-
PDF
- Split View
-
Views
-
Cite
Cite
Raunel Tinoco, Abisaí Acevedo, Enrique Galindo, Leobardo Serrano-Carreón, Increasing Pleurotus ostreatus laccase production by culture medium optimization and copper/lignin synergistic induction, Journal of Industrial Microbiology and Biotechnology, Volume 38, Issue 4, 1 April 2011, Pages 531–540, https://doi.org/10.1007/s10295-010-0797-3
- Share Icon Share
Abstract
Laccases have great biotechnological potential in diverse industries as they catalyze the oxidation of a broad variety of chemical compounds. Production of laccases by basidiomycetes has been broadly studied as they secrete the enzymes, grow on cheap substrates, and they generally produce more than one isoenzyme (constitutive and/or inducible). Laccase production and isoenzyme profile can be modified through medium composition and the use of inducers. The objective of this work was to increase laccase production by Pleurotus ostreatus CP-50 through culture medium optimization and the simultaneous use of copper and lignin as inducers. Increased fungal growth was obtained through the use of a factorial fractional experimental design 26–2 where the influence of the nature and concentration of carbon and nitrogen sources was assessed. Although specific laccase production (U/mg biomass) decreased when malt extract medium was supplemented with carbon and nitrogen sources, fungal growth and laccase volumetric activity increased four and sixfold, respectively. The effect of media supplementation with copper and/or lignin on laccase production by P. ostreatus CP-50 was studied. A positive synergistic effect between copper and lignin was observed on laccase production. Overall, the use of an optimized medium and the simultaneous addition of copper and lignin improved growth, laccase volumetric activity, and process productivity by 4-, 60-, and 10-fold, respectively.
Introduction
Laccases (p-diphenol:oxygen oxidoreductases, EC 1.10.3.2) are multicopper enzymes that catalyze the oxidation of a broad variety of substrates such as mono-, di-, and polyphenols, aminophenols, methoxyphenols, aromatic amines, and ascorbic acid [19]. They have a great biotechnological potential in diverse fields of industrial application, including effluent detoxification, kraft pulp and dye bleaching, polymer synthesis, bioremediation of contaminated soils, baking, wine and beverage stabilization, the manufacture of anticancer drugs, and recently, in nanobiotechnology as part of biosensors for immunoassays [2, 16]. Production of laccases by basidiomycetes has been broadly studied as they secrete enzymes and grow on cheap substrates. Furthermore, more than one laccase isoenzyme, either constitutive or inducible, has been detected in most white-rot fungi [19]. However, as constitutive extracellular laccases from basidiomycetes are produced only in small amounts, the use of laccases for industrial applications has been limited by low process productivities and, as a consequence, high enzyme costs [12, 22]. Enhancing laccase production through the use of inducers and different nutritional conditions has been reported [6, 11, 25, 28]. Particularly, laccase production is influenced by carbon and nitrogen concentrations in culture media [3, 6, 20, 21, 26]. The use of enzyme inducers has also been investigated as they not only influence the type of isoenzymes produced but also increase volumetric activity. Much attention has been paid to the chemical induction of laccases by the addition of aromatic or phenolic compounds [12, 18], copper [15, 23], lignin [7], and ethanol [17], among others. However, the use of a combination of inducers for increasing laccase production remains almost unexplored [13].
Despite the large number of reports dealing with factors affecting laccase production by basidiomycetes, the effects of such factors on mycelial growth have been generally neglected. This is particularly important when inducers are added to the cultures, as most of them are toxic to the producing strains. Although the specific production (U/mg biomass) is frequently enhanced, enzyme titers and process productivities remain low as mycelial growth is severely affected [28].
The purpose of this work was to increase the production of laccases by Pleurotus ostreatus CP-50, which is a widely distributed cultivar for commercial mushroom production in Mexico. This strain produces two different laccases in a non-induced malt extract medium [9]. Laccases from this strain have been successfully applied for the oxidation of phenolic compounds (unpublished results). However, maximal volumetric activities were low (200 U l−1) as biomass growth was scarce (up to 2.5 g l−1). In order to increase laccase production by P. ostreatus CP50, we propose to firstly enhance fungal growth by medium improvement to increase biosynthesis potential of the culture and then evaluate the addition of inducers in order to maximize laccase production. In line with this strategy, the effect of different carbon and nitrogen sources on Pleurotus ostreatus growth was assessed, through a fractional factorial experimental design, and a medium composition improving biomass growth was selected. Then the individual and interaction effects of the culture media supplementation with copper and lignin on laccase production were evaluated.
