-
PDF
- Split View
-
Views
-
Cite
Cite
Francisca Lúcia Lima, Maria Auxiliadora Roque de Carvalho, Ana Carolina Morais Apolônio, Marcelo Porto Bemquerer, Marcelo Matos Santoro, Jamil Silvano Oliveira, Celuta Sales Alviano, Luiz de Macêdo Farias, Actinomycetemcomitin: a new bacteriocin produced by Aggregatibacter (Actinobacillus) actinomycetemcomitans, Journal of Industrial Microbiology and Biotechnology, Volume 35, Issue 2, 1 February 2008, Pages 103–110, https://doi.org/10.1007/s10295-007-0271-z
- Share Icon Share
Abstract
Aggregatibacter (Actinobacillus) actinomycetemcomitans P7–20 strain isolated from a periodontally diseased patient has produced a bacteriocin (named as actinomycetemcomitin) that is active against Peptostreptococcus anaerobius ATCC 27337. Actinomycetemcomitin was produced during exponential and stationary growth phases, and its amount decreased until it disappeared during the decline growth phase. It was purified by ammonium sulphate precipitation (30–60% saturation), and further by FPLC (mono-Q ionic exchange and Phenyl Superose hydrophobic interaction) and HPLC (C-18 reversed-phase). This bacteriocin loses its activity after incubation at a pH below 7.0 or above 8.0, following heating for 30 min at 45°C, and after treatment with proteolytic enzymes such as trypsin, α-chymotrypsin, and papain. Actinomycetemcomitin has a molecular mass of 20.3 KDa and it represents a new bacteriocin from A. actinomycetemcomitans.
Introduction
Aggregatibacter (formerly Actinobacillus) actinomycetemcomitans is a microorganism associated with various diseases, particularly early-onset periodontitis [27, 35, 40, 33]. This microorganism produces a number of virulence factors, including LPS, adhesins, leukotoxin, colagenase and invasins [11, 36].
The relationship between A. actinomycetemcomitans and other oral microorganisms is complex and currently of much interest [36, 37, 40]. Positive associations between A. actinomycetemcomitans and oral microbiota have been reported, including a close relationship with species of Capnocytophaga and Eikenella corrodens [2, 31]. Also, a negative association has been reported with A. actinomycetemcomitans and Streptococcus sanguinis [19, 20, 25, 37].
The ability of A. actinomycetemcomitans to produce a bacteriocin (actinobacillin) was established by Hammond et al. [16], and this inhibitory factor was active against S. sanguinis strains, Streptococcus uberis FDC 1 and Actinomyces viscosus T14, as well as against other A. actinomycetemcomitans strains. It is expected that this substance would be involved in the relationship between A. actinomycetemcomitans and other oral microorganisms.
To study the bactericin action and its characteristics, one must first understand its production, which may be influenced by methodological factors such as medium composition, incubation atmosphere, pH and temperature [4, 23]. The production is also influenced by the growth phase of the microorganism [1, 5, 7, 29]. Although for some bacteria, such as for Enterococcus faecium, the production of bacteriocin has been detected during all growth phases [21, 34], for other microorganisms, this production has been detected just in a specific growth phase, such as for A. actinomycetemcomitans [38], Lactobacillus acidophilus IBB 801 [41] and Bacillus thuringiensis BMG 1.7 [6], in which the production occurred at the stationary phase.
The bacteriocin previously reported by Hammond et al. [16] was purified and its molecular mass was determined to be 120 KDa, with two subunits of 50 and 70 KDa [38]. It has also been shown that A. Actinomycetemcomitans strains recovered from marmosets (Callithrix penicillata) produce antagonistic substances that have the properties of bacteriocins and haemolysins [28].
Recently, Lima et al. [22] observed in vitro a wide spectrum of activity against Gram-positive and Gram-negative bacteria and suggested that more than one bacteriocin is produced by A. actinomycetemcomitans. To confirm this hypothesis, the aim of the present investigation was to purify a novel bacteriocin from a strain of A. actinomycetemcomitans isolated from human periodontal disease.
