by experimental design to define the optimal conditions for succinic acid production, and the predicted values were validated experimentally in batch fermentation.
Fermentation assays in bioreactor using synthetic medium
All batch fermentations were carried out at 37°C in a 2-l bioreactor (BIOFLO III; New Brunswick Scientific, Edison, NJ) with a working volume of 1.5 l. The initial xylose concentration in the medium was 20 g l−1, and the temperature and agitation speed were controlled at 37°C and 150 rpm, respectively. The bioreactor was continuously supplied with CO2 from the gas cylinder at a flow rate of 0.05 vvm during the whole fermentation period. A second bioreactor was operated without a supply of external CO2 to compare how essential the feed of this gas was in the succinic acid production. The pH was monitored using a sterilizable pH electrode and maintained at 7.0 through the addition of 1 M NaOH. The kinetics of the fermentation process were evaluated under the optimal conditions established in the shake flask experiments. In the second part of this study, the fermentation was performed using the hydrolysate obtained from sugarcane bagasse with a high xylose concentration (52 g l−1).
Sugarcane diluted acid pretreatment/hemicellulose hydrolysis
The sugarcane bagasse (Saccharum spp.) was provided by Costa Pinto Distillery (SP, Brazil). An acid pretreatment was developed to disrupt the lignocellulosic matrix of the sugarcane bagasse and hydrolyze the hemicellulosic component [H2SO4, 1% (v/v); solid:liquid ratio, 1:2; 121°C; 40-min pretreatment] [4]. The hydrolysate obtained was separated from the solid phase by pressure filtration and neutralized until pH 6.0 with Ca(OH)2; it was then filtered again to remove the generated precipitate.
Fermentation assays in bioreactor using sugarcane hemicellulose hydrolysate
The cells were initially activated in the synthetic medium described above and then cultured in two acclimatization stages on a hydrolysate-containing medium; first, with 25% (v/v) medium and second, with 50% (v/v) medium. The cultures were grown in a rotatory shaker at 37°C and 150 rpm. After acclimatization, the cells required for each fermentation assay were separated from the media by centrifugation at 8000 rpm and 10°C for 20 min and inoculated into the bioreactor with all of the same nutrients used in the inoculum preparation medium, with the exception of xylose, which was substituted by the hemicellulose hydrolysate (xylose concentration of 52 g l−1) derived from sugarcane bagasse pretreatment. The kinetics of the fermentation process operating in the bioreactor were evaluated under the optimal conditions established in the shake flask experiments using the statistical software package Design-Expert ver. 6.0 (Stat-Easem Minneapolis, MN).
Optimization of conditions for succinic acid production
The data collected on succinic acid production were subjected to analysis of variance (ANOVA) in order to evaluate statistical significance. The mathematical relationship between the independent and response variables was calculated. The strategy of sequential experimental design was adopted for optimization of succinic acid production. The first experimental 24−1 fractional factorial design with four variables (NaHCO3, yeast extract, K2HPO4, MgSO4) was carried out on two levels, namely, minimum and maximum, coded as “−1” e “+1”, respectively, with two replicates of the center point (Table 1). A second experimental central composite rotational design (CCRD) (Table 2) was conducted to study the influence of the medium components on succinic acid production.
Parameters and levels used in the experimental 24−1 fractional factorial design
Parameters . | Minimum value . | Maximum value . | |
---|---|---|---|
Code . | Component . | −1 . | +1 . |
A | K2HPO4 (g l−1) | 0.0 | 10.0 |
B | MgSO4 (g l−1) | 0.0 | 2.0 |
C | Yeast extract (g l−1) | 0.0 | 5.0 |
D | NaHCO3 (g l−1) | 0.0 | 10.0 |
Parameters . | Minimum value . | Maximum value . | |
---|---|---|---|
Code . | Component . | −1 . | +1 . |
A | K2HPO4 (g l−1) | 0.0 | 10.0 |
B | MgSO4 (g l−1) | 0.0 | 2.0 |
C | Yeast extract (g l−1) | 0.0 | 5.0 |
D | NaHCO3 (g l−1) | 0.0 | 10.0 |
Parameters and levels used in the experimental 24−1 fractional factorial design
Parameters . | Minimum value . | Maximum value . | |
---|---|---|---|
Code . | Component . | −1 . | +1 . |
A | K2HPO4 (g l−1) | 0.0 | 10.0 |
B | MgSO4 (g l−1) | 0.0 | 2.0 |
C | Yeast extract (g l−1) | 0.0 | 5.0 |
D | NaHCO3 (g l−1) | 0.0 | 10.0 |
Parameters . | Minimum value . | Maximum value . | |
---|---|---|---|
Code . | Component . | −1 . | +1 . |
A | K2HPO4 (g l−1) | 0.0 | 10.0 |
B | MgSO4 (g l−1) | 0.0 | 2.0 |
C | Yeast extract (g l−1) | 0.0 | 5.0 |
D | NaHCO3 (g l−1) | 0.0 | 10.0 |
Parameters and levels used in the experimental central composite rotational design (CCRD) for optimization of succinic acid production
Parameters . | Real levels . | |||||
---|---|---|---|---|---|---|
Axial . | Minimum value . | Center point . | Maximum value . | Axial . | ||
Code . | Component . | −α . | − . | 0 . | + . | +α . |
A | K2HPO4 (g l−1) | 2.5 | 5.0 | 7.0 | 10.0 | 12.5 |
B | MgSO4 (g l−1) | 1.0 | 2.0 | 3.0 | 4.0 | 5.0 |
C | Yeast extract (g l−1) | 0.0 | 1.0 | 3.0 | 5.0 | 7.0 |
D | NaHCO3 (g l−1) | 1.5 | 5.0 | 10.0 | 15.0 | 20.0 |
Parameters . | Real levels . | |||||
---|---|---|---|---|---|---|
Axial . | Minimum value . | Center point . | Maximum value . | Axial . | ||
Code . | Component . | −α . | − . | 0 . | + . | +α . |
A | K2HPO4 (g l−1) | 2.5 | 5.0 | 7.0 | 10.0 | 12.5 |
B | MgSO4 (g l−1) | 1.0 | 2.0 | 3.0 | 4.0 | 5.0 |
C | Yeast extract (g l−1) | 0.0 | 1.0 | 3.0 | 5.0 | 7.0 |
D | NaHCO3 (g l−1) | 1.5 | 5.0 | 10.0 | 15.0 | 20.0 |
Parameters and levels used in the experimental central composite rotational design (CCRD) for optimization of succinic acid production
Parameters . | Real levels . | |||||
---|---|---|---|---|---|---|
Axial . | Minimum value . | Center point . | Maximum value . | Axial . | ||
Code . | Component . | −α . | − . | 0 . | + . | +α . |
A | K2HPO4 (g l−1) | 2.5 | 5.0 | 7.0 | 10.0 | 12.5 |
B | MgSO4 (g l−1) | 1.0 | 2.0 | 3.0 | 4.0 | 5.0 |
C | Yeast extract (g l−1) | 0.0 | 1.0 | 3.0 | 5.0 | 7.0 |
D | NaHCO3 (g l−1) | 1.5 | 5.0 | 10.0 | 15.0 | 20.0 |
Parameters . | Real levels . | |||||
---|---|---|---|---|---|---|
Axial . | Minimum value . | Center point . | Maximum value . | Axial . | ||
Code . | Component . | −α . | − . | 0 . | + . | +α . |
A | K2HPO4 (g l−1) | 2.5 | 5.0 | 7.0 | 10.0 | 12.5 |
B | MgSO4 (g l−1) | 1.0 | 2.0 | 3.0 | 4.0 | 5.0 |
C | Yeast extract (g l−1) | 0.0 | 1.0 | 3.0 | 5.0 | 7.0 |
D | NaHCO3 (g l−1) | 1.5 | 5.0 | 10.0 | 15.0 | 20.0 |
The response surface methodology (RSM) and the Pareto chart were also used to investigate the aggregate effect of multiple variables and to seek the optimal conditions for this multivariable system [5].