Materials and methods
Fungal strain
P. ostreatus CP-50 was obtained from the Postgraduate College at Puebla (Colegio de Postgraduados, Unidad Puebla, Km 125.5 Carretera México-Puebla La Libertad, Cholula, 72130, Mexico). The microorganism was maintained on 2% (w/v) malt extract-agar slants at 4°C.
Chemicals
ABTS (2,2′-azinobis [3-ethylbenzo-thiazoline-6-sulfonic acid]) was from Sigma-Aldrich (St. Louis, MO). Glucose, buffer salts, and all other chemicals were from J.T. Baker (Phillipsburg, NJ). Food-grade malt extract was from Drogueria Cosmopolita, S.A. de C. V. (Mexico City, Mexico). Bacto™ Agar, Yeast extract, Bacto™ Peptone and Bacto™ Tryptone were from DIFCO (Lawrence, KS). CuSO4·5H2O was obtained from J.T. Baker (Phillipsburg, NJ) and soluble lignin (alkali, low sulfonate content) was from Sigma-Aldrich (St. Louis, MO).
Inoculum
P. ostreatus was grown on plates containing 2% (w/v) malt extract-agar at 30°C. Pre-inoculum was produced in 500-ml Erlenmeyer flasks containing 100 ml malt extract (2% w/v, pH 6). The flasks were inoculated with 2-cm2 agar plugs covered by mycelia from a 6-day-old culture previously homogenized with 10 ml of culture medium. After 4 days of incubation at 150 rev min−1 and 30°C, the mycelium was collected, washed twice with a salt solution (NaCl, 0.9% w/v), and homogenized for 10 s in a Sorvall Omni-mixer (model 17150; Ivan Sorvall Inc., Newtown, CT) with distilled water (1:3 v/v, mycelium:water). One ml of this suspension was used as inoculum for 100-ml liquid cultures in 500-ml Erlenmeyer flasks and incubated on a rotary shaker at 30°C and 150 rev min−1.
Effect of carbon and nitrogen sources on growth and laccase production by Pleurotus ostreatus CP50
Fractional factorial 26–2 experimental design used to evaluate the influence of the nature and concentration of carbon and nitrogen sources on growth and laccases production by P. ostreatus CP-50
Run . | Variables in coded levels . | Responses . | ||||||||
---|---|---|---|---|---|---|---|---|---|---|
Peptone . | Yeast extract . | Tryptone . | Xylose . | Glycerol . | Glucose . | Biomassa (mg ml−1) . | Biomassb (mg ml−1) . | Laccasea (U ml−1) . | Laccaseb (U ml−1) . | |
1 | −1 | −1 | −1 | −1 | −1 | −1 | 6.8 ± 0.60 | 7.1 | 0.6 ± 0.028 | 0.6 |
2 | +1 | −1 | −1 | −1 | +1 | −1 | 10.0 ± 0.89 | 9.8 | 0.6 ± 0.029 | 0.6 |
3 | −1 | +1 | −1 | −1 | +1 | +1 | 9.4 ± 1.54 | 9.0 | 0.6 ± 0.061 | 0.6 |
4 | +1 | +1 | −1 | −1 | −1 | +1 | 9.8 ± 1.15 | 10.6 | 1.2 ± 0.115 | 1.1 |
