-
PDF
- Split View
-
Views
-
Cite
Cite
Mark V Horton, Emily F Eix, Chad J Johnson, Megan E B Dean, Brody D Andes, Kayla M Wartman, Jeniel E Nett, Impact of Micafungin on Candida auris β-glucan Masking and Neutrophil Interactions, The Journal of Infectious Diseases, Volume 230, Issue 3, 15 September 2024, Pages 763–767, https://doi.org/10.1093/infdis/jiae043
- Share Icon Share
Abstract
Invasive fungal pathogen Candida auris has become a public health threat causing outbreaks of high mortality infections. Drug resistance often limits treatment options. For Candida albicans, subinhibitory concentrations of echinocandins unmask immunostimulatory β-glucan, augmenting immunity. Here we analyze the impact of echinocandin treatment of C. auris on β-glucan exposure and human neutrophil interactions. We show subinhibitory concentrations lead to minimal glucan unmasking and only subtle influences on neutrophil functions for the isolates belonging to circulating clades. The data suggest that echinocandin treatment will not largely alter phagocytic responses. Glucan masking pathways appear to differ between C. auris and C. albicans.
The recently emergent fungal pathogen Candida auris colonizes the skin of patients in health care settings and can cause invasive disease with mortality reports as high as over 50% [1–3]. C. auris frequently exhibits antifungal resistance to 1 or more major antifungal drug classes, limiting effective available treatment options [2, 4]. Resistance patterns vary by geography and strain, but are estimated at >90% for fluconazole, near 35% for amphotericin B, and 5%–40% for echinocandin drugs [1, 2]. In addition to the organism's concerning intrinsic resistance patterns, antifungal resistance can emerge during treatment and strains resistant to all approved therapies have arisen [5].
Additionally, C. auris appears to evade some immune responses, including phagocytosis by neutrophils [6, 7]. C. auris displays outer cell wall N-linked mannans that mask immunostimulatory pathogen-associated molecular patterns (PAMPs), including β-glucan and chitin [8]. In work examining Candida albicans, echinocandin treatment has been shown to unmask these PAMPs and augment immune responses, including neutrophil phagocytosis and killing [9–11]. We questioned if echinocandin treatment would similarly promote glucan unmasking for C. auris. We were particularly interested in examining this response for isolates with higher echinocandin minimum inhibitory concentrations (MICs) given the limited treatment options for patients with infections caused by these strains. We reasoned that if echinocandins were found to alter the surface of C. auris to promote immunity, the drugs may have therapeutic value even if the organisms were relatively resistant to direct killing at therapeutic concentrations.
METHODS
For study, we selected C. auris isolates from the Centers for Disease Control and Prevention and previously described C. albicans isolates (Supplementary Table 1) [1, 12, 13]. To determine subinhibitory concentrations, overnight cultures were diluted 1:15 in yeast extract peptone dextrose (YPD) broth, grown for 2 hours in an orbital shaker, washed, diluted to 2 × 103 cells/mL in YPD, added to a microtiter plate containing serial dilutions of micafungin, and analyzed at optical density 600 nm (OD600) after 24 hours. We analyzed β-glucan cell wall exposure following micafungin treatment as previously described [8] using Fc (human):dectin-1 (mouse) recombinant protein (Adipogen) with Alexa Fluor 488-conjugated anti-human immunoglobulin G (IgG) Fc antibody (Fc + 488; Biolegend). We quantified fluorescence by plater reader (488/519 nm) or imaged with fluorescence microscopy [8].
For phagocytosis assays, human blood was obtained from donors with informed written consent through a protocol that was approved by the Internal Review Board of the University of Wisconsin-Madison. Neutrophils were isolated using MACSxpress negative antibody selection kit and purified with the MACSxpress erythrocyte depletion kit (Miltenyi Biotec, Inc) and fluorescently labeled with calcein AM. Neutrophils (1 × 105) were then added to tissue-cultured microslides (Ibidi) containing 4 × 105 calcofluor white-stained Candida (with or without micafungin pretreatment) for 1 hour and imaged as previously described [6]. To assess fungal killing, neutrophils (106 cells/mL in Roswell Park Memorial Institute medium [RPMI] + 2% fetal bovine serum) were cocultured with micafungin-treated and -untreated cells (0.015 and 0.03 µg/mL) for 2 hours. To lyse neutrophils and disrupt aggregates, we treated with DNase (1 mg/mL in double-distilled H2O for 20 minutes) twice, transferred contents to Eppendorf tubes for vortexing, and serially diluted and plated to enumerate colony-forming units.