Analytical methods
Cell concentration was determined by measuring the optical density of a diluted sample at 600 nm (Spectrum Lab 22 PC; S) using a standard curve of absorbance against dry cell mass [16, 30]. Xylose concentration in the samples was determined by high-performance liquid chromatography (HPLC) using the Waters chromatographic system (Waters, Milford, MA) consisting of a HPX-87p column (Bio-Rad, Hercules, CA), a Waters 510 pump, a refractive index detector (Waters model 410) and HP 3390A integrator. The succinic acid concentration produced also was estimated on high performance liquid chromatography (HPLC). Samples (20 μl) were injected into a column (C18, 250 length × 4.6 mm internal diameter, 9 μm; StrodsII Peek) at a flow rate of 0.9 ml min−1. Degassed and filtered sulfuric acid (0.01 N) was used as the mobile phase [8]. Succinic acid yield was defined as the amount of succinic acid produced from 1 g of xylose (expressed as a percentage).
Results and discussion
Fractional factorial design and analysis
The results of the experimental 24−1 fractional factorial design with four variables are presented in Table 3. A set of ten trials was generated (8 independent and 3 repetitions of the central point). Statistical significance of the respective model equations was checked using ANOVA. The final succinic acid concentration ranged from 4.74 to 9.02 g l−1. The highest succinic acid concentrations (experiments 3, 5, 8, 9 and 10) were coincident with the presence of NaHCO3 and MgSO4, and the maximum succinic acid concentration of 9.02 g l−1 was achieved in 24 h of incubation at 37°C and an agitation speed of 150 rpm, with the following medium composition: xylose, 20 g l−1; K2HPO4, 10.0 g l−1; MgSO4, 2.0 g l−1; yeast extract, 5.0 g l−1; NaHCO3, 10.0 g l−1.
Matrix of experimental 24−1 fractional factorial design and corresponding results on succinic acid concentration
Number of trialsa . | Parametersb . | Measured response . | |||
---|---|---|---|---|---|
A . | B . | C . | D . | Succinic acid (g l−1) . | |
K2HPO4 (g l−1) . | MgSO4 (g l−1) . | Yeast extract (g l−1) . | NaHCO3 (g l−1) . | ||
1 | 0.0 | 0.0 | 0.0 | 0.0 | 5.53 |
2 | 10.0 | 0.0 | 0.0 | 10.0 | 4.74 |
3 | 0.0 | 2.0 | 0.0 | 10.0 | 8.36 |
4 | 10.0 | 2.0 | 0.0 | 0.0 | 5.96 |
5 | 0.0 | 0.0 | 5.0 | 10.0 | 7.66 |
6 | 10.0 | 0.0 | 5.0 | 0.0 | 4.96 |
7 | 0.0 | 2.0 | 5.0 | 0.0 | 6.08 |
8 | 10.0 | 2.0 | 5.0 | 10.0 | 9.02 |
9 (CP) | 5.0 | 1.0 | 2.5 | 5.0 | 7.16 |
10 (CP) | 5.0 | 1.0 | 2.5 | 5.0 | 7.58 |
11 (CP) | 5.0 | 1.0 | 2.5 | 5.0 | 7.23 |
Number of trialsa . | Parametersb . | Measured response . | |||
---|---|---|---|---|---|
A . | B . | C . | D . | Succinic acid (g l−1) . | |
K2HPO4 (g l−1) . | MgSO4 (g l−1) . | Yeast extract (g l−1) . | NaHCO3 (g l−1) . | ||
1 | 0.0 | 0.0 | 0.0 | 0.0 | 5.53 |
2 | 10.0 | 0.0 | 0.0 | 10.0 | 4.74 |
3 | 0.0 | 2.0 | 0.0 | 10.0 | 8.36 |
4 | 10.0 | 2.0 | 0.0 | 0.0 | 5.96 |
5 | 0.0 | 0.0 | 5.0 | 10.0 | 7.66 |
6 | 10.0 | 0.0 | 5.0 | 0.0 | 4.96 |
7 | 0.0 | 2.0 | 5.0 | 0.0 | 6.08 |
8 | 10.0 | 2.0 | 5.0 | 10.0 | 9.02 |
9 (CP) | 5.0 | 1.0 | 2.5 | 5.0 | 7.16 |
10 (CP) | 5.0 | 1.0 | 2.5 | 5.0 | 7.58 |
11 (CP) | 5.0 | 1.0 | 2.5 | 5.0 | 7.23 |
aTrials: eight independent and three repetitions of the center point (CP)
bThe code for variables A, B, C, D are given in Table 1
Matrix of experimental 24−1 fractional factorial design and corresponding results on succinic acid concentration
Number of trialsa . | Parametersb . | Measured response . | |||
---|---|---|---|---|---|
A . | B . | C . | D . | Succinic acid (g l−1) . | |
K2HPO4 (g l−1) . | MgSO4 (g l−1) . | Yeast extract (g l−1) . | NaHCO3 (g l−1) . | ||
1 | 0.0 | 0.0 | 0.0 | 0.0 | 5.53 |
2 | 10.0 | 0.0 | 0.0 | 10.0 | 4.74 |
3 | 0.0 | 2.0 | 0.0 | 10.0 | 8.36 |
4 | 10.0 | 2.0 | 0.0 | 0.0 | 5.96 |
5 | 0.0 | 0.0 | 5.0 | 10.0 | 7.66 |
6 | 10.0 | 0.0 | 5.0 | 0.0 | 4.96 |
7 | 0.0 | 2.0 | 5.0 | 0.0 | 6.08 |
8 | 10.0 | 2.0 | 5.0 | 10.0 | 9.02 |
9 (CP) | 5.0 | 1.0 | 2.5 | 5.0 | 7.16 |
10 (CP) | 5.0 | 1.0 | 2.5 | 5.0 | 7.58 |
11 (CP) | 5.0 | 1.0 | 2.5 | 5.0 | 7.23 |
Number of trialsa . | Parametersb . | Measured response . | |||
---|---|---|---|---|---|
A . | B . | C . | D . | Succinic acid (g l−1) . | |
K2HPO4 (g l−1) . | MgSO4 (g l−1) . | Yeast extract (g l−1) . | NaHCO3 (g l−1) . | ||
1 | 0.0 | 0.0 | 0.0 | 0.0 | 5.53 |
2 | 10.0 | 0.0 | 0.0 | 10.0 | 4.74 |
3 | 0.0 | 2.0 | 0.0 | 10.0 | 8.36 |
4 | 10.0 | 2.0 | 0.0 | 0.0 | 5.96 |
5 | 0.0 | 0.0 | 5.0 | 10.0 | 7.66 |
6 | 10.0 | 0.0 | 5.0 | 0.0 | 4.96 |
7 | 0.0 | 2.0 | 5.0 | 0.0 | 6.08 |
8 | 10.0 | 2.0 | 5.0 | 10.0 | 9.02 |
9 (CP) | 5.0 | 1.0 | 2.5 | 5.0 | 7.16 |
10 (CP) | 5.0 | 1.0 | 2.5 | 5.0 | 7.58 |
11 (CP) | 5.0 | 1.0 | 2.5 | 5.0 | 7.23 |
aTrials: eight independent and three repetitions of the center point (CP)
bThe code for variables A, B, C, D are given in Table 1
According to the variance analysis, the best model for succinic acid concentration was the Reduced Quadratic Model, and the values of R2 (0.952) and pure error (0.064) show that the model is in agreement with the experimental data. The model remained significant, with a Fisher’s value of 29.85 and a statistically nonsignificant lack of fit (0.120) (P > 0.1), as shown in Table 4.
