-
PDF
- Split View
-
Views
-
Cite
Cite
Arabella S V Stuart, Miia Virta, Kristi Williams, Ilkka Seppa, Robyn Hartvickson, Melanie Greenland, Edmund Omoruyi, Arangassery Rosemary Bastian, Wouter Haazen, Nadine Salisch, Efi Gymnopoulou, Benoit Callendret, Saul N Faust, Matthew D Snape, Esther Heijnen, Phase 1/2a Safety and Immunogenicity of an Adenovirus 26 Vector Respiratory Syncytial Virus (RSV) Vaccine Encoding Prefusion F in Adults 18–50 Years and RSV-Seropositive Children 12–24 Months, The Journal of Infectious Diseases, Volume 227, Issue 1, 1 January 2023, Pages 71–82, https://doi.org/10.1093/infdis/jiac407
- Share Icon Share
Abstract
Respiratory syncytial virus (RSV) remains a leading cause of pediatric morbidity, with no approved vaccine. We assessed the safety and immunogenicity of the Ad26.RSV.preF vaccine candidate in adults and children.
In this randomized, double-blind, phase 1/2a, placebo-controlled study, 12 adults (18–50 years) and 36 RSV-seropositive children (12–24 months) were randomized 2:1 to Ad26.RSV.preF (1 × 1011 viral particles [vp] for adults, 5 × 1010 vp for children) or placebo, at day 1 and 29, with 6-month immunogenicity and 1-year safety follow-up. Respiratory syncytial virus infection was an exploratory outcome in children.
In adults, solicited adverse events (AEs) were generally mild to moderate, with no serious AEs. In children, no vaccination-related serious AEs were reported; fever was reported in 14 (58.3%) Ad26.RSV.preF recipients. Baseline pediatric geometric mean titers for RSV A2 neutralization increased from 121 (95% confidence interval [CI], 76–191) to 1608 (95% CI, 730–3544) at day 29, and 2235 (95% CI, 1586–3150) at day 57, remaining elevated over 7 months. Respiratory syncytial virus infection was confirmed in fewer children receiving Ad26.RSV.preF (1, 4.2%) than placebo (5, 41.7%).
Ad26.RSV.preF demonstrated immunogenicity in healthy adults and toddlers, with no safety concerns raised. Evaluations in RSV-seronegative children are underway.
Respiratory syncytial virus (RSV) is the most important cause of serious acute respiratory illness in infants globally, with an estimated 33.1 million episodes of RSV acute lower respiratory tract infection (LRTI) and 3.2 million hospitalizations in children <5 years annually [1]. Despite this, there is no current licensed vaccine for RSV. An early vaccine developed against RSV in the 1960s, formalin-inactivated-RSV, was associated with enhanced respiratory disease (ERD) and deaths in a study of infants and toddlers [2]. Although the mechanisms of ERD are not fully understood, it is thought that formalin-inactivated-RSV failed to induce adequate RSV-neutralizing antibodies and induced a T-helper cell type 2 (Th2) skewed response.
There are currently several RSV fusion protein (F-protein)-based vaccines targeting older adults and maternal immunization in development; however, there have been many late-stage failures in this field [3, 4]. Until recently, a single neutralizing monoclonal antibody was the only effective prophylaxis available, but high-cost and intensive dosing largely restricted use to high-risk infants. Although advances with antenatal immunization [5] and a recently licensed extended half-life monoclonal antibody [6, 7] that may provide seasonal RSV protection through passive immunization show potential, neither approach has been shown to prevent transmission of RSV. In contrast, active immunization has the potential to provide both direct and indirect protection to at-risk groups.
An efficacious active RSV vaccine is believed to require potent neutralizing antibodies and T-helper cell type 1 (Th1)-skewed cellular immune responses [8]. This type of response has been demonstrated in other vaccines utilizing the Ad26-based platform, including against Ebola virus disease and coronavirus disease 2019 (COVID-19) [9–11]. The RSV F-protein is an attractive vaccine target, because it is essential in the development of infection in its prefusion (pre-F) conformation and is highly conserved across RSV strains [12, 13]. Epitopes specific to the pre-F protein appear to be more potent than those on the postfusion (post-F) conformation of the RSV-F protein [12, 13].