Materials and methods
Bacterial strains and culture media
A. actinomycetemcomitans P7.20, isolated from the periodontal pocket of a patient with progressive rapid periodontitis, was used to obtain the bacteriocin for this study. This strain, previously screened for antagonistic activity, showed a wide spectrum of activity against both Gram-negative and -positive bacteria, and the antagonistic substance presented a proteic nature [22]. Peptostreptococcus anaerobius ATCC 27337, Bacteroides merdae ATCC 43184, L. casei ATCC 7469, F. nucleatum P12.2, M5.7, P. gingivalis ATCC 33277, S. sanguinis ATCC 10557, and S. uberis ATCC 9927 were used as indicator strains. The culture was routinely stored at −86°C in tryptic-soy broth media (Difco) supplemented with 0.5% yeast extract and 10% glycerol (TSB-S), pH 7.2. TSB-S supplemented with 0.5% yeast extract, 0.1% L-cystine (Difco), and 0.04% sodium bicarbonate (Difco), pH 8.0 was used to produce bacteriocin. The assay to evaluate the bacteriocin-like activity was determined via the double-layer method using tryptic-soy agar media (Difco) supplemented as cited above, and soft TSB agar (0.7%) supplemented with 0.5% yeast extract (Difco), 0.005% hemin (Difco) and 0.001% menadione (TSA-S; Difco).
Bacteriocin-like activity assay and protein determinations
Bacteriocin activity was assayed by the agar diffusion method, and the bacteriocin titer was determined by the serial dilution method, as previously described [3, 26]. Aliquots of filtrate extracts were serially twofold diluted with sterilized ultra-pure water in microtitration plates (Micrower Plate 96F). Aliquots of filtrate extracts (20, 10 or 5 μl, depending on the purification step) were spotted onto the surface of the media. After absorption of the extract, the Petri dishes were overlaid with 4 ml TSB-S soft agar (0.7%) that had been inoculated with 0.2 ml of a 24-h TSB-S culture of P. anaerobius ATCC 27337. The plates were incubated at 37°C for 24–48 h in an anaerobic chamber (85% N2, 10% H2 and 5% CO2, Forma Scientific Co., USA), and the titer was defined as 2n, where n is the reciprocal of the highest dilution that resulted in inhibition of the indicator strain. Thus, the arbitrary unit (AU) of antibacterial activity per milliliter (AU ml−1) was defined as 2n × 1000 μl × ν μl−1, where ν is the volume of bacteriocin used in the test. The protein measurements were determined according to Lowry et al. [24]. Bovine serum albumin (BSA) was used as a standard.
Kinetics of bacteriocin production
TSB-S medium (300 ml) was inoculated with a 24 h culture of A. actinomycetemcomitans P7.20 strain (106 CFU/ml final concentration) and incubated in anaerobic medium up to 96 h at 37°C. During this period, aliquots were taken up to measure the absorbance at 600 nm. The bacteriocin-like activity was measured at 18, 24, 36, 48, 72 and 96 h of growth. At each time point, the cultures were centrifuged at 2,500 g for 10 min (Sorvall RC5C Instruments Du Pont), and the supernatant was filtered (0.22 μm, Millipore). A. actinomycetemcomitans cells (sediment) recovered after centrifugation were washed three times in Tris–HCl buffer, pH 8.0 (Sigma), sonicated (Branson Sonifier 450) for 10 min, with 20 cycles of 30 s at 50 W in an ice bath, and ultra-centrifuged for 1 h to obtain the intracellular fraction. The supernatants and intracellular fractions were lyophilized (Freeze Dry System-Labconco), and then diluted in 0.01 M Tris–HCl to achieve 1 mg ml−1. Subsequently, 50 μl were spotted onto TSA-S and assayed as described above.
Production of the crude extract
A. actinomycetemcomitans P7.20 strain was cultured in 50 ml TSB-S for 24 h at 37°C in an anaerobic chamber. Next, the culture was inoculated in 950 ml TSB-S, and incubated for a further 24 h under the same conditions. The culture was centrifuged at 2,500 g for 20 min at 4°C (Sorvall RC5C Instruments Du Pont), and the cells were washed thrice with 0.01 M Tris–HCl buffer, pH 8.0. After washing, the cells were suspended in 20 ml of the same buffer and sonicated (Branson Sonifier 450) for 10 min, with 20 cycles of 30 s at 50 W in an ice bath. Cellular disruption was verified under the microscope. The extract was centrifuged at 100,000 g for 60 min at 4°C, and the supernatant (crude extract, 8 mg ml−1) was maintained at −40°C.