Variance analysis (ANOVA) of succinic acid concentration [partial sum of squares] in the experimental 24−1 fractional factorial design
Sourcea . | Sum of squares . | df . | Mean square . | F value . | P > F . |
---|---|---|---|---|---|
Model | 18.0797875 | 7 | 1.80797875 | 29.85843805 | <0.0001 |
A | 1.9110125 | 1 | 1.9110125 | 19.49093364 | 0.1159 |
B | 3.0876125 | 1 | 3.0876125 | 47.64834105 | 0.0916 |
C | 2.9701125 | 1 | 1.9701125 | 41.40297068 | 0.0982 |
D | 7.7815125 | 1 | 7.7815125 | 120.0850694 | 0.0579 |
AB | 0.8385125 | 1 | 0.8385125 | 12.94000772 | 0.1726 |
AC | 2.4090125 | 1 | 2.4090125 | 31.17611883 | 0.0922 |
AD | 0.0820125 | 1 | 0.0820125 | 1.265625 | 0.4626 |
Curvature | 1.3140625 | 1 | 1.3140625 | 30.27874228 | 0.0952 |
Lack of fit | 45.641850 | 9 | 4.564185 | 27.43712 | 0.1204 |
Pure error | 1.5634 | 5 | 0.0648 | ||
Correlation total | 19.04526 | 19 | |||
[Succinic acid]: [R2 = 0.9526, Adjusted R2 = 0.9399] |
Sourcea . | Sum of squares . | df . | Mean square . | F value . | P > F . |
---|---|---|---|---|---|
Model | 18.0797875 | 7 | 1.80797875 | 29.85843805 | <0.0001 |
A | 1.9110125 | 1 | 1.9110125 | 19.49093364 | 0.1159 |
B | 3.0876125 | 1 | 3.0876125 | 47.64834105 | 0.0916 |
C | 2.9701125 | 1 | 1.9701125 | 41.40297068 | 0.0982 |
D | 7.7815125 | 1 | 7.7815125 | 120.0850694 | 0.0579 |
AB | 0.8385125 | 1 | 0.8385125 | 12.94000772 | 0.1726 |
AC | 2.4090125 | 1 | 2.4090125 | 31.17611883 | 0.0922 |
AD | 0.0820125 | 1 | 0.0820125 | 1.265625 | 0.4626 |
Curvature | 1.3140625 | 1 | 1.3140625 | 30.27874228 | 0.0952 |
Lack of fit | 45.641850 | 9 | 4.564185 | 27.43712 | 0.1204 |
Pure error | 1.5634 | 5 | 0.0648 | ||
Correlation total | 19.04526 | 19 | |||
[Succinic acid]: [R2 = 0.9526, Adjusted R2 = 0.9399] |
ANOVA, Analysis of variance
aThe code for variables A, B, C, D are given in Table 1
Variance analysis (ANOVA) of succinic acid concentration [partial sum of squares] in the experimental 24−1 fractional factorial design
Sourcea . | Sum of squares . | df . | Mean square . | F value . | P > F . |
---|---|---|---|---|---|
Model | 18.0797875 | 7 | 1.80797875 | 29.85843805 | <0.0001 |
A | 1.9110125 | 1 | 1.9110125 | 19.49093364 | 0.1159 |
B | 3.0876125 | 1 | 3.0876125 | 47.64834105 | 0.0916 |
C | 2.9701125 | 1 | 1.9701125 | 41.40297068 | 0.0982 |
D | 7.7815125 | 1 | 7.7815125 | 120.0850694 | 0.0579 |
AB | 0.8385125 | 1 | 0.8385125 | 12.94000772 | 0.1726 |
AC | 2.4090125 | 1 | 2.4090125 | 31.17611883 | 0.0922 |
AD | 0.0820125 | 1 | 0.0820125 | 1.265625 | 0.4626 |
Curvature | 1.3140625 | 1 | 1.3140625 | 30.27874228 | 0.0952 |
Lack of fit | 45.641850 | 9 | 4.564185 | 27.43712 | 0.1204 |
Pure error | 1.5634 | 5 | 0.0648 | ||
Correlation total | 19.04526 | 19 | |||
[Succinic acid]: [R2 = 0.9526, Adjusted R2 = 0.9399] |
Sourcea . | Sum of squares . | df . | Mean square . | F value . | P > F . |
---|---|---|---|---|---|
Model | 18.0797875 | 7 | 1.80797875 | 29.85843805 | <0.0001 |
A | 1.9110125 | 1 | 1.9110125 | 19.49093364 | 0.1159 |
B | 3.0876125 | 1 | 3.0876125 | 47.64834105 | 0.0916 |
C | 2.9701125 | 1 | 1.9701125 | 41.40297068 | 0.0982 |
D | 7.7815125 | 1 | 7.7815125 | 120.0850694 | 0.0579 |
AB | 0.8385125 | 1 | 0.8385125 | 12.94000772 | 0.1726 |
AC | 2.4090125 | 1 | 2.4090125 | 31.17611883 | 0.0922 |
AD | 0.0820125 | 1 | 0.0820125 | 1.265625 | 0.4626 |
Curvature | 1.3140625 | 1 | 1.3140625 | 30.27874228 | 0.0952 |
Lack of fit | 45.641850 | 9 | 4.564185 | 27.43712 | 0.1204 |
Pure error | 1.5634 | 5 | 0.0648 | ||
Correlation total | 19.04526 | 19 | |||
[Succinic acid]: [R2 = 0.9526, Adjusted R2 = 0.9399] |
ANOVA, Analysis of variance
aThe code for variables A, B, C, D are given in Table 1
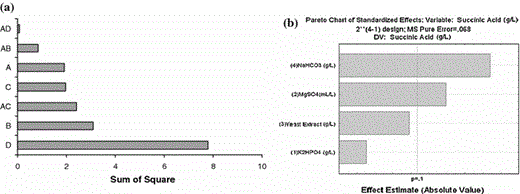
a Histogram of the influence of variables and their interactions, b Pareto chart for minimal confidence range of 90%, according to the sum of squares in 24−1 fractional factorial design. A K2HPO4, B MgSO4, C Yeast extract, D NaHCO3
Figure 2 reveals that NaHCO3 had a significant effect on achieving a high succinic acid concentration, indicating that in the next trial the concentration of this parameter should be increased. In contrast, K2HPO4 showed no significant effect (0.1159), suggesting that K2HPO4 could be eliminated from the medium. However, the interaction between K2HPO4 and yeast extract (AC) had a significant influence in this analysis, with values of P > F of less than 0.1 (0.0952). MgSO4 and K2HPO4, with their components magnesium and phosphorus, play an important role in cell physiology and morphology [8].
According to the results of the first experimental design, the greatest efficiency was obtained in experiment no. 8. ANOVA analysis showed that the maximum succinic acid concentration occurred when the highest levels of parameters (B) and ©) were combined with a lower concentration of K2HPO4, indicating that there is a maximum. However, the values of the central points are close to the maximum obtained in the investigated range, indicating the need to add axial points to a subsequent trial in order to optimize succinic acid production (in factorial design only linear models can be evaluated). Thus, the next step was to evaluate succinic acid production using the CCRD technique, since this approach enables the response surface to be predicted with curvature plots and the maximum values of the response variables to be visualized. The maximum succinic acid concentration (9.02 g l−1) can be enhanced, as the center points are still lower than the factorial points, showing an increasing tendency in the direction of the maximum point. All four parameters evaluated were significant (high F value) and kept in the model. The analysis of effects revealed that the levels of NaHCO3 and MgSO4 could be increased. However, any increase in K2HPO4 concentration may reduce succinic acid production since impurities, such as other organic acids (acetic and formic acids) and salts (HPO42−), can cause a decrease in the extraction yield of succinic acid from an aqueous phase due to their ionic strength. Thus, the concentration of K2HPO4 should be minimized in the fermentation process to optimize the downstream processing of succinic acid separation [9].