RESULTS
To determine if echinocandin treatment may augment immune recognition for drug-resistant isolates, we selected 2 C. auris isolates (B11219 and B11211) with high MICs for the echinocandin, micafungin (MIC = 4 µg/mL) for study (Supplementary Table 1) [1]. These strains exhibit MICs at the tentative breakpoint for echinocandin resistance. The higher MICs correlate with higher treatment failure rates for those with invasive C. auris infection (https://www.cdc.gov/fungal/candida-auris/c-auris-antifungal.html). We incubated the isolates with serial dilutions of micafungin to identify subinhibitory concentrations that resulted in less than 25% inhibition in our assay conditions (Figure 1A). For both isolates, we selected 0.015, 0.03, 0.06, and 0.125 µg/mL for further study, as these concentrations had minimal inhibition and represent achievable free drug concentrations in patients [14].
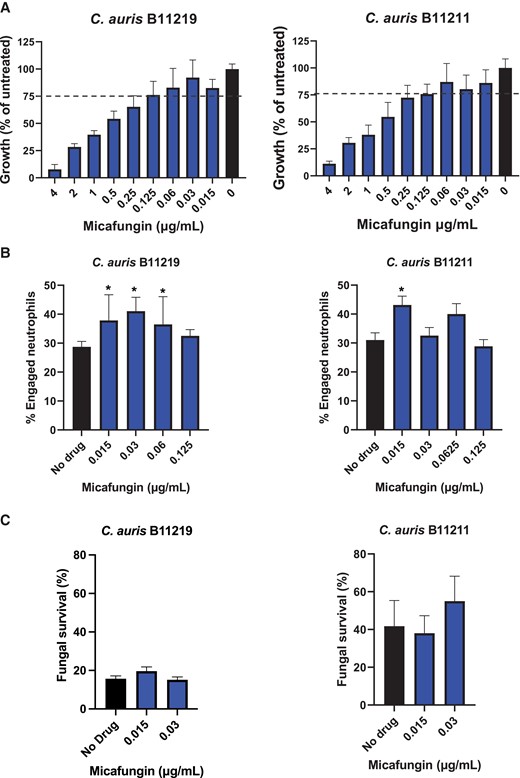
Impact of subinhibitory micafungin treatment of Candida auris on phagocytosis and killing by neutrophils. A, Micafungin was serially diluted in yeast extract peptone dextrose (YPD) and combined with C. auris B11219 or B11211 in YPD for 24 hours. Growth was assessed by OD600. Data are presented as a percentage of untreated controls with mean and SEM. Bars above the dashed line represent drug concentrations resulting in 25% or less grow inhibition. B, C. auris strains were treated with micafungin for 24 hours, labeled with calcofluor white, incubated with human neutrophils for 1 hour, and imaged via fluorescence microscopy. The number of neutrophils engulfing yeast cells was enumerated and the percentage of total engaged neutrophils was calculated. C, C. auris was treated with micafungin for 24 hours and cocultured with human neutrophils for 2 hours. Fungal survival was determined by plating for viable burden; n ≥ 3, mean with standard error of the mean is shown. *P < .05 by 1-way ANOVA with Holm-Sidak multiple comparisons to untreated controls.
To assess if echinocandin treatment of C. auris alters the neutrophil interactions, we pretreated C. auris with subinhibitory drug concentrations and measured phagocytosis (Figure 1B). We found that echinocandin treatment enhanced phagocytosis by a relatively small, but statistically significant, percentage for a subset of concentrations. The greatest increases (approximately 5% to 15%) were found for the lower drug concentrations (0.015 and 0.03 µg/mL), so we elected to use these in subsequent studies. To assess the biological relevance of the changes in phagocytosis, we examined the capacity of neutrophils to kill C. auris with and without micafungin pretreatment (Figure 1C). We observed minimal changes in fungal killing that did not reach statistical significance. Similarly, we analyzed the impact of micafungin treatment of C. auris on other neutrophil processes and did not find differences in reactive oxygen production or neutrophil extracellular trap formation (Supplementary Figure 1).
To determine if micafungin treatment impacts β-glucan masking, we analyzed cell surface glucan using a recombinant dectin-1 receptor. Compared to C. albicans, we observed very little fluorescence for either isolate (Figure 2A). For strain B11219 we found decreased surface glucan following micafungin treatment. In contrast, we noted a small increase for the B11211 strain after the 0.03 µg/mL micafungin treatment, but this value was over 100-fold lower than values observed for C. albicans. We subsequently examined C. auris by fluorescence microscopy and found no obvious unmasking patterns upon micafungin treatment (Figure 2B). The overall fluorescence for each C. auris condition was minimal in comparison to what was observed for micafungin-treated C. albicans. These data suggest that subinhibitory concentrations of micafungin induce minimal, if any, glucan unmasking.