Bacteriocin purification
The crude extract (20 ml at 8 mg ml−1) was precipitated with ammonium sulphate (Labsynth) at three increasing concentration ranges (0–30, 30–60 and 60–90%), followed by an ion-exchange chromatography on a fast protein liquid chromatography (FPLC) system equipped with a Mono-Q HR 5/5 (Pharmacia) column, equilibrated with Tris–HCl buffer (pH 8.0, 0.01 M), eluted with the same buffer, followed by a gradient from 0 to 0.06 M to elute the active fraction, and then raised to 1.0 M NaCl in Tris–HCl buffer, pH 8.0. Aliquots of 1.5 ml were collected, lyophilized and assayed for antagonistic activity. The active aliquots were individually subjected to hydrophobic interaction chromatography on a Phenyl Superose HR 5/5 (Pharmacia) column, equilibrated with 1.7 M ammonium sulphate in 0.01 M phosphate buffer, pH 8.0. Separation was performed by a linear gradient up to 0.01 M phosphate buffer, pH 8.0. The final step was a reversed-phase chromatography conducted in an HPLC system equipped with a C18 (Pharmacia) column (300 × 4.6 mm). Mobile phases A and B were 0.1% aqueous trifluoroacetic acid (TFA) solution and 50% aqueous acetonitrile solution (containing 0.1% TFA), respectively. The aliquots were collected, concentrated and assayed as previously described. Elution profiles were monitored at 280 nm using both FPLC and HPLC analysis. The active fractions were freeze-dried.
Molecular mass determination
ESI-Q-TOF mass spectrometry analyses were carried out using a Q-TOF MicroTM (Micromass, Manchester, UK) equipped with an electrospray ionization source operated in positive or negative ion mode. The capillary voltage was 3.0–3.5 kV and sample cone voltages were 40–60 V. The samples were diluted in 50% aqueous acetonitrile containing 0·1% TFA and introduced by using a syringe pump with flow rates of 5–10 μl min−1 [32]. Data were analysed with MassLynx® 3·5 software (Micromass).
Determination of amino acid sequences
Peptides that failed to yield a sequence when subjected to Edman degradation were unblocked by treatment with pyroglutamate aminopeptidase in 50 mM Na phosphate buffer, pH 7.0, containing 10 mM dithiothreitol and 10 mM ethylenediaminetetraacetic acid (EDTA) at 50°C for 6 h.
The N-terminal amino acid sequences of the S-pyridyl-ethylated intact proteins (2–10 nmol) and the peptides derived from them by enzymatic digestions were determined by Edman degradation using a Shimadzu PPSQ-21A automated protein sequencer.
Sequence comparisons
The amino acid sequences of the various peptides/proteins were compared with the sequences of other related proteins in the SWISS-PROT/TREMBL databases using the FASTA 3 and BLAST programs.
Sodium dodecyl sulphate-polyacrylamide gel electrophoresis
Bacteriocinogenic active fractions from each step of purification were submitted to sodium dodecyl suphate-polyacrilamide gel electrophoresis (SDS-PAGE, at 12%), with the addition of 5% by volume 2-mercaptoethanol, using two standard molecular weight markers, one ranging from 14.3 to 200 kDa (Gibco) and another from 6.5 to 66 kDa (Sigma). The gel was silver-stained according to the protocol described by Tuñon and Johansson [39].
Effect of different treatments on the bacteriocin activity
Aliquots of 50 μl (2.25 mg ml−1) of the partially purified bacteriocin were added to 450 μl of each filter-sterilized buffer and subjected to heat treatment and enzyme digestions, and adjusted to various pH values [12]. After the different treatments, the inhibitory activities of the bacteriocin samples were assayed as described above. Non-treated bacteriocin, buffers alone and enzyme solutions alone were used as controls.
Heat treatments: The effect of temperature was evaluated by incubation at 37, 45, 60, 80 and 100°C for 20 min up to 24 h in a water bath. The treatments were performed in 10 mM Tris–HCl buffer, pH 8.0.