Central composite rotational design and analysis
The results of the CCRD are presented in Table 5. A set of 30 trials was generated (27 independent and 3 repetitions of the central point). According to the results of the second experimental design, an increase in succinic acid concentration was achieved, increasing from a maximum of 9.05 g l−1 (first experimental design) to 12.8 g l−1 (second experimental design—CCRD). The final succinic acid concentration varied between 6.04 and 12.8 g l−1. A maximum succinic acid concentration was obtained in experiment no. 2, with the following conditions: xylose concentration, 20 g l−1; K2HPO4, 5.0 g l−1; MgSO4, 2.0 g l−1; yeast extract, 3.0 g l−1; NaHCO3; 10 g l−1.
Matrix of experimental central composite rotational design (CCRD) investigating the effects of the concentrations of K2HPO4, MgSO4, yeast extract and NaHCO3 on succinic acid production
Number of trialsa . | Parametersb . | Measured response . | |||
---|---|---|---|---|---|
A . | B . | C . | D . | Succinic acid (g l−1) . | |
K2HPO4 (g l−1) . | MgSO4 (g l−1) . | Yeast extract (g l−1) . | NaHCO3 (g l−1) . | ||
1 | 5.0 | 4.0 | 3.0 | 5.0 | 11.55 |
2 | 5.0 | 2.0 | 3.0 | 10.0 | 12.85 |
3 | 10.0 | 3.0 | 5.0 | 15.0 | 11.12 |
4 | 10.0 | 3.0 | 1.0 | 15.0 | 11.42 |
5 | 7.5 | 2.0 | 0.0 | 10.0 | 6.04 |
6 | 7.5 | 4.0 | 3.0 | 1.5 | 10.45 |
7 | 5.0 | 4.0 | 1.0 | 5.0 | 10.26 |
8 | 7.5 | 2.0 | 3.0 | 10.0 | 9.42 |
9 | 10.0 | 2.0 | 1.0 | 15.0 | 9.10 |
10 | 10.0 | 1.0 | 5.0 | 15.0 | 8.45 |
11 | 10.0 | 4.0 | 5.0 | 5.0 | 12.15 |
12 | 10.0 | 3.0 | 1.0 | 5.0 | 12.10 |
13 | 12.5 | 4.0 | 3.0 | 10.0 | 12.27 |
14 | 10.0 | 4.0 | 3.0 | 20.0 | 12.45 |
15 | 5.0 | 2.0 | 5.0 | 15.0 | 7.85 |
16 | 7.5 | 2.0 | 3.0 | 10.0 | 7.12 |
17 | 7.5 | 3.0 | 3.0 | 10.0 | 12.20 |
18 | 5.0 | 4.0 | 1.0 | 15.0 | 12.41 |
19 | 5.0 | 3.0 | 5.0 | 5.0 | 11.02 |
20 | 7.5 | 3.0 | 5.0 | 5.0 | 10.78 |
21 | 5.0 | 4.0 | 1.0 | 5.0 | 7.56 |
22 | 10.0 | 3.0 | 1.0 | 5.0 | 10.23 |
23 | 2.5 | 2.0 | 3.0 | 10.0 | 8.86 |
24 | 5.0 | 2.0 | 1.0 | 15.0 | 6.54 |
25 | 5.0 | 3.0 | 5.0 | 15.0 | 7.45 |
26 | 7.5 | 3.0 | 7.0 | 10.0 | 11.24 |
27 | 7.5 | 2.0 | 3.0 | 10.0 | 9.25 |
28 (CP) | 7.5 | 3.0 | 3.0 | 10.0 | 10.15 |
29 (CP) | 7.5 | 3.0 | 3.0 | 10.0 | 11.23 |
30 (CP) | 7.5 | 3.0 | 3.0 | 10.0 | 11.12 |
Number of trialsa . | Parametersb . | Measured response . | |||
---|---|---|---|---|---|
A . | B . | C . | D . | Succinic acid (g l−1) . | |
K2HPO4 (g l−1) . | MgSO4 (g l−1) . | Yeast extract (g l−1) . | NaHCO3 (g l−1) . | ||
1 | 5.0 | 4.0 | 3.0 | 5.0 | 11.55 |
2 | 5.0 | 2.0 | 3.0 | 10.0 | 12.85 |
3 | 10.0 | 3.0 | 5.0 | 15.0 | 11.12 |
4 | 10.0 | 3.0 | 1.0 | 15.0 | 11.42 |
5 | 7.5 | 2.0 | 0.0 | 10.0 | 6.04 |
6 | 7.5 | 4.0 | 3.0 | 1.5 | 10.45 |
7 | 5.0 | 4.0 | 1.0 | 5.0 | 10.26 |
8 | 7.5 | 2.0 | 3.0 | 10.0 | 9.42 |
9 | 10.0 | 2.0 | 1.0 | 15.0 | 9.10 |
10 | 10.0 | 1.0 | 5.0 | 15.0 | 8.45 |
11 | 10.0 | 4.0 | 5.0 | 5.0 | 12.15 |
12 | 10.0 | 3.0 | 1.0 | 5.0 | 12.10 |
13 | 12.5 | 4.0 | 3.0 | 10.0 | 12.27 |
14 | 10.0 | 4.0 | 3.0 | 20.0 | 12.45 |
15 | 5.0 | 2.0 | 5.0 | 15.0 | 7.85 |
16 | 7.5 | 2.0 | 3.0 | 10.0 | 7.12 |
17 | 7.5 | 3.0 | 3.0 | 10.0 | 12.20 |
18 | 5.0 | 4.0 | 1.0 | 15.0 | 12.41 |
19 | 5.0 | 3.0 | 5.0 | 5.0 | 11.02 |
20 | 7.5 | 3.0 | 5.0 | 5.0 | 10.78 |
21 | 5.0 | 4.0 | 1.0 | 5.0 | 7.56 |
22 | 10.0 | 3.0 | 1.0 | 5.0 | 10.23 |
23 | 2.5 | 2.0 | 3.0 | 10.0 | 8.86 |
24 | 5.0 | 2.0 | 1.0 | 15.0 | 6.54 |
25 | 5.0 | 3.0 | 5.0 | 15.0 | 7.45 |
26 | 7.5 | 3.0 | 7.0 | 10.0 | 11.24 |
27 | 7.5 | 2.0 | 3.0 | 10.0 | 9.25 |
28 (CP) | 7.5 | 3.0 | 3.0 | 10.0 | 10.15 |
29 (CP) | 7.5 | 3.0 | 3.0 | 10.0 | 11.23 |
30 (CP) | 7.5 | 3.0 | 3.0 | 10.0 | 11.12 |
aTrials: eight independent and three repetitions of the CP
bThe code for variables A, B, C, D are given in Table 1