5 | −1 | −1 | +1 | −1 | +1 | +1 | 8.2 ± 0.88 | 7.6 | 0.7 ± 0.061 | 0.7 |
6 | +1 | −1 | +1 | −1 | −1 | +1 | 9.7 ± 1.57 | 9.2 | 0.7 ± 0.105 | 0.7 |
7 | −1 | +1 | +1 | −1 | −1 | −1 | 5.6 ± 1.37 | 5.6 | 0.7 ± 0.047 | 0.7 |
8 | +1 | +1 | +1 | −1 | +1 | −1 | 6.9 ± 0.61 | 7.3 | 1.1 ± 0.102 | 1.2 |
9 | −1 | −1 | −1 | +1 | −1 | +1 | 7.0 ± 0.22 | 7.2 | 0.7 ± 0.104 | 0.8 |
10 | +1 | −1 | −1 | +1 | +1 | +1 | 7.0 ± 0.27 | 7.0 | 1.0 ± 0.070 | 1.0 |
11 | −1 | +1 | −1 | +1 | +1 | −1 | 7.5 ± 0.18 | 7.3 | 1.0 ± 0.068 | 1.0 |
12 | +1 | +1 | −1 | +1 | −1 | −1 | 7.0 ± 0.13 | 7.1 | 1.2 ± 0.105 | 1.3 |
13 | −1 | −1 | +1 | +1 | +1 | −1 | 7.2 ± 0.38 | 7.3 | 1.0 ± 0.092 | 0.9 |
14 | +1 | −1 | +1 | +1 | −1 | −1 | 7.0 ± 0.21 | 7.1 | 1.1 ± 0.098 | 1.0 |
15 | -1 | +1 | +1 | +1 | −1 | +1 | 7.1 ± 0.27 | 7.1 | 1.0 ± 0.068 | 1.1 |
16 | +1 | +1 | +1 | +1 | +1 | +1 | 7.1 ± 0.11 | 6.9 | 1.4 ± 0.152 | 1.4 |
Run . | Variables in coded levels . | Responses . | ||||||||
---|---|---|---|---|---|---|---|---|---|---|
Peptone . | Yeast extract . | Tryptone . | Xylose . | Glycerol . | Glucose . | Biomassa (mg ml−1) . | Biomassb (mg ml−1) . | Laccasea (U ml−1) . | Laccaseb (U ml−1) . | |
1 | −1 | −1 | −1 | −1 | −1 | −1 | 6.8 ± 0.60 | 7.1 | 0.6 ± 0.028 | 0.6 |
2 | +1 | −1 | −1 | −1 | +1 | −1 | 10.0 ± 0.89 | 9.8 | 0.6 ± 0.029 | 0.6 |
3 | −1 | +1 | −1 | −1 | +1 | +1 | 9.4 ± 1.54 | 9.0 | 0.6 ± 0.061 | 0.6 |
4 | +1 | +1 | −1 | −1 | −1 | +1 | 9.8 ± 1.15 | 10.6 | 1.2 ± 0.115 | 1.1 |
5 | −1 | −1 | +1 | −1 | +1 | +1 | 8.2 ± 0.88 | 7.6 | 0.7 ± 0.061 | 0.7 |
6 | +1 | −1 | +1 | −1 | −1 | +1 | 9.7 ± 1.57 | 9.2 | 0.7 ± 0.105 | 0.7 |
7 | −1 | +1 | +1 | −1 | −1 | −1 | 5.6 ± 1.37 | 5.6 | 0.7 ± 0.047 | 0.7 |
8 | +1 | +1 | +1 | −1 | +1 | −1 | 6.9 ± 0.61 | 7.3 | 1.1 ± 0.102 | 1.2 |
9 | −1 | −1 | −1 | +1 | −1 | +1 | 7.0 ± 0.22 | 7.2 | 0.7 ± 0.104 | 0.8 |
10 | +1 | −1 | −1 | +1 | +1 | +1 | 7.0 ± 0.27 | 7.0 | 1.0 ± 0.070 | 1.0 |
11 | −1 | +1 | −1 | +1 | +1 | −1 | 7.5 ± 0.18 | 7.3 | 1.0 ± 0.068 | 1.0 |
12 | +1 | +1 | −1 | +1 | −1 | −1 | 7.0 ± 0.13 | 7.1 | 1.2 ± 0.105 | 1.3 |
13 | −1 | −1 | +1 | +1 | +1 | −1 | 7.2 ± 0.38 | 7.3 | 1.0 ± 0.092 | 0.9 |
14 | +1 | −1 | +1 | +1 | −1 | −1 | 7.0 ± 0.21 | 7.1 | 1.1 ± 0.098 | 1.0 |
15 | -1 | +1 | +1 | +1 | −1 | +1 | 7.1 ± 0.27 | 7.1 | 1.0 ± 0.068 | 1.1 |
16 | +1 | +1 | +1 | +1 | +1 | +1 | 7.1 ± 0.11 | 6.9 | 1.4 ± 0.152 | 1.4 |
aExperimental data
bEstimated data
Fractional factorial 26–2 experimental design used to evaluate the influence of the nature and concentration of carbon and nitrogen sources on growth and laccases production by P. ostreatus CP-50