Enzymes: Incubations with proteolytic enzymes were performed for 2 h at 37°C. The enzyme solutions (1 mg ml−1) used were: protease type VI, protease type XXVIII, α-chymotrypsin and papain in 50 mM phosphate buffer (pH 7.0), trypsin in 20 mM Tris–HCl (pH 8.0) and proteinase K in 20 mM borate buffer (pH 7.2). Before use, all enzymes (Sigma) were sterilized through a 0.22 μm filter.
pH variations: The effect of pH on the bacteriocin activity was tested in the following buffers: 10 mM KCl–HCl (2.0), 10 mM glycine–HCl (3.0), 10 mM citrate (4.0), 10 mM phosphate–citrate (5.0, 6.0), 10 mM Tris–HCl (7.0, 8.0), and 10 mM carbonate–bicarbonate (9.0, 10.0). Each buffer was filter-sterilized before being used. Incubation was performed at 37°C for 2 h.
Results
Aggregatibacter (formerly Actinobacillus) actinomycetemcomitans P7.20, which was selected to obtain and purify a bacteriocin, was able, under anaerobiosis, to antagonise P. anaerobius ATCC 27337, B. merdae ATCC 43184, L. casei ATCC 7469, F. nucleatum P12.2, M 5.7, P. gingivalis ATCC 33277 and S. uberis ATCC 9927, but not S. sanguinis ATCC 10557.
In culture media selected for production of the bacteriocin (TSB-S, pH 8.0), the A. actinomycetemcomitans P7.20 showed a lag phase, which extended over 8 h and the maximum growth occurred at 18 h. Bacteriocin activity began to be detected after 18 h of culture, at the end of the exponential phase, and it reached the highest activity level at 24 h of growth, during the stationary phase. A small antagonistic activity could still be observed after 72 h of culture. It was possible to detect a bacteriocin activity in the crude extract from the intracellular, but not from the extracellular, fraction following the methodology used in this study.
The fraction obtained at 30–60% saturation of ammonium sulphate (fraction I, Table 1) was positive for bacteriocin-like activity. This fraction was submitted to anion exchange to determine the elution profile. Bacteriocin activity was observed at the fraction eluted with 0.06 M NaCl (fraction II, Table 1).
Purification of the bacteriocin produced by A. actinomycetemcomitants P7–20 strain
Fraction . | Volume (ml) . | Total protein (mg/ml) . | Total activity (AU/ml) . | Specific activity (AU/mg) . | Yield (%) . | Indicator samples of antagonism . |
---|---|---|---|---|---|---|
Crude extract | 20 | 8 | 50 | 6.25 | 1 | P. anaerobius ATCC 27337, B. merdae ATCC 43184, L. casei ATCC 7469, F. nucleatum P12.2, M 5.7, P. gingivalis ATCC 33277, and S. uberis ATCC 9927 |
Precipitation with ammonium sulphate (30–60%; fraction I) | 5 | 4.5 | 75 | 16.65 | 1.5 | P. anaerobius ATCC 27337, P. gingivalis ATCC 33277 |
Ion-exchange (fraction II) | 5 | 2 | 100 | 50 | 2.0 | P. anaerobius ATCC 27337 |
Hydrophobic interaction (53% and 39; fraction III) | 2 | 0.5 | 180 | 90 | 3.6 | P. anaerobius ATCC 27337 |
RP-HPLC (fraction IV) | 1 | 0.01 | 440 | 44,000 | 8.8 | P. anaerobius ATCC 27337 |
Fraction . | Volume (ml) . | Total protein (mg/ml) . | Total activity (AU/ml) . | Specific activity (AU/mg) . | Yield (%) . | Indicator samples of antagonism . |
---|---|---|---|---|---|---|
Crude extract | 20 | 8 | 50 | 6.25 | 1 | P. anaerobius ATCC 27337, B. merdae ATCC 43184, L. casei ATCC 7469, F. nucleatum P12.2, M 5.7, P. gingivalis ATCC 33277, and S. uberis ATCC 9927 |