Matrix of experimental central composite rotational design (CCRD) investigating the effects of the concentrations of K2HPO4, MgSO4, yeast extract and NaHCO3 on succinic acid production
Number of trialsa . | Parametersb . | Measured response . | |||
---|---|---|---|---|---|
A . | B . | C . | D . | Succinic acid (g l−1) . | |
K2HPO4 (g l−1) . | MgSO4 (g l−1) . | Yeast extract (g l−1) . | NaHCO3 (g l−1) . | ||
1 | 5.0 | 4.0 | 3.0 | 5.0 | 11.55 |
2 | 5.0 | 2.0 | 3.0 | 10.0 | 12.85 |
3 | 10.0 | 3.0 | 5.0 | 15.0 | 11.12 |
4 | 10.0 | 3.0 | 1.0 | 15.0 | 11.42 |
5 | 7.5 | 2.0 | 0.0 | 10.0 | 6.04 |
6 | 7.5 | 4.0 | 3.0 | 1.5 | 10.45 |
7 | 5.0 | 4.0 | 1.0 | 5.0 | 10.26 |
8 | 7.5 | 2.0 | 3.0 | 10.0 | 9.42 |
9 | 10.0 | 2.0 | 1.0 | 15.0 | 9.10 |
10 | 10.0 | 1.0 | 5.0 | 15.0 | 8.45 |
11 | 10.0 | 4.0 | 5.0 | 5.0 | 12.15 |
12 | 10.0 | 3.0 | 1.0 | 5.0 | 12.10 |
13 | 12.5 | 4.0 | 3.0 | 10.0 | 12.27 |
14 | 10.0 | 4.0 | 3.0 | 20.0 | 12.45 |
15 | 5.0 | 2.0 | 5.0 | 15.0 | 7.85 |
16 | 7.5 | 2.0 | 3.0 | 10.0 | 7.12 |
17 | 7.5 | 3.0 | 3.0 | 10.0 | 12.20 |
18 | 5.0 | 4.0 | 1.0 | 15.0 | 12.41 |
19 | 5.0 | 3.0 | 5.0 | 5.0 | 11.02 |
20 | 7.5 | 3.0 | 5.0 | 5.0 | 10.78 |
21 | 5.0 | 4.0 | 1.0 | 5.0 | 7.56 |
22 | 10.0 | 3.0 | 1.0 | 5.0 | 10.23 |
23 | 2.5 | 2.0 | 3.0 | 10.0 | 8.86 |
24 | 5.0 | 2.0 | 1.0 | 15.0 | 6.54 |
25 | 5.0 | 3.0 | 5.0 | 15.0 | 7.45 |
26 | 7.5 | 3.0 | 7.0 | 10.0 | 11.24 |
27 | 7.5 | 2.0 | 3.0 | 10.0 | 9.25 |
28 (CP) | 7.5 | 3.0 | 3.0 | 10.0 | 10.15 |
29 (CP) | 7.5 | 3.0 | 3.0 | 10.0 | 11.23 |
30 (CP) | 7.5 | 3.0 | 3.0 | 10.0 | 11.12 |
Number of trialsa . | Parametersb . | Measured response . | |||
---|---|---|---|---|---|
A . | B . | C . | D . | Succinic acid (g l−1) . | |
K2HPO4 (g l−1) . | MgSO4 (g l−1) . | Yeast extract (g l−1) . | NaHCO3 (g l−1) . | ||
1 | 5.0 | 4.0 | 3.0 | 5.0 | 11.55 |
2 | 5.0 | 2.0 | 3.0 | 10.0 | 12.85 |
3 | 10.0 | 3.0 | 5.0 | 15.0 | 11.12 |
4 | 10.0 | 3.0 | 1.0 | 15.0 | 11.42 |
5 | 7.5 | 2.0 | 0.0 | 10.0 | 6.04 |
6 | 7.5 | 4.0 | 3.0 | 1.5 | 10.45 |
7 | 5.0 | 4.0 | 1.0 | 5.0 | 10.26 |
8 | 7.5 | 2.0 | 3.0 | 10.0 | 9.42 |
9 | 10.0 | 2.0 | 1.0 | 15.0 | 9.10 |
10 | 10.0 | 1.0 | 5.0 | 15.0 | 8.45 |
11 | 10.0 | 4.0 | 5.0 | 5.0 | 12.15 |
12 | 10.0 | 3.0 | 1.0 | 5.0 | 12.10 |
13 | 12.5 | 4.0 | 3.0 | 10.0 | 12.27 |
14 | 10.0 | 4.0 | 3.0 | 20.0 | 12.45 |
15 | 5.0 | 2.0 | 5.0 | 15.0 | 7.85 |
16 | 7.5 | 2.0 | 3.0 | 10.0 | 7.12 |
17 | 7.5 | 3.0 | 3.0 | 10.0 | 12.20 |
18 | 5.0 | 4.0 | 1.0 | 15.0 | 12.41 |
19 | 5.0 | 3.0 | 5.0 | 5.0 | 11.02 |
20 | 7.5 | 3.0 | 5.0 | 5.0 | 10.78 |
21 | 5.0 | 4.0 | 1.0 | 5.0 | 7.56 |
22 | 10.0 | 3.0 | 1.0 | 5.0 | 10.23 |
23 | 2.5 | 2.0 | 3.0 | 10.0 | 8.86 |
24 | 5.0 | 2.0 | 1.0 | 15.0 | 6.54 |
25 | 5.0 | 3.0 | 5.0 | 15.0 | 7.45 |
26 | 7.5 | 3.0 | 7.0 | 10.0 | 11.24 |
27 | 7.5 | 2.0 | 3.0 | 10.0 | 9.25 |
28 (CP) | 7.5 | 3.0 | 3.0 | 10.0 | 10.15 |
29 (CP) | 7.5 | 3.0 | 3.0 | 10.0 | 11.23 |
30 (CP) | 7.5 | 3.0 | 3.0 | 10.0 | 11.12 |
aTrials: eight independent and three repetitions of the CP
bThe code for variables A, B, C, D are given in Table 1
The data presented in Table 6 corroborate the results of the experimental 24−1 fractional factorial design in showing that the best model for succinic acid concentration was the Reduced Quadratic Model, based on the highest value for R2 (0.995), indicating 99.5% of the response variability. This model remained significant, with a Fisher value of 34.81, thus denoting a curvilinear plane, as can be observed in the ANOVA (Table 4). Moreover, the residue was low and statistically nonsignificant, which did not invalidate the model for predictive purposes because the equation had a high R2 value. The lack of fit was not significant (P > 0.05) which, combined with the F values of the parameters and the determination coefficient (R2), showed that the model adequately adjusts to the experimental points, representing the confidence of the results [2].