The RSV adenovirus 26 vector encoding pre-F vaccine (Ad26.RSV.preF) utilizes the Ad26 platform with RSV F-protein stabilized in the pre-F conformation and has demonstrated immunogenicity, safety, and protection from RSV infection in adult phase 1 and human challenge trials [14, 15]. This vaccine induces a Th1-biased response in neonatal mice, providing reassurance for its further development in infants [16].
This study investigated the safety, tolerability, and immunogenicity of the Ad26.RSV.preF vaccine candidate in RSV-seropositive 12- to 24-month-olds, as a precursor to the use of this vaccine in younger seronegative children (EudraCT 2017:-001345-27). Because this was the first study of Ad26.RSV.preF in a new buffer formulation, a “run-in” cohort of healthy adults aged 18–50 years was vaccinated before children to assess reactogenicity.
METHODS
Study Design
This was a randomized, double-blind, placebo-controlled phase 1/2a study with vaccination on days 1 and 29, immune follow up through 6 months post second dose, and safety follow up through 1-year after first dose (Clinical Trials Registration: NCT03303625). The study included 2 cohorts: adults aged 18–50 years and RSV-seropositive 12- to 24-month-olds.
The study was conducted at sites in the United States, Finland, and the United Kingdom, in compliance with Good Clinical Practice guidelines, the Declaration of Helsinki, and national regulatory requirements and was approved by the institutional review boards and research ethics in relevant countries. All participants or their parents/legal guardians provided written informed consent.
The primary objective was to assess the safety and tolerability of a 2-dose regimen of Ad26.RSV.preF in adults and RSV-seropositive children. The secondary objective was to assess humoral and cellular immune responses to Ad26.RSV.preF as measured by virus neutralization assay (VNA), F-protein binding antibodies (pre-F and post-F), and intracellular cytokine staining ([ICS] to assess Th1/Th2 phenotype). Exploratory objectives were to further assess (1) humoral and cellular immune responses in all participants and (2) RSV infection rates, symptoms of respiratory illness, and severe RSV-LRTI in children. The original sample size of 12 adults and 48 children was chosen to provide a preliminary safety and immunogenicity assessment; the pediatric sample size was subsequently amended to 36, after an initial safety assessment of the first 24 enrolled participants, which raised no concerns.
Randomization and Blinding
Participants were randomized within adult and pediatric cohorts in a 2:1 ratio to receive either 2 single doses of Ad26.RSV.preF or equivalent of placebo. Doses were given at days 1 and 29. Randomization was balanced using randomly permuted blocks. Two sentinel participants in each cohort (1:1, active/placebo) were enrolled for safety monitoring. The remainder of the blocks were filled to overall 2:1 randomization. Placebo recipients were included for blinding and safety purposes and provided additional control specimens for immunogenicity assays.
Only staff with responsibility for vaccine preparation and dispensing were aware of vaccine allocation. The investigator was not unblinded until the final analysis was performed. Primary and interim analyses were performed on data unblinded at the group level.
Participants
Included adults were aged 18–50 years and determined to be in good health. Children were aged 12–24 months, seropositive for RSV, and in good health by assessment of medical history and physical examination. Respiratory syncytial virus seropositivity was assessed by RSV enzyme-linked immune assay ([EIA] titer > 10 EIA units) or VNA (titer > 4). See Supplementary Methods for full inclusion and/or exclusion criteria.
Interventions
Ad26.RSV.preF vaccine was formulated for intramuscular injection, presented in single-use 2-mL vials, with doses of 0.5 mL (1 × 1011 viral particles [vp]) for adults and 0.25 mL (5 × 1010 vp) for children. Placebo was supplied in 2-mL ampoules (sterile 0.9% saline) for intramuscular injection.
Endpoints
Primary Endpoints
Solicited local and systemic adverse events (AEs) were collected daily for 7 days after vaccination; a full list of these symptoms and grading are provided in Supplementary Tables 1 and 2. Unsolicited AEs were collected through 28 days, and serious AEs (SAEs) were collected through 1 year postvaccination.