Precipitation with ammonium sulphate (30–60%; fraction I) | 5 | 4.5 | 75 | 16.65 | 1.5 | P. anaerobius ATCC 27337, P. gingivalis ATCC 33277 |
Ion-exchange (fraction II) | 5 | 2 | 100 | 50 | 2.0 | P. anaerobius ATCC 27337 |
Hydrophobic interaction (53% and 39; fraction III) | 2 | 0.5 | 180 | 90 | 3.6 | P. anaerobius ATCC 27337 |
RP-HPLC (fraction IV) | 1 | 0.01 | 440 | 44,000 | 8.8 | P. anaerobius ATCC 27337 |
Purification of the bacteriocin produced by A. actinomycetemcomitants P7–20 strain
Fraction . | Volume (ml) . | Total protein (mg/ml) . | Total activity (AU/ml) . | Specific activity (AU/mg) . | Yield (%) . | Indicator samples of antagonism . |
---|---|---|---|---|---|---|
Crude extract | 20 | 8 | 50 | 6.25 | 1 | P. anaerobius ATCC 27337, B. merdae ATCC 43184, L. casei ATCC 7469, F. nucleatum P12.2, M 5.7, P. gingivalis ATCC 33277, and S. uberis ATCC 9927 |
Precipitation with ammonium sulphate (30–60%; fraction I) | 5 | 4.5 | 75 | 16.65 | 1.5 | P. anaerobius ATCC 27337, P. gingivalis ATCC 33277 |
Ion-exchange (fraction II) | 5 | 2 | 100 | 50 | 2.0 | P. anaerobius ATCC 27337 |
Hydrophobic interaction (53% and 39; fraction III) | 2 | 0.5 | 180 | 90 | 3.6 | P. anaerobius ATCC 27337 |
RP-HPLC (fraction IV) | 1 | 0.01 | 440 | 44,000 | 8.8 | P. anaerobius ATCC 27337 |
Fraction . | Volume (ml) . | Total protein (mg/ml) . | Total activity (AU/ml) . | Specific activity (AU/mg) . | Yield (%) . | Indicator samples of antagonism . |
---|---|---|---|---|---|---|
Crude extract | 20 | 8 | 50 | 6.25 | 1 | P. anaerobius ATCC 27337, B. merdae ATCC 43184, L. casei ATCC 7469, F. nucleatum P12.2, M 5.7, P. gingivalis ATCC 33277, and S. uberis ATCC 9927 |
Precipitation with ammonium sulphate (30–60%; fraction I) | 5 | 4.5 | 75 | 16.65 | 1.5 | P. anaerobius ATCC 27337, P. gingivalis ATCC 33277 |
Ion-exchange (fraction II) | 5 | 2 | 100 | 50 | 2.0 | P. anaerobius ATCC 27337 |
Hydrophobic interaction (53% and 39; fraction III) | 2 | 0.5 | 180 | 90 | 3.6 | P. anaerobius ATCC 27337 |
RP-HPLC (fraction IV) | 1 | 0.01 | 440 | 44,000 | 8.8 | P. anaerobius ATCC 27337 |
The positive fraction was then submitted to hydrophobic interaction chromatography, and the active substance was detected between 53 and 39% ammonium sulphate (fraction III, Table 1).
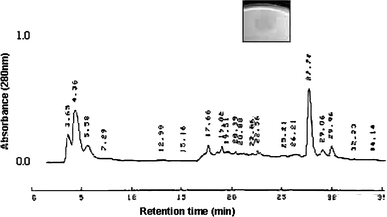
Elution profile of the fourth-round purification by reversed-phase chromatography in HPLC, in a C-18 column using pooled active fraction from the third pass as the starting material. Bacteriocinogenic activity was found in the fraction eluted at 27.72 min, and an inhibition halo was inserted
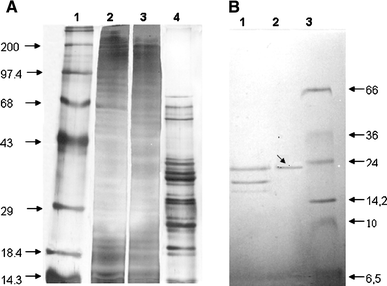
SDS-PAGE 12% from each purification step of actinomycetemcomitin. a Lanes: 1 molecular weight standards (200–14.5 KDa), 2 crude extract, 3 fraction precipited with 30–60% ammonium sulphate, 4 ionic exchange FPLC. b Lanes: 1 hydrophobic interaction in FPLC, 2 gel filtration in HPLC, 3 molecular weight standards (66–6.5 KDa). The protein band presenting bacteriocin activity is indicated with an arrow
N-terminal sequencing of the 20320 Da protein using Edman degradation identified 11 amino acids in the following order: SQRLVVYCSAY, of which 36.4% were hydrophobic, and also included both acidic and basic amino acids.