ANOVA for succinic acid concentration [partial sum of squares] in the experimental CCRD
Sourcea . | Sum of squares . | df . | Mean square . | F value . | P > F . |
---|---|---|---|---|---|
Model | 149.903075 | 14 | 14.9903075 | 34.81492204 | <0.0001 |
A | 0.881666667 | 1 | 0.881666667 | 0.624275956 | 0.4418 |
B | 22.08001667 | 1 | 22.08001667 | 45.63405313 | 0.0013 |
C | 8.401666667 | 1 | 8.401666667 | 35.948913221 | 0.0276 |
D | 88.16666667 | 1 | 88.16666667 | 62.42759559 | <0.0001 |
AB | 7.1824 | 1 | 7.1824 | 5.085595039 | 0.0395 |
AC | 0.126025 | 1 | 0.126025 | 10.089233698 | 0.7693 |
AD | 1.177225 | 1 | 1.177225 | 20.833550014 | 0.3757 |
BC | 4.3264 | 1 | 4.3264 | 33.06336578 | 0.1000 |
BD | 4.2849 | 1 | 4.2849 | 8.033981146 | 0.1020 |
CD | 0.912025 | 1 | 0.912025 | 0.645771583 | 0.4342 |
Residual | 21.18454167 | 15 | 1.412302778 | ||
Lack of fit | 39.18699167 | 9 | 3.918699167 | 35.80263114 | 0.0486 |
Pure error | 5.99755 | 5 | 0.39951 | ||
[Succinic acid]: [R2 = 0.995, Adjusted R2 = 0.9699] |
Sourcea . | Sum of squares . | df . | Mean square . | F value . | P > F . |
---|---|---|---|---|---|
Model | 149.903075 | 14 | 14.9903075 | 34.81492204 | <0.0001 |
A | 0.881666667 | 1 | 0.881666667 | 0.624275956 | 0.4418 |
B | 22.08001667 | 1 | 22.08001667 | 45.63405313 | 0.0013 |
C | 8.401666667 | 1 | 8.401666667 | 35.948913221 | 0.0276 |
D | 88.16666667 | 1 | 88.16666667 | 62.42759559 | <0.0001 |
AB | 7.1824 | 1 | 7.1824 | 5.085595039 | 0.0395 |
AC | 0.126025 | 1 | 0.126025 | 10.089233698 | 0.7693 |
AD | 1.177225 | 1 | 1.177225 | 20.833550014 | 0.3757 |
BC | 4.3264 | 1 | 4.3264 | 33.06336578 | 0.1000 |
BD | 4.2849 | 1 | 4.2849 | 8.033981146 | 0.1020 |
CD | 0.912025 | 1 | 0.912025 | 0.645771583 | 0.4342 |
Residual | 21.18454167 | 15 | 1.412302778 | ||
Lack of fit | 39.18699167 | 9 | 3.918699167 | 35.80263114 | 0.0486 |
Pure error | 5.99755 | 5 | 0.39951 | ||
[Succinic acid]: [R2 = 0.995, Adjusted R2 = 0.9699] |
aThe code for variables A, B, C, D are given in Table 1
ANOVA for succinic acid concentration [partial sum of squares] in the experimental CCRD
Sourcea . | Sum of squares . | df . | Mean square . | F value . | P > F . |
---|---|---|---|---|---|
Model | 149.903075 | 14 | 14.9903075 | 34.81492204 | <0.0001 |
A | 0.881666667 | 1 | 0.881666667 | 0.624275956 | 0.4418 |
B | 22.08001667 | 1 | 22.08001667 | 45.63405313 | 0.0013 |
C | 8.401666667 | 1 | 8.401666667 | 35.948913221 | 0.0276 |
D | 88.16666667 | 1 | 88.16666667 | 62.42759559 | <0.0001 |
AB | 7.1824 | 1 | 7.1824 | 5.085595039 | 0.0395 |
AC | 0.126025 | 1 | 0.126025 | 10.089233698 | 0.7693 |
AD | 1.177225 | 1 | 1.177225 | 20.833550014 | 0.3757 |
BC | 4.3264 | 1 | 4.3264 | 33.06336578 | 0.1000 |
BD | 4.2849 | 1 | 4.2849 | 8.033981146 | 0.1020 |
CD | 0.912025 | 1 | 0.912025 | 0.645771583 | 0.4342 |
Residual | 21.18454167 | 15 | 1.412302778 | ||
Lack of fit | 39.18699167 | 9 | 3.918699167 | 35.80263114 | 0.0486 |
Pure error | 5.99755 | 5 | 0.39951 | ||
[Succinic acid]: [R2 = 0.995, Adjusted R2 = 0.9699] |
Sourcea . | Sum of squares . | df . | Mean square . | F value . | P > F . |
---|---|---|---|---|---|
Model | 149.903075 | 14 | 14.9903075 | 34.81492204 | <0.0001 |
A | 0.881666667 | 1 | 0.881666667 | 0.624275956 | 0.4418 |
B | 22.08001667 | 1 | 22.08001667 | 45.63405313 | 0.0013 |
C | 8.401666667 | 1 | 8.401666667 | 35.948913221 | 0.0276 |
D | 88.16666667 | 1 | 88.16666667 | 62.42759559 | <0.0001 |
AB | 7.1824 | 1 | 7.1824 | 5.085595039 | 0.0395 |
AC | 0.126025 | 1 | 0.126025 | 10.089233698 | 0.7693 |
AD | 1.177225 | 1 | 1.177225 | 20.833550014 | 0.3757 |
BC | 4.3264 | 1 | 4.3264 | 33.06336578 | 0.1000 |
BD | 4.2849 | 1 | 4.2849 | 8.033981146 | 0.1020 |
CD | 0.912025 | 1 | 0.912025 | 0.645771583 | 0.4342 |
Residual | 21.18454167 | 15 | 1.412302778 | ||
Lack of fit | 39.18699167 | 9 | 3.918699167 | 35.80263114 | 0.0486 |
Pure error | 5.99755 | 5 | 0.39951 | ||
[Succinic acid]: [R2 = 0.995, Adjusted R2 = 0.9699] |
aThe code for variables A, B, C, D are given in Table 1
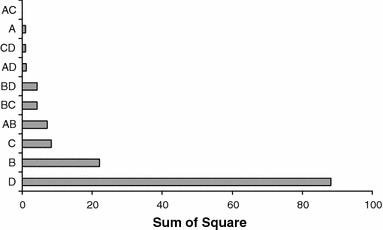
Histogram of the influence of the process variables and their interactions, according to the sum of squares in the experimental central composite rotational design (CCRD). A K2HPO4, B MgSO4, C yeast extract, D NaHCO3
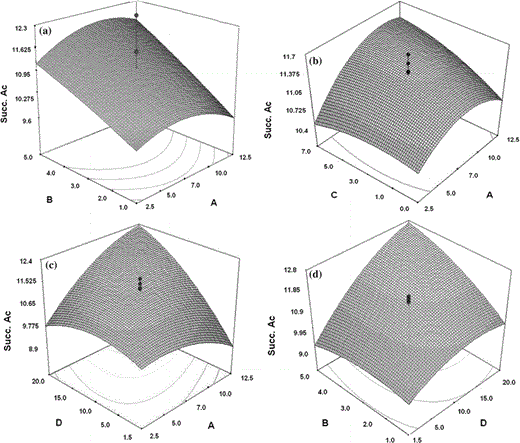
Response surface plots showing the effect of parameters and their combined effects on succinic acid concentration. Interaction between the parameters AB (a), AC (b), AD (c), BD (d). A K2HPO4, B MgSO4, C Yeast extract, D NaHCO3
Succinic acid production was increased by A. succinogenes when an optimal combination of nutrients was used. Analysis of the response measured (concentration of succinic acid) emphasizes experimental condition number 2 since it is the most efficient. The effect of NaHCO3, keeping MgSO4 to a minimum level of (2.0 g l−1) and yeast extract at the center point (3.0 g l−1), indicates that the optimal point for succinic acid production is around the maximum level of NaHCO3 (10.0 g l−1). Therefore, when the fermentation process occurs in the presence of high levels of K2HPO4, succinic acid concentration is affected. All of the nutrients were significant and essential to the metabolism of the bacteria. The concentrations of amino nitrogen (yeast extract) in the fermentation broth usually reflect the concentrations of proteins and amino acids [21].
Liu et al. [16] demonstrated that there was no significant inhibition of cell growth or succinic acid production by magnesium ions using A. succinogenes strain CGMCC1593. Nitrogen sources generally play a significant role because these nutrients are directly linked with cell proliferation and metabolite biosynthesis. It has been shown that yeast extract, which contains protein, lipid, vitamins and others, supplies important growth factors for succinic acid production, but its cost may be a limitation for its application in industrial processes [25]. Recent studies on similar types of fermentations have demonstrated that the nitrogen deficiency stimulated the biosynthesis of other metabolites, such as acetic and formic acids [20]. Variance analysis showed the adequacy of the model, which was validated experimentally in the bioreactor.