Clinical hematology and biochemistry blood parameters were examined in adults at baseline and 7 days after first vaccination. Clinically significant changes in vital signs were reported as AEs (baseline, day 1, day 7, day 28, and day 211).
Secondary Endpoints
Immunogenicity samples were collected on days 1, 29, 57, and 211 and evaluated according to the after assays.
Respiratory Syncytial Virus Neutralization Assays
Vaccine-induced antibody responses were investigated by determination of virus-neutralizing antibodies in a VNA using A549 cells and RSV A2 or B virus, which expresses luciferase (RSV A2-FFL or B-FFL) as previously described [15]. Assay responses of serially diluted sample were plotted in a 4-parameter logistic regression curve, and the 50% inhibitory concentration was reported as the neutralization titer.
F-Protein-Specific Immunoglobulin G Enzyme-Linked Immunosorbent Assays
A reference curve enzyme-linked immunosorbent assay (ELISA) was used to determine geometric mean binding concentrations (in ELISA units/liter) of immunoglobulin (Ig)G targeting the pre- or post-F-protein of RSV as previously described [15]. Total IgG against the randomly biotinylated pre-F protein in the stabilized conformation (RSV A2 F; GenBank ACO83301.1) and the post-F protein was evaluated.
Cell-Mediated Responses
Total cytokine response after in vitro RSV F-protein peptide stimulation was determined by ICS as described in the Supplementary Methods and reported as the percentage of CD4+ and CD8+ T cells that produced at least 1 of 3 cytokines (interferon gamma [IFNγ], tumor necrosis factor alpha [TNFα], interleukin-2 [IL-2]). The Th1-type response was defined as an increase in the percentage of IFNγ+ CD4+ T cells, and Th2-type response was defined as an increase in the percentage of IL-4+ CD4+ T cells.
Exploratory Endpoints
Immunogenicity
Further immunogenicity endpoints included (1) RSV strain cross-neutralization and (2) neutralizing antibody titers to the Ad26 vector (Supplementary Table 3).
Clinical
Surveillance for symptoms of LRTI and severe RSV-LRTI was performed in children. Samples and symptoms were collected from all participants with RTI in days 0–28 postvaccination and beyond day 28, samples and symptoms were collected only during the RSV season. Samples were collected throughout the study from any participants with a medically attended RSV-RTI or severe LRTI (see Supplementary Methods).
Statistical Analysis
The study was descriptive, without formal statistical testing. Per-protocol immunogenicity ([PPI] randomized and vaccinated participants with immunogenicity data, excluding participants with major protocol deviations impacting immunogenicity outcomes) and full analyses (all who were randomized and received at least 1 dose of study vaccine) were conducted.
Descriptive statistics (geometric mean and 95% confidence interval [CI] for ELISA and VNAs; median and quartiles for ICS) were calculated for continuous immunologic parameters at all timepoints. Primary analysis for immunogenicity was performed on the PPI set.
All reported AEs with onset up to 28 days postvaccination, and all SAEs, were included in the analysis. For each AE, the percentage of participants experiencing at least 1 occurrence were summarized by vaccination group. Unsolicited AEs were presented by System Organ Class and preferred term.
Clinical biochemistry and hematology blood parameters, and postvaccination vital signs (adult cohort), were tabulated by worst grade (US Food and Drug Administration grading). In children, clinically significant vital sign abnormalities were recorded as AEs.
RESULTS
Study Population
Twelve adults (16 screened) and 36 children (77 screened) were enrolled and received at least 1 dose of study vaccine (Figure 1). In children, 63.4% (26 of 41) of screening failures were due to RSV seronegativity.
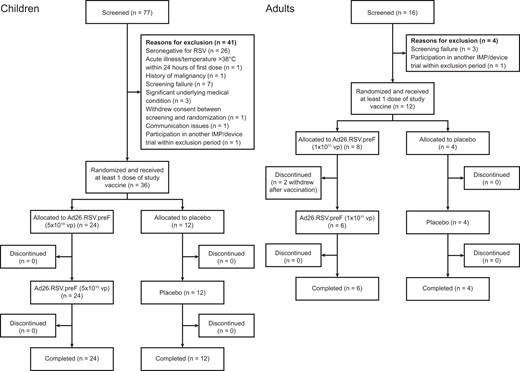
Consort flow of participants by cohort. Ad26.RSV.preF, adenovirus serotype 26 prefusion conformation-stabilized F protein; IMP, investigational medicinal product; RSV, respiratory syncytial virus; vp, viral particles.