Aliquots from all purification steps were assayed against all the seven indicator strains sensitive to A. actinomycetemcomitans P7.20 and the results are shown in Table 1. The bacteriocin showed stability from pH 7 to 8, but it lost its activity at other pH values. The bacteriocin was stable at 37°C for 48 h and lost its activity after 1 h of incubation at 45°C.
Trypsin, α-chymotrypsin and papain destroyed bacteriocin activity, but proteinase K only decreased the diameter of the inhibition halo (by approximately 50%).
Discussion
The classic concept of bacteriocin, from colicin studies, implies that these substances are active against bacteria from the same or similar species. However, several authors have reported about bacteriocins having a broad spectrum of action [15, 30, 10, 2]. This is also the case for the bacteriocin-like substances produced by A. actinomycetemcomitans P7.20 [22] that was submitted to partial purification and characterization processes.
The selection of culture media, supplements and incubation atmosphere were performed following an evaluation of the expression of the antagonistic activity on solid media [23]. Based on these results (data not shown), a culture medium was selected to investigate the kinetics of bacteriocin production in liquid media. Detection of bacteriocin production occurred at the end of the exponential phase, with the highest activity observed during the stationary phase. Stevens et al. [38] obtained actinobacillin from the stationary phase of A. actinomycetemcomitans growth. The production of acidiphilin 801 [6] and thuricin 7 [6] also occurred during the stationary phase. On the other hand, some bacteriocins are produced throughout bacterial growth, as observed for Enterococcus faecium [6, 34].
We purified a new bacteriocin from an intracellular extract following the procedure of Guyonnet et al. [13]. However, the antagonistic activity observed in solid media assays suggests that the bacteriocin is exported when A. actinomycetemcomitans P7.20 is grown in liquid culture media. The antagonistic activity is unstable if the crude extract is cooled or freeze-dried (data not shown).
Although Stevens et al. [38] chose to use isoelectric focusing to fraction the crude extract, we decided to fraction the intracellular extract using ammonium sulphate precipitation. A similar methodology was used to purify the fragilicin from Bacteroides fragilis [10].
Usually the methods for purification of Class IIa bacteriocins require multiple steps, including gel filtration, ion exchange, hydrophobic interaction and reverse phase chromatographic methods [13]. We have used two steps in FPLC, specifically anion exchange followed by hydrophobic interaction. Ion exchange conducted on FPLC allowed an adequate separation, once most of the protein contents were eluted at different salt concentrations compared to that which is ideal for bacteriocin elution (Fig. 2). Reversed-phase chromatography on HPLC showed a single fraction with antagonistic activity (Fig. 1). Its molecular mass is lower than 120 KDa, as described for actinobacillin purified by Stevens et al. [38].
This new bacteriocin was inactivated by heating to 45°C for 30 min, similar to actinobacillin (inactivated by 45 min exposure to 56°C). However, the actinobacillin was stable at pH values above 6.5 [16] and the bacteriocin described in this study was stable only at the narrow pH range from 7.0 to 8.0. It is worth noting that this pH range coincides with the pH range found in gingival crevices and periodontal pockets during periodontal disease [9]. On the other hand, actinobacillin was sensitive to proteolysis by pronase and trypsin [16], while our bacteriocin was sensitive to trypsin, α-chymotrypsin and papain, but only partially sensitive to proteinase K.