Run . | Variables in coded levels . | Responses . | ||||||||
---|---|---|---|---|---|---|---|---|---|---|
Peptone . | Yeast extract . | Tryptone . | Xylose . | Glycerol . | Glucose . | Biomassa (mg ml−1) . | Biomassb (mg ml−1) . | Laccasea (U ml−1) . | Laccaseb (U ml−1) . | |
1 | −1 | −1 | −1 | −1 | −1 | −1 | 6.8 ± 0.60 | 7.1 | 0.6 ± 0.028 | 0.6 |
2 | +1 | −1 | −1 | −1 | +1 | −1 | 10.0 ± 0.89 | 9.8 | 0.6 ± 0.029 | 0.6 |
3 | −1 | +1 | −1 | −1 | +1 | +1 | 9.4 ± 1.54 | 9.0 | 0.6 ± 0.061 | 0.6 |
4 | +1 | +1 | −1 | −1 | −1 | +1 | 9.8 ± 1.15 | 10.6 | 1.2 ± 0.115 | 1.1 |
5 | −1 | −1 | +1 | −1 | +1 | +1 | 8.2 ± 0.88 | 7.6 | 0.7 ± 0.061 | 0.7 |
6 | +1 | −1 | +1 | −1 | −1 | +1 | 9.7 ± 1.57 | 9.2 | 0.7 ± 0.105 | 0.7 |
7 | −1 | +1 | +1 | −1 | −1 | −1 | 5.6 ± 1.37 | 5.6 | 0.7 ± 0.047 | 0.7 |
8 | +1 | +1 | +1 | −1 | +1 | −1 | 6.9 ± 0.61 | 7.3 | 1.1 ± 0.102 | 1.2 |
9 | −1 | −1 | −1 | +1 | −1 | +1 | 7.0 ± 0.22 | 7.2 | 0.7 ± 0.104 | 0.8 |
10 | +1 | −1 | −1 | +1 | +1 | +1 | 7.0 ± 0.27 | 7.0 | 1.0 ± 0.070 | 1.0 |
11 | −1 | +1 | −1 | +1 | +1 | −1 | 7.5 ± 0.18 | 7.3 | 1.0 ± 0.068 | 1.0 |
12 | +1 | +1 | −1 | +1 | −1 | −1 | 7.0 ± 0.13 | 7.1 | 1.2 ± 0.105 | 1.3 |
13 | −1 | −1 | +1 | +1 | +1 | −1 | 7.2 ± 0.38 | 7.3 | 1.0 ± 0.092 | 0.9 |
14 | +1 | −1 | +1 | +1 | −1 | −1 | 7.0 ± 0.21 | 7.1 | 1.1 ± 0.098 | 1.0 |
15 | -1 | +1 | +1 | +1 | −1 | +1 | 7.1 ± 0.27 | 7.1 | 1.0 ± 0.068 | 1.1 |
16 | +1 | +1 | +1 | +1 | +1 | +1 | 7.1 ± 0.11 | 6.9 | 1.4 ± 0.152 | 1.4 |
Run . | Variables in coded levels . | Responses . | ||||||||
---|---|---|---|---|---|---|---|---|---|---|
Peptone . | Yeast extract . | Tryptone . | Xylose . | Glycerol . | Glucose . | Biomassa (mg ml−1) . | Biomassb (mg ml−1) . | Laccasea (U ml−1) . | Laccaseb (U ml−1) . | |
1 | −1 | −1 | −1 | −1 | −1 | −1 | 6.8 ± 0.60 | 7.1 | 0.6 ± 0.028 | 0.6 |
2 | +1 | −1 | −1 | −1 | +1 | −1 | 10.0 ± 0.89 | 9.8 | 0.6 ± 0.029 | 0.6 |
3 | −1 | +1 | −1 | −1 | +1 | +1 | 9.4 ± 1.54 | 9.0 | 0.6 ± 0.061 | 0.6 |
4 | +1 | +1 | −1 | −1 | −1 | +1 | 9.8 ± 1.15 | 10.6 | 1.2 ± 0.115 | 1.1 |
5 | −1 | −1 | +1 | −1 | +1 | +1 | 8.2 ± 0.88 | 7.6 | 0.7 ± 0.061 | 0.7 |
6 | +1 | −1 | +1 | −1 | −1 | +1 | 9.7 ± 1.57 | 9.2 | 0.7 ± 0.105 | 0.7 |
7 | −1 | +1 | +1 | −1 | −1 | −1 | 5.6 ± 1.37 | 5.6 | 0.7 ± 0.047 | 0.7 |
8 | +1 | +1 | +1 | −1 | +1 | −1 | 6.9 ± 0.61 | 7.3 | 1.1 ± 0.102 | 1.2 |
9 | −1 | −1 | −1 | +1 | −1 | +1 | 7.0 ± 0.22 | 7.2 | 0.7 ± 0.104 | 0.8 |