Baseline characteristics were generally well balanced between vaccination groups in both cohorts (Table 1). The majority of adults were female (8 of 12, 66.7%), White (10 of 12, 83.3%), with median age of 36.5 years (range, 27–47). The majority of children were female (21 of 36, 58.3%), White (35 of 36, 97.2%), with median age 17.5 months (range, 13–23).
. | Children . | Adults . | ||||
---|---|---|---|---|---|---|
Characteristic . | Ad26.RSV.preF (5 × 1010 vp), Ad26.RSV.preF (5 × 1010 vp) (N = 24) . | Placebo, Placebo (N = 12) . | All Participants (N = 36) . | Ad26.RSV.preF (1 × 1011 vp), Ad26.RSV.preF (1 × 1011 vp) (N = 8) . | Placebo, Placebo (N = 4) . | All Participants (N = 12) . |
Female, n (%) | 13 (54.2) | 8 (66.7) | 21 (58.3) | 6 (75.0) | 2 (50.0) | 8 (66.7) |
Agea, median (min, max) | 18.0 (13, 23) | 17.0 (14, 23) | 17.5 (13, 23) | 37.5 (28, 47) | 32.0 (27, 41) | 36.5 (27, 47) |
Ethnicity, n (%) | ||||||
Asian | … | … | … | 1 (12.5) | … | 1 (8.3) |
White | 24 (100) | 11 (91.7) | 35 (97.2) | 6 (75.0) | 4 (100) | 10 (83.3) |
Multiple | … | … | … | 1 (12.5) | … | 1 (8.3) |
Not reported | … | 1 (8.3) | 1 (2.8) | … | … | … |
Hispanic or Latino ethnicity | 1 (4.2) | 1 (8.3) | 2 (5.6) | … | 1 (25.0) | 1 (8.3) |
Height (cm), median (min, max) | … | … | … | 167.5 (154.0, 182.0) | 173.3 (160.0, 184.0) | 167.5 (154.0, 184.0) |
Weight (kg), median (min, max) | … | … | … | 74.5 (57.5, 98.3) | 80.3 (62.7, 99.6) | 74.8 (57.5, 99.6) |
BMI (kg/m2), median (min, max) | … | … | … | 26.5 (20.6, 34.3) | 26.1 (20.9, 36.1) | 26.1 (20.6, 36.1) |
Length percentile, median (min, max) | 57.0 (9.0, 99.0) | 50.0 (3.0, 97.0) | 52.0 (3.0, 99.0) | … | … | … |
Weight percentile, median (min, max) | 83.5 (40.0, 98.9) | 56.5 (10.0, 100) | 75.0 (10.0, 100) | … | … | … |
. | Children . | Adults . | ||||
---|---|---|---|---|---|---|
Characteristic . | Ad26.RSV.preF (5 × 1010 vp), Ad26.RSV.preF (5 × 1010 vp) (N = 24) . | Placebo, Placebo (N = 12) . | All Participants (N = 36) . | Ad26.RSV.preF (1 × 1011 vp), Ad26.RSV.preF (1 × 1011 vp) (N = 8) . | Placebo, Placebo (N = 4) . | All Participants (N = 12) . |
Female, n (%) | 13 (54.2) | 8 (66.7) | 21 (58.3) | 6 (75.0) | 2 (50.0) | 8 (66.7) |
Agea, median (min, max) | 18.0 (13, 23) | 17.0 (14, 23) | 17.5 (13, 23) | 37.5 (28, 47) | 32.0 (27, 41) | 36.5 (27, 47) |
Ethnicity, n (%) | ||||||
Asian | … | … | … | 1 (12.5) | … | 1 (8.3) |
White | 24 (100) | 11 (91.7) | 35 (97.2) | 6 (75.0) | 4 (100) | 10 (83.3) |
Multiple | … | … | … | 1 (12.5) | … | 1 (8.3) |
Not reported | … | 1 (8.3) | 1 (2.8) | … | … | … |
Hispanic or Latino ethnicity | 1 (4.2) | 1 (8.3) | 2 (5.6) | … | 1 (25.0) | 1 (8.3) |