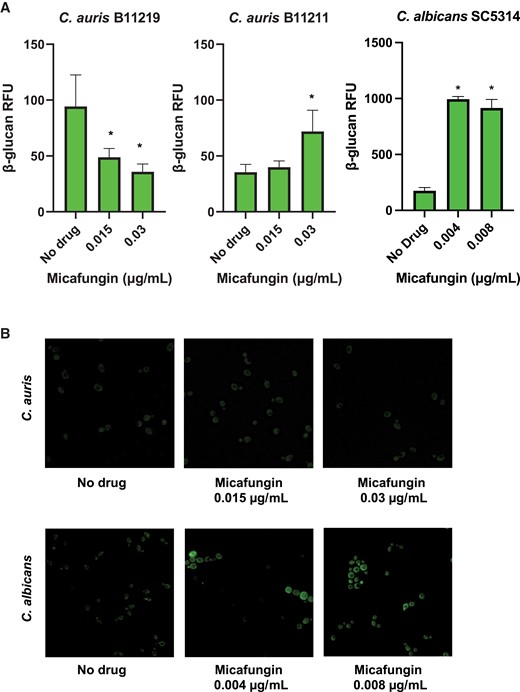
Candida auris cell surface β-glucan exposure following micafungin treatment. A, C. auris and Candida albicans were treated for 24 hours with micafungin and cell surface β-glucan was labeled using Fc:dectin-1 protein with Alexa Fluor 488-conjugated anti-human IgG Fc antibody. Fluorescently labeled exposed β-glucan was quantified by plate reader; n = 3 mean with standard error of the mean is shown. *P < .05 by 1-way ANOVA with Holm-Sidak multiple comparisons to untreated C. auris. B, Representative fluorescence images at 60x are shown for C. auris B11211 and C. albicans SC5314. Abbreviations: IgG, immunoglobulin G; RFU, relative fluorescence unit.
We next questioned if the low-level glucan exposure correlated with the high micafungin MICs for these isolates. We examined glucan exposure for the collection of 10 C. auris isolates and observed variability (Supplementary Figure 2). However, high micafungin MICs did not appear to correlate with glucan exposure as all the other isolates had lower MICs and some strains displayed more glucan and some displayed less. We next questioned if micafungin treatment impacted chitin exposure and found trends similar to β-glucan exposure for both isolates, without statistical significance (Supplementary Figure 3). To determine if this phenotype of persistently masked glucan was relevant to other C. auris isolates, we examined 4 other strains, including 1 from each of 4 clades, and isolates with lower MICs (Supplementary Figure 4). Upon treatment with subinhibitory micafungin concentrations, minimal unmasking was observed for all strains except 1. We observed unmasking for B11220, an isolate from Japan belonging to the East Asia clade, a unique clade with the propensity for the ear that does not cause large outbreaks of invasive disease [15].
DISCUSSION
Echinocandin drugs remain a first-line treatment for C. auris infections in light of high resistance rates to fluconazole and amphotericin B. However, echinocandin resistance is emerging and isolates resistant to all 3 drug classes have been identified [1, 2]. We considered if echinocandin therapy may alter the cell walls of C. auris and augment immunity, similar to responses observed for C. albicans [9]. However, we found that subinhibitory echinocandin treatment minimally influenced glucan exposure and neutrophil responses, suggesting that the drug is unlikely to produce immune-mediated therapeutic benefit. Of note, an isolate of the East Asia clade did exhibit unmasking and further understanding of this signaling mechanism may be of interest for future study.
In other work, unmasking of glucan through genetic disruption of mannosylation correlated with increased phagocytosis and killing by neutrophils [8]. Interestingly, the C. auris machinery for glucan masking appears divergent from C. albicans and C. glabrata. It is possible that the regulatory pathways induced by the stress of echinocandins vary between C. auris and C. albicans. Given the limited therapeutic options for treatment of C. auris infections, further understanding of the regulation of glucan exposure may identify pathways to target and augment immunity.
Supplementary Data
Supplementary materials are available at The Journal of Infectious Diseases online (http://jid.oxfordjournals.org/). Supplementary materials consist of data provided by the author that are published to benefit the reader. The posted materials are not copyedited. The contents of all supplementary data are the sole responsibility of the authors. Questions or messages regarding errors should be addressed to the author.
Notes
Financial support. This work was supported by the National Institutes of Health (grant numbers R01AI145939 and R21AI159583 to J. E. N.); and the National Science Foundation Graduate Research Fellowship Program (grant number DGE-1747503 to E. F. E.).
References
Author notes
M. V. H., E. F. E, and C. J. J. contributed equally to this work.
Potential conflicts of interest. All authors: No reported conflicts of interest. All authors have submitted the ICMJE Form for Disclosure of Potential Conflicts of Interest. Conflicts that the editors consider relevant to the content of the manuscript have been disclosed.