10 | +1 | −1 | −1 | +1 | +1 | +1 | 7.0 ± 0.27 | 7.0 | 1.0 ± 0.070 | 1.0 |
11 | −1 | +1 | −1 | +1 | +1 | −1 | 7.5 ± 0.18 | 7.3 | 1.0 ± 0.068 | 1.0 |
12 | +1 | +1 | −1 | +1 | −1 | −1 | 7.0 ± 0.13 | 7.1 | 1.2 ± 0.105 | 1.3 |
13 | −1 | −1 | +1 | +1 | +1 | −1 | 7.2 ± 0.38 | 7.3 | 1.0 ± 0.092 | 0.9 |
14 | +1 | −1 | +1 | +1 | −1 | −1 | 7.0 ± 0.21 | 7.1 | 1.1 ± 0.098 | 1.0 |
15 | -1 | +1 | +1 | +1 | −1 | +1 | 7.1 ± 0.27 | 7.1 | 1.0 ± 0.068 | 1.1 |
16 | +1 | +1 | +1 | +1 | +1 | +1 | 7.1 ± 0.11 | 6.9 | 1.4 ± 0.152 | 1.4 |
aExperimental data
bEstimated data
Effect of copper and lignin on growth and laccase production by Pleurotus ostreatus
Medium 4 (Table 1) was selected as high biomass concentration was obtained and laccase production was significantly improved. Lignin (0.5 g l−1) and CuSO4 (0.5 mM) were added individually or combined at the middle of the growth phase (60 h).
Analytical techniques
Mycelial biomass was measured as reported before [9]. ABTS (2,2′-azinobis [3-ethylbenzothia-zoline-6-sulfonic acid]) was used as laccase substrate (1 mM in acetate buffer pH 3.6). The enzymatic reaction was monitored in a Beckman DU 650 spectrophotometer at 436 nm (ε 436 = 29,300 M−1 cm−1). One enzyme unit (U) was defined as the amount of enzyme catalyzing the production of 1 μmol of oxidized product per minute.
Statistical analysis
All the experiments were independently performed by triplicate and differences among treatments were evaluated by ANOVA analysis (p = 0.05) using DESIGN-EXPERT, version 5.0.7 (Stat-Ease Inc., Minneapolis, MN).
Results and discussion
The effect of medium composition (carbon and nitrogen sources) on maximal P. ostreatus growth and laccase volumetric activity was evaluated through a fractional factorial 26–2 and the results are summarized in Table 1.
Effects of carbon and nitrogen sources on P. ostreatus growth
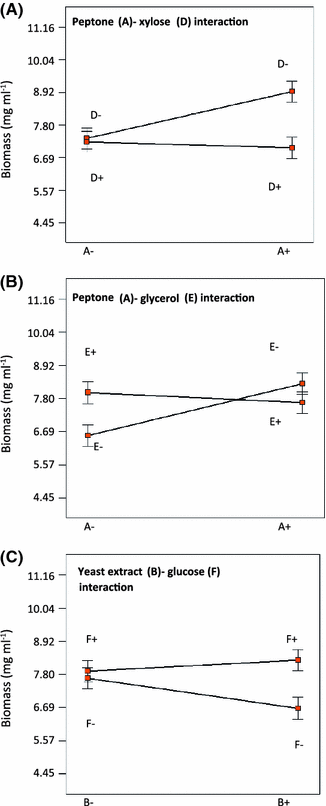
Relevant factor interactions (p < 0.05) affecting growth of P. ostreatus CP-50. a Peptone–xylose interaction. b Peptone–glycerol interaction. c Yeast extract–glucose interaction
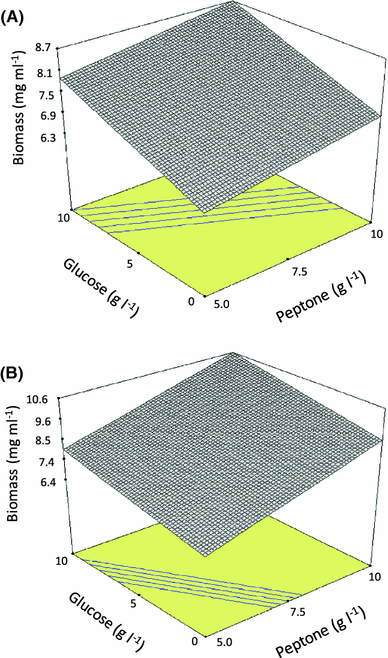
Response surface representing maximal biomass concentration versus glucose and peptone concentration. Media composition (g l−1): a tryptone 5, yeast extract 10, glycerol 0, xylose 10; b tryptone 5, yeast extract 10, glycerol 0, xylose 0
Effects of carbon and nitrogen sources on laccase production by P. ostreatus
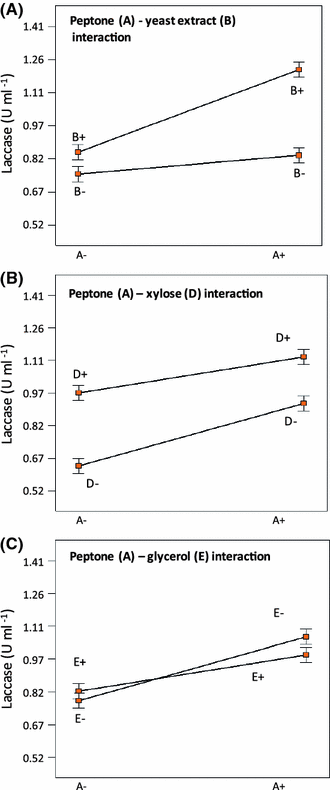