Height (cm), median (min, max) | … | … | … | 167.5 (154.0, 182.0) | 173.3 (160.0, 184.0) | 167.5 (154.0, 184.0) |
Weight (kg), median (min, max) | … | … | … | 74.5 (57.5, 98.3) | 80.3 (62.7, 99.6) | 74.8 (57.5, 99.6) |
BMI (kg/m2), median (min, max) | … | … | … | 26.5 (20.6, 34.3) | 26.1 (20.9, 36.1) | 26.1 (20.6, 36.1) |
Length percentile, median (min, max) | 57.0 (9.0, 99.0) | 50.0 (3.0, 97.0) | 52.0 (3.0, 99.0) | … | … | … |
Weight percentile, median (min, max) | 83.5 (40.0, 98.9) | 56.5 (10.0, 100) | 75.0 (10.0, 100) | … | … | … |
Abbreviations: Ad26.RSV.preF, adenovirus serotype 26 prefusion conformation-stabilized F protein; BMI, body mass index; max, maximum; min, minimum; vp, viral particles.
Age in months for children, age in years for adults.
. | Children . | Adults . | ||||
---|---|---|---|---|---|---|
Characteristic . | Ad26.RSV.preF (5 × 1010 vp), Ad26.RSV.preF (5 × 1010 vp) (N = 24) . | Placebo, Placebo (N = 12) . | All Participants (N = 36) . | Ad26.RSV.preF (1 × 1011 vp), Ad26.RSV.preF (1 × 1011 vp) (N = 8) . | Placebo, Placebo (N = 4) . | All Participants (N = 12) . |
Female, n (%) | 13 (54.2) | 8 (66.7) | 21 (58.3) | 6 (75.0) | 2 (50.0) | 8 (66.7) |
Agea, median (min, max) | 18.0 (13, 23) | 17.0 (14, 23) | 17.5 (13, 23) | 37.5 (28, 47) | 32.0 (27, 41) | 36.5 (27, 47) |
Ethnicity, n (%) | ||||||
Asian | … | … | … | 1 (12.5) | … | 1 (8.3) |
White | 24 (100) | 11 (91.7) | 35 (97.2) | 6 (75.0) | 4 (100) | 10 (83.3) |
Multiple | … | … | … | 1 (12.5) | … | 1 (8.3) |
Not reported | … | 1 (8.3) | 1 (2.8) | … | … | … |
Hispanic or Latino ethnicity | 1 (4.2) | 1 (8.3) | 2 (5.6) | … | 1 (25.0) | 1 (8.3) |
Height (cm), median (min, max) | … | … | … | 167.5 (154.0, 182.0) | 173.3 (160.0, 184.0) | 167.5 (154.0, 184.0) |
Weight (kg), median (min, max) | … | … | … | 74.5 (57.5, 98.3) | 80.3 (62.7, 99.6) | 74.8 (57.5, 99.6) |
BMI (kg/m2), median (min, max) | … | … | … | 26.5 (20.6, 34.3) | 26.1 (20.9, 36.1) | 26.1 (20.6, 36.1) |
Length percentile, median (min, max) | 57.0 (9.0, 99.0) | 50.0 (3.0, 97.0) | 52.0 (3.0, 99.0) | … | … | … |
Weight percentile, median (min, max) | 83.5 (40.0, 98.9) | 56.5 (10.0, 100) | 75.0 (10.0, 100) | … | … | … |
. | Children . | Adults . | ||||
---|---|---|---|---|---|---|
Characteristic . | Ad26.RSV.preF (5 × 1010 vp), Ad26.RSV.preF (5 × 1010 vp) (N = 24) . | Placebo, Placebo (N = 12) . | All Participants (N = 36) . | Ad26.RSV.preF (1 × 1011 vp), Ad26.RSV.preF (1 × 1011 vp) (N = 8) . | Placebo, Placebo (N = 4) . | All Participants (N = 12) . |
Female, n (%) | 13 (54.2) | 8 (66.7) | 21 (58.3) | 6 (75.0) | 2 (50.0) | 8 (66.7) |
Agea, median (min, max) | 18.0 (13, 23) | 17.0 (14, 23) | 17.5 (13, 23) | 37.5 (28, 47) | 32.0 (27, 41) | 36.5 (27, 47) |
Ethnicity, n (%) | ||||||