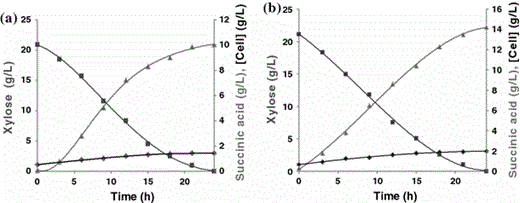
Concentration of cells (filled diamond), xylose (filled square) and succinic acid (filled triangle) during the fermentation process carried out under controlled conditions (37°C, pH 7.0, 150 rpm) using synthetic medium with 22.0 g/l of xylose. Medium with low (a) and high (b) CO2 supply
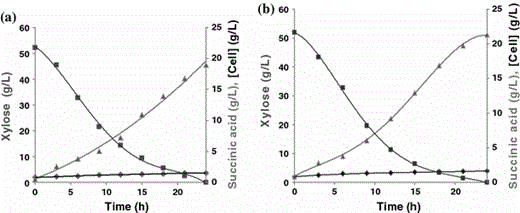
Concentration of cells (filled diamond), xylose (filled square) and succinic acid (filled triangle) during the fermentation of sugarcane bagasse hemicellulose hydrolysate containing 52.0 g l−1 xylose, carried out under controlled conditions (37°C, pH 7.0, 150 rpm). Medium with low (a) and high (b) CO2 supply
Succinic acid fermentation
The best conditions for the fermentation process indicated by CCRD (Table 5) were used to prepare the fermentation medium for succinic acid production in the bioreactor experiment. Under response surface methodology (RSM)-optimized conditions, a concentration of 10.04 g l−1 succinic acid, a volumetric productivity (Q P) of 0.478 g l−1 h−1 and an efficiency (E f) of 58.4% were obtained in 24 h (Fig. 5a). An increase in succinic acid production was subsequently obtained in a second bioreactor operated with a supply of external CO2, resulting in a final succinic acid concentration of 14.22 g l−1, a volumetric productivity of 0.677 g l−1 h−1 and an efficiency (E f) of 82.8% (Fig. 5b). A similar trend was reported by Lee et al. [13], who observed that 13.5 g l−1 of succinic acid was produced when 100% CO2 was supplied to the bacterium M. succiniciproducens. These outcomes were likely achieved because of the supply of carbon dioxide. Similarly, van der Werf et al. [26] suggested that the production of succinate requires CO2 fixation and also that CO2 concentration regulates the level of the key enzymes of the PEP carboxykinase pathway in A. succinogenes. These researchers further confirmed that high levels of CO2 stimulated PEP carboxykinase levels, whereas the levels of alcohol dehydrogenase and lactate dehydrogenases were significantly decreased.
Succinic acid fermentation of sugarcane bagasse hemicellulose hydrolysate
The final verification of the statistical model for succinic acid production was also carried in a 2-l bioreactor. Figure 6 shows the behavior of the hydrolysate during the fermentation process under the conditions predicted in the statistical analysis. The hydrolysate was inoculated with a cell concentration of 1.02 g l−1; the fermentation was run at 37°C for 24 h, pH 7.0, with an agitation of 150 rpm, and the initial xylose concentration was 52 g l−1.
The succinic acid concentration reached its maximum value (19.0 g l−1) after 24 h of fermentation, corresponding to a yield of product per substrate consumed of 0.465 g g−1, a volumetric productivity of 0.903 g l−1 h−1 and a fermentation efficiency of 45.6% (Fig. 6a).
The effect of 10 g l−1 of NaHCO3 was similar to that of MgCO3 used by Vemuri et al. [27], who obtained a final succinic acid concentration of 10.53 g l−1; nonetheless, concentrations of NaHCO3 higher than 10.0 g l−1 did not significantly increase the succinic acid concentration. The results of this analysis also suggest strategies for improving CO2 utilization. For example, at intermediate CO2 concentrations, overexpression of the carbonic anhydrase enzyme, which catalyzes the hydration of CO2 to HCO3−, may be effective. If pure CO2 were to be used, further improvement in succinate production should result from increased enzyme expression, as has been previously observed with pyruvate carboxylase [27].
Similarly, Fig. 6b shows the fermentation of hemicellulose hydrolysate in the presence of carbon dioxide, which was continuously introduced into the medium at 0.05 vvm throughout the process. The final succinic acid concentration, volumetric productivity and efficiency were 22.5 g l−1, 1.014 g l−1 h−1 and 55.4%, respectively, with a fermentation time of approximately 24 h, at a temperature of 37°C, agitation of 150 rpm and pH 7.0 (Table 7).
Results of the fermentation process for succinic acid production carried out in a bioreactor under controlled conditions (37°C, pH 7.0, 150 rpm)
Fermentation process . | Conditions . | Maximum values . | ||||||||
---|---|---|---|---|---|---|---|---|---|---|
So (g l−1) . | Time (h) . | Low CO2 tension . | High CO2 tension . | |||||||
AS (g l−1) . | Q P (g l−1 h−1) . | Y P/S (g/g) . | E f (%) . | AS (g/l) . | Y P/S (g g−1) . | E f (%) . | Q p (g l−1 h−1) . | |||
Synthetic medium | 22.0 | 24 | 10.04 | 0.47 | 0.45 | 58.4 | 14.2 | 0.64 | 82.8 | 0.67 |
Hydrolysate | 52.0 | 24 | 18.5 | 0.90 | 0.35 | 45.6 | 22.5 | 0.43 | 55.4 | 1.01 |
Fermentation process . | Conditions . | Maximum values . | ||||||||
---|---|---|---|---|---|---|---|---|---|---|
So (g l−1) . | Time (h) . | Low CO2 tension . | High CO2 tension . | |||||||
AS (g l−1) . | Q P (g l−1 h−1) . | Y P/S (g/g) . | E f (%) . | AS (g/l) . | Y P/S (g g−1) . | E f (%) . | Q p (g l−1 h−1) . | |||
Synthetic medium | 22.0 | 24 | 10.04 | 0.47 | 0.45 | 58.4 | 14.2 | 0.64 | 82.8 | 0.67 |
Hydrolysate | 52.0 | 24 | 18.5 | 0.90 | 0.35 | 45.6 | 22.5 | 0.43 | 55.4 | 1.01 |
S 0 Initial xylose concentration (g l−1), AS succinic acid (g l−1), Q P productivity (g l−1 h−1), Y P/S product yield in relation to the substrate, E f efficiency
Results of the fermentation process for succinic acid production carried out in a bioreactor under controlled conditions (37°C, pH 7.0, 150 rpm)
Fermentation process . | Conditions . | Maximum values . | ||||||||
---|---|---|---|---|---|---|---|---|---|---|
So (g l−1) . | Time (h) . | Low CO2 tension . | High CO2 tension . | |||||||
AS (g l−1) . | Q P (g l−1 h−1) . | Y P/S (g/g) . | E f (%) . | AS (g/l) . | Y P/S (g g−1) . | E f (%) . | Q p (g l−1 h−1) . | |||
Synthetic medium | 22.0 | 24 | 10.04 | 0.47 | 0.45 | 58.4 | 14.2 | 0.64 | 82.8 | 0.67 |
Hydrolysate | 52.0 | 24 | 18.5 | 0.90 | 0.35 | 45.6 | 22.5 | 0.43 | 55.4 | 1.01 |
Fermentation process . | Conditions . | Maximum values . | ||||||||
---|---|---|---|---|---|---|---|---|---|---|
So (g l−1) . | Time (h) . | Low CO2 tension . | High CO2 tension . | |||||||
AS (g l−1) . | Q P (g l−1 h−1) . | Y P/S (g/g) . | E f (%) . | AS (g/l) . | Y P/S (g g−1) . | E f (%) . | Q p (g l−1 h−1) . | |||
Synthetic medium | 22.0 | 24 | 10.04 | 0.47 | 0.45 | 58.4 | 14.2 | 0.64 | 82.8 | 0.67 |
Hydrolysate | 52.0 | 24 | 18.5 | 0.90 | 0.35 | 45.6 | 22.5 | 0.43 | 55.4 | 1.01 |
S 0 Initial xylose concentration (g l−1), AS succinic acid (g l−1), Q P productivity (g l−1 h−1), Y P/S product yield in relation to the substrate, E f efficiency
Table 8 shows the results previously reported in the literature using various bacterial strains in succinic acid fermentation processes as well as the main results obtained in our study. A review of the relevant literature reveals that many published studies used other materials, such as wood hydrolysate and corn steep liquor [1, 14], and other microorganisms, such as naturally occurring M. succiniciproducens [9, 10, 13], A. succiniciproducens [12, 18] and genetically engineered E. coli [15, 19]. Other authors reported that A. succinogenes could use a wide range of carbohydrates as carbon sources and ferment straw hydrolysate or cane molasses, making this species a promising agent for succinic acid production [16].