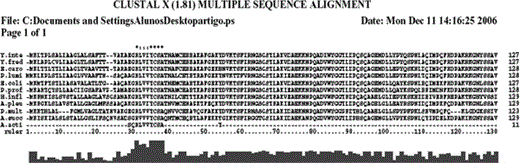
Sequence alignment of the N-terminal eleven residues of actinomycetemcomitin (Actino), with the deposited sequences of bacteriocins from other ten microorganisms, in that order: Yersinia intermedia ATCC 29909 (ZP_00832621: ABC-type Fe3+ transport system, periplasmic component); Yersinia frederiksenii ATCC 33641(ZP_00828095: ABC-type Fe3+ transport system, periplasmic component); Erwinia carotovora subsp. atroseptica SCRI1043 (YP_049599: ABC transporter iron-binding protein); Photorhabdus luminescens subsp. laumondii TTO1 (NP_928157: periplasmic ferric iron-binding protein AfuA); Escherichia coli O157:H7 EDL933 (NP_286103: periplasmic ferric iron-binding protein); Photobacterium profundum 3TCK (ZP_01219408: putative iron(III) ABC transporter, periplasmic iron-compound-binding protein); Haemophilus influenzae Rd KW20 (NP_438300: ferric ABC transporter protein); Actinobacillus pleuropneumoniae 4074 (AAB05032: AfuA); Pasteurella multocida subsp. multocida str. Pm70 (NP_245891: AfuA); Actinobacillus succinogenes 130Z (ZP_00732906: ABC-type iron/thiamine transport systems, periplasmic component); A. actinomycetemcomitans P7–20 (actinomycetemcomitin)
The availability of iron appears to be an important signal that regulates the expression of many virulence factors in pathogenic bacteria [18]. It is accepted that low concentration of free iron in the human body constitutes a limiting factor for invading pathogenic bacteria by creating conditions of iron restriction [18, 17]. Actinomycetemcomitin could present both bacteriocin and an iron related metabolic function. The mechanism of action of that substance could be linked to the capture of iron, thus limiting its availability for the growth of sensitive samples. However, little is known about iron sources and its concentration in the periodontal pocket. Recently, a microcin of Klebsiella pneumoniae RYC492 (E492) was shown to bind ferric ions and it became a novel type of antibacterial peptide termed siderophore-peptide, with a higher specific activity in terms of antibacterial properties [8]. In the pathogenesis of A. actinomycetemcomitans infections, iron regulation is thought to be important [17]. However, more work should be done, mainly regarding the action mechanism that can prove the capacity of actinomycetemcomitin in capturing iron.
Stevens, et al. [38] showed that the amount of S. sanguinis decreased in biofilms when A. actinomycetemcomitans was found, and the authors attributed this phenomenon to the production of actinobacillin, since the antagonistic substance had been shown to be active against S. sanguinis strains. However, actinomycetemcomitin did not inhibit this bacterium (data not shown), reinforcing the hypothesis that a new bacteriocin had been discovered. The complete molecular characterization of actinomycetemcomitin is now in progress.
The spectrum of action of the P7–20 sample was first tested by the overlay method against 34 reference bacteria, both Gram-positive and Gram-negative ones, and against other 75 samples of A. actinomycetemcomitans [22]. After the purification, actinomycetemcomitin was only active against P. anaerobious ATCC 27337.
Besides other ecological parameters, such as local Eh values, bacteriocin production should certainly be considered to explain the occurrence of the bacterium in diseased as well as in healthy periodontal sites. Since antagonistic abilities may confer ecological advantages for microorganisms in competitive ecosystems, it is interesting to note that A actinomycetemcomitans and Peptostretococcus anaerobius are usually recovered in increased numbers from diseased periodontal sites with different probe depth. Possible factors that might impact on the nature of colonizing species such as adhesion, coaggregation, nutrient availability and antagonistic and synergistic interactions have been studied in vitro for many years. However, the validity, or lack thereof, of postulated mechanisms of the acquisition, development and regulation of the complex microbial ecosystem has been difficult to confirm or refute in vivo [14].
The limited spectrum of action of this new bacteriocin contrasts with the ability of A. actinomycetemcomitans to antagonize a wide spectrum of Gram-positive and Gram-negative bacteria, such as P. anaerobius ATCC 27337, B. merdae ATCC 43184, L. casei ATCC 7469, F. nucleatum P12.2, M 5.7, P. gingivalis ATCC 33277 and S. uberis ATCC 9927 [22]. This observation suggests that other antagonistic substances may be produced by A. actinomycetemcomitans and that they may have an important ecological role in regulating the oral biolfilm. Further studies are necessary to elucidate these ecological aspects.
Acknowledgments
The authors are grateful to Luzia R. Rezende and José Sérgio B. Souza (AT/CNPq) for their technical support with the experiments and to the following institutions for their financial support and the use of their facilities: CNPq, FAPEMIG and CAPES.
References
Mayr-Harting A, HedgesAJ, Berkeley RCW (1972) Methods for studying bacteriocins. In: Methods in microbiology. Academic, Cap 7A, New York, pp 315–422