Relevant factor interactions (p < 0.05) affecting laccase production by P. ostreatus CP-50. a Peptone–yeast extract interaction. b Peptone–xylose interaction. c Peptone–glycerol interaction
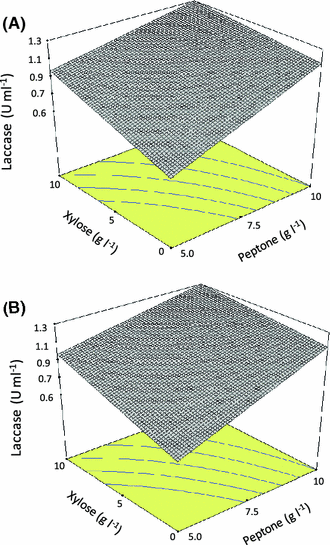
Response surface representing maximal laccase volumetric activity versus xylose and peptone concentration. Media composition (g l−1): a tryptone 5, yeast extract 10, glycerol 0, glucose 0; b tryptone 5, yeast extract 10, glycerol 0, glucose 10
The relevant literature shows that the best carbon sources for laccase production by Trametes pubescens are cellobiose, glucose, and glycerol, while lactose and cellulose are poor carbon sources for laccase production [11]. Prasad et al. [25] showed that increasing glucose concentration (from 10 to 30 g l−1) resulted in an enhanced laccase production (50% increase) by P. ostreatus 1804. Mikiashvili et al. [20, 21] reported that polysaccharides (Avicel and CMC) are poor substrates for laccase production by Trametes versicolor, while easily metabolizable sugars (such as glucose and cellobiose) supported high biomass and laccase activities. Our results showed that the use of xylose as the only carbon source yields an increased specific laccase production (U/mg biomass) but in detriment of fungal growth. On the other hand, the use of glucose instead has the opposite effect.
Although the type and concentration of nitrogen sources strongly affects fungal growth and metabolite production, it is generally accepted that organic nitrogen sources enhance growth yields of macrofungi; however, the effect of organic nitrogen source on laccase production depends on the microorganism [8, 14]. Laccase activities of both P. ostreatus and Lentinus edodes were greatly stimulated in a peptone nitrogen-based medium [14]. Galhaup et al. [11] showed that the use of peptone, tryptone, and yeast extract increases laccase production by Trametes pubescens on a simple glucose-based medium with 2 mM of copper, although growth of the microorganism was not affected. In the case of P. ostreatus 1804, yeast extract has shown a positive effect on laccase production when its concentration does not exceed 15 g l−1 [25]. Peptone, followed by casein hydrolysate, was the best nitrogen source for biomass growth and laccases production by two strains of P. ostreatus [21]. Recently, it has been reported that casein is the best nitrogen source for laccase production by Pleurotus sajor-caju when compared to peptone, beer yeast, and soy extract [3].