Main results reported in the literature for succinic acid production
So (g l−1) . | Q p (g l−1 h−1) . | Y p/s (g/g) . | Carbon source . | Organism . | Cultivation . | Reference . |
---|---|---|---|---|---|---|
35.6 | 1.01 | 0.82 | Wheat | Actinobacillus succinogenes | Fed-batch | Du et al. [6] |
43.0 | 0.72 | 0.53 | Glucose | Escherichia coli | Batch | Lin et al. [15] |
58.3 | 1.08 | 0.62 | Glucose | Escherichia coli | Fed-batch | Lin et al. [15] |
83.0 | 10.40 | 0.89 | Glucose | Anaerobiospirillum succiniciproducens | Fed-batch/electrodialysis | Meynial-Salles et al. [18] |
55.2 | 1.15 | – | Cane molasses | Actinobacillus succinogenes | Batch | Liu et al. [16] |
52.0 | 1.80 | 0.76 | Glucose | Mannheimia succiniciproducens | Batch | Lee et al. [13] |
22.0 | 0.67 | 0.64 | Xylose | Actinobacillus succinogenes | Batch | This work |
52.0 | 1.01 | 0.43 | sugarcane bagasse | Actinobacillus succinogenes | Batch | This work |
So (g l−1) . | Q p (g l−1 h−1) . | Y p/s (g/g) . | Carbon source . | Organism . | Cultivation . | Reference . |
---|---|---|---|---|---|---|
35.6 | 1.01 | 0.82 | Wheat | Actinobacillus succinogenes | Fed-batch | Du et al. [6] |
43.0 | 0.72 | 0.53 | Glucose | Escherichia coli | Batch | Lin et al. [15] |
58.3 | 1.08 | 0.62 | Glucose | Escherichia coli | Fed-batch | Lin et al. [15] |
83.0 | 10.40 | 0.89 | Glucose | Anaerobiospirillum succiniciproducens | Fed-batch/electrodialysis | Meynial-Salles et al. [18] |
55.2 | 1.15 | – | Cane molasses | Actinobacillus succinogenes | Batch | Liu et al. [16] |
52.0 | 1.80 | 0.76 | Glucose | Mannheimia succiniciproducens | Batch | Lee et al. [13] |
22.0 | 0.67 | 0.64 | Xylose | Actinobacillus succinogenes | Batch | This work |
52.0 | 1.01 | 0.43 | sugarcane bagasse | Actinobacillus succinogenes | Batch | This work |
Main results reported in the literature for succinic acid production
So (g l−1) . | Q p (g l−1 h−1) . | Y p/s (g/g) . | Carbon source . | Organism . | Cultivation . | Reference . |
---|---|---|---|---|---|---|
35.6 | 1.01 | 0.82 | Wheat | Actinobacillus succinogenes | Fed-batch | Du et al. [6] |
43.0 | 0.72 | 0.53 | Glucose | Escherichia coli | Batch | Lin et al. [15] |
58.3 | 1.08 | 0.62 | Glucose | Escherichia coli | Fed-batch | Lin et al. [15] |
83.0 | 10.40 | 0.89 | Glucose | Anaerobiospirillum succiniciproducens | Fed-batch/electrodialysis | Meynial-Salles et al. [18] |
55.2 | 1.15 | – | Cane molasses | Actinobacillus succinogenes | Batch | Liu et al. [16] |
52.0 | 1.80 | 0.76 | Glucose | Mannheimia succiniciproducens | Batch | Lee et al. [13] |
22.0 | 0.67 | 0.64 | Xylose | Actinobacillus succinogenes | Batch | This work |
52.0 | 1.01 | 0.43 | sugarcane bagasse | Actinobacillus succinogenes | Batch | This work |
So (g l−1) . | Q p (g l−1 h−1) . | Y p/s (g/g) . | Carbon source . | Organism . | Cultivation . | Reference . |
---|---|---|---|---|---|---|
35.6 | 1.01 | 0.82 | Wheat | Actinobacillus succinogenes | Fed-batch | Du et al. [6] |
43.0 | 0.72 | 0.53 | Glucose | Escherichia coli | Batch | Lin et al. [15] |
58.3 | 1.08 | 0.62 | Glucose | Escherichia coli | Fed-batch | Lin et al. [15] |
83.0 | 10.40 | 0.89 | Glucose | Anaerobiospirillum succiniciproducens | Fed-batch/electrodialysis | Meynial-Salles et al. [18] |
55.2 | 1.15 | – | Cane molasses | Actinobacillus succinogenes | Batch | Liu et al. [16] |
52.0 | 1.80 | 0.76 | Glucose | Mannheimia succiniciproducens | Batch | Lee et al. [13] |
22.0 | 0.67 | 0.64 | Xylose | Actinobacillus succinogenes | Batch | This work |
52.0 | 1.01 | 0.43 | sugarcane bagasse | Actinobacillus succinogenes | Batch | This work |
Conclusions
The sequential experimental design strategy used to study the addition of nutrients in the fermentation medium was an important tool for maximizing final succinic acid concentration through RSM.
The results of our study on succinic acid production from sugarcane bagasse hemicellulose hydrolysate by means of A. succinogenes fermentation indicate that it is a promising procedure provided that an optimal combination of nutrients is used. Increased levels of NaHCO3 and MgSO4 were primarily responsible for the increased succinic acid concentration, followed by yeast extract and KH2PO4, which were less significant as independent variables, but revealed themselves to be important when they were involved in the interactions.
The conversion yield of succinic acid from sugarcane bagasse was relatively high in the batch cultivation of A. succinogenes. Under the best conversion conditions, dictated by the results of our statistical analysis and validated experimentally, a succinic acid concentration of 22.5 g l−1 was achieved in hemicellulose hydrolysate fermentation.
Succinic acid will likely be one of the future platform chemical derivatives from renewable resources. Microbial production of succinic acid, in particular, may prove a novel “green technology,” since CO2 fixation is integrated to carboxylation and decarboxylation in both catabolism and anabolism. Based on recent research results, cost reduction and scale-up are the main objectives of new process development in this area.
Acknowledgments
The authors are grateful to the Brazilian Council for Research (CNPq); the Rio de Janeiro Foundation for Science and Technology (FAPERJ) and the Brazilian Oil Company (PETROBRAS) for financial support of this research.
References
Betancur GV, Pereira Jr N (2010) sugarcane bagasse as feedstock for second generation ethanol production. Part I: diluted acid pre-treatment optimization. Electron J Biotechnol. Available at: http://www.ejbiotechnology.info/content/vol13/issue3/full/3/
Kim DY, Yim SC, Lee PC, Lee WG, Lee SY, Chang HN (2004) Batch and continuous fermentation of succinic acid from wood hydrolyzate by Mannheimia succiniciproducens MBEL55E. Enzyme Microb Technol 34(6–7):648–653
Pereira N, Couto MAPG, Santa Anna LM (2008) Series on biotechnology: biomass of lignocellulosic composition for fuel ethanol production within the context of biorefinery. Amigadigital Press, Rio de Janeiro