In the above-mentioned literature, most of the studies only evaluate the carbon/nitrogen sources effect on laccase production with practically no discussion about the effects on fungal growth. Indeed, to our knowledge, no studies about the individual effects, and their interactions, among different carbon and nitrogen sources on growth and laccases production by any microorganism, have been previously reported.
As can be expected, the medium composition, which allowed to significantly increase the specific laccase production (U/mg biomass), is not the best medium for fungal growth. Our results showed that medium 16 allowed the maximal laccase volumetric activity of 1.4 U ml−1 and 7 g l−1 of biomass, which is 30% lower than the maximal biomass achieved with other media compositions (i.e., 9.8 g l−1 with medium 4). It is worth noting that none of the media suppressed laccase production. However, the best compromise to significantly (p = 0.05) increase biomass growth, without a significant (p = 0.05) difference in laccase production, was medium 4, in which 9.8 g l−1 and 1.2 U ml−1 were obtained, respectively. The study of fungal growth and laccase production by P. ostreatus in the control medium (malt extract 2%) and medium 4 was then undertaken.
As the initial objective of this work was to enhance the growth of P. ostreatus without a repression of laccase production, carbon (glucose, xylose, and glycerol) and nitrogen sources (peptone, yeast extract, and tryptone) were selected on the basis of the literature survey (presented earlier in this section) of the effect on medium composition on laccase production by basidiomycetes. The basal medium contains only malt extract (2%), and maximal fungal growth (2.5 g l−1) and laccase volumetric activity (0.5 U ml−1) were very low. In such conditions it is likely that fungal growth was principally limited by nitrogen availability. To overcome this fungal growth limitation, the experimental design focused on increasing nitrogen content (all the nitrogen sources are present in all the tested media) in relation to carbon content. The effect of carbon and nitrogen source on fungal growth and laccase production was assessed through a fractional factorial experimental design 26–2.
The experimental design allowed to obtain a significant increase of fungal growth as well as laccase production. Under our conditions, all the media tested supported more than 5 g l−1 of biomass production after 96 h of incubation, representing an increase of 100% of the biomass concentration in comparison with the basal medium. Furthermore, medium 4 supported a maximal biomass concentration of 9.8 g l−1 together with 1.2 U ml−1 of laccase volumetric activity after 96 h of cultivation, yielding 370 and 250% for fungal growth and laccase production, respectively, compared to the basal medium. This is an important issue as maximal laccase production by basidiomycetes usually takes much longer cultivation periods on the order of hundreds of hours [27] and yielding low process productivities.
Kinetic characterization of growth and laccase production by P. ostreatus CP50
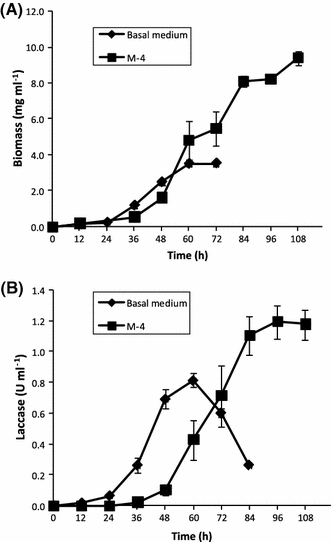
Kinetics of growth and laccase volumetric activity in P. ostreatus CP-50 cultures as a function of the medium composition
Based on our results, medium 4 was selected in order to study the influence of the addition of copper and/or lignin on the laccase production by P. ostreatus. Laccase production by P. ostreatus CP-50 is growth-associated when cultured in either medium 4 and basal medium and no differences were found in their specific growth rates (0.1 h−1). Comparing our results with previous reports, it has been shown that laccase production by P. ostreatus ATCC 32783 is growth-associated, with a specific growth rate of 0.02 h−1, in glucose-yeast extract based medium supplemented with copper [27]. Differences in the specific growth rate could be due to the timing of the addition of copper. In the case of P. ostreatus ATCC 32783, 1.5 mM copper was added at the beginning of the culture and it is well known that copper can be toxic for microorganisms [1]. Nevertheless, maximal growth (8 g l−1) and laccase volumetric activity (1.2 U ml−1) were almost identical to that obtained in this work; however, an incubation time of 500 h was required.
Our results showed that maximal laccase volumetric activity was reached before fungal growth stopped. However, while no significant decrease on laccase activity was found in cultures carried out in medium 4, this was not the case for the basal medium, where 50% of the activity was lost. Production of proteases by P. ostreatus has been reported at the later stages of fungal growth [24]. Differences in the stability of laccase activity in both cultures media could be explained by the high protein content of medium 4, which can compete for proteolysis with laccase.
Furthermore, when P. ostreatus was cultured in medium 4, only a third of the specific productivity of that obtained in the basal medium was achieved and no significant influence of the medium composition on the specific growth rate (0.1 h−1) was found. However, the onset of laccase production on medium 4 showed a delay of 24 h, as compared with that observed in the basal medium. As both media contain malt extract, it is likely that protein supplementation (as sole nitrogen sources) of basal media induces higher proteolytic activities. Therefore, measured laccase activities should reflect the difference between synthesis and degradation rates. However, proteolytic activity was not assessed during cultures.
Effect of copper and lignin on laccase production by P. ostreatus CP50
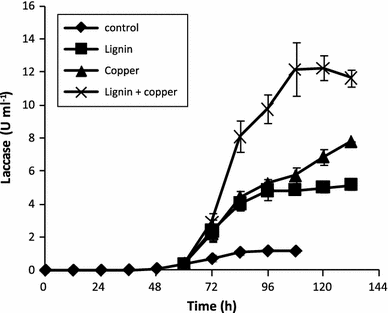
Laccase production during the cultures of P. ostreatus CP-50 where copper and/or lignin were added as inducers at the middle of the growth phase (60 h)
Overall, laccase volumetric activity was significantly enhanced by the simultaneous use of copper and lignin in an optimized culture media for P. ostreatus CP-50 growth. For instance, in malt extract medium, maximal biomass growth and laccase activity were 2.5 g l−1 and 0.2 U ml−1 after 72 h of cultivation, respectively; while for the induced system, the values were 10 g l−1 and 12 U ml−1 after 108 h, respectively. An overall productivity of 1.1 U g biomass−1 h−1 was obtained in malt extract cultures, while a tenfold increase (11.1 U g biomass−1 h−1) was obtained in the induced cultures.
Based on the fungal growth kinetics on medium 4, the influence of copper and/or lignin supplementation on laccase production by P. ostreatus CP-50 was evaluated. Copper induction of laccases enzymes has been previously shown in Trametes versicolor [5], Pleurotus ostreatus [23], Trametes pubescens [10], and Botryosphaeria rhodina [6] cultures. Such studies showed that regulation of the synthesis of different laccases isoenzymes by copper was at the level of gene transcription. The use of lignin and lignin sulphonate for increased laccase production by Trametes versicolor has been recently reported [29]. However, very little has been done on the simultaneous use of two inducers on laccase production. Indeed, to the authors' knowledge, only Minussi et al. [22] have reported that significant enhancement of laccase production by Trametes versicolor can be obtained with the simultaneous addition of Cu and 2,5-xylidine.
Our results showed that maximal laccase volumetric activity was reached before fungal growth stopped. Thus, under our conditions, laccase production was growth-associated. In light of this, media design to improve fungal growth and further addition of inducer to increase laccase production was adequate. Previous results showed that the addition of copper at the beginning of culture drastically reduced growth and laccase production by P. ostreatus CP-50 (results not shown). However, laccase production by Pleurotus ostreatus CP-50 was significantly enhanced when lignin (threefold) or Cu (sevenfold) were added at the middle of the exponential growth phase. Furthermore, laccase volumetric activity was influenced by the type of the inducer. For instance, laccase production in lignin-added cultures was strongly stimulated during the first 36 h after induction, and no significant differences in volumetric activity were found if compared with Cu-induced cultures. After that, no further increase on enzyme activity (4.5 U ml−1) was observed. However, in Cu-induced cultures, laccase production was stimulated until 132 h of cultivation (62 h after Cu addition) when 7.0 U ml−1 were obtained.
When Cu and lignin were added simultaneously, laccase volumetric activity was strongly enhanced reaching its maximum (12.2 U ml−1) only 48 h after induction (108 h of cultivation). It is worth noting that by analyzing the laccase increase relative to the sum of laccase volumetric activities of individually induced cultures (at 108 h of cultivation), the simultaneous addition of Cu and lignin exhibited a synergistic effect, resulting in a significant increase (30%) of laccase productivity by P. ostreatus CP-50. This was obtained without a formal optimization of the inducers concentration and/or the time of addition.
Acknowledgments
This study was financed by DGAPA-UNAM (IN217909). We thank M. Caro, V. Albiter and M. García for their technical assistance. The authors also thank J.M. Hurtado for computer support.