Asian | … | … | … | 1 (12.5) | … | 1 (8.3) |
White | 24 (100) | 11 (91.7) | 35 (97.2) | 6 (75.0) | 4 (100) | 10 (83.3) |
Multiple | … | … | … | 1 (12.5) | … | 1 (8.3) |
Not reported | … | 1 (8.3) | 1 (2.8) | … | … | … |
Hispanic or Latino ethnicity | 1 (4.2) | 1 (8.3) | 2 (5.6) | … | 1 (25.0) | 1 (8.3) |
Height (cm), median (min, max) | … | … | … | 167.5 (154.0, 182.0) | 173.3 (160.0, 184.0) | 167.5 (154.0, 184.0) |
Weight (kg), median (min, max) | … | … | … | 74.5 (57.5, 98.3) | 80.3 (62.7, 99.6) | 74.8 (57.5, 99.6) |
BMI (kg/m2), median (min, max) | … | … | … | 26.5 (20.6, 34.3) | 26.1 (20.9, 36.1) | 26.1 (20.6, 36.1) |
Length percentile, median (min, max) | 57.0 (9.0, 99.0) | 50.0 (3.0, 97.0) | 52.0 (3.0, 99.0) | … | … | … |
Weight percentile, median (min, max) | 83.5 (40.0, 98.9) | 56.5 (10.0, 100) | 75.0 (10.0, 100) | … | … | … |
Abbreviations: Ad26.RSV.preF, adenovirus serotype 26 prefusion conformation-stabilized F protein; BMI, body mass index; max, maximum; min, minimum; vp, viral particles.
Age in months for children, age in years for adults.
Primary Endpoint: Safety and Reactogenicity
Adults
In adults, local and systemic solicited AEs were mostly grade 1 or 2 severity and transient (Supplementary Tables 4–7).
All unsolicited AEs (within 28 days postdose) in adults were severity grade 1 or 2, and there were no SAEs. Vital sign and laboratory hematology abnormalities are presented in Supplementary Tables 8 and 9. There were no postvaccination biochemistry abnormalities.
Children
In children, injection site pain and/or tenderness was the most common solicited local AE, and this was reported after each dose in 14 of 24 (58.4%) in the Ad26.RSV.preF group and in 4 of 12 (33.3%) in the placebo group (Figure 2). Most solicited local AEs were severity grade 1 or 2, with only 1 participant (4.2%) experiencing grade 3 injection site pain and/or tenderness after each vaccination; all were of transient duration (Supplementary Table 7).
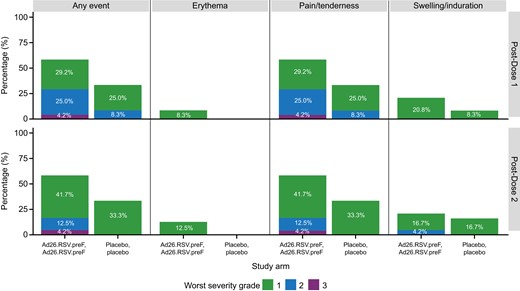
Solicited local adverse events in days 0–7 postvaccination by worst severity grade, dose, and study arm in children (full analysis set, Ad26.RSV.preF n = 24, placebo n = 12). Ad26.RSV.preF, adenovirus serotype 26 prefusion conformation-stabilized F protein.
Solicited systemic AEs in the Ad26.RSV.preF group were reported after first and second doses in 23 of 24 (95.8%) and 22 of 24 (91.7%) of participants, respectively. This compares with 10 of 12 (83.3%) and 9 of 12 (75.0%) after first and second doses in the placebo group (Figure 3). Most common were irritability and/or crying, loss of appetite, and decreased activity and/or lethargy. The majority were grade 1 or 2, with grade 3 reported in 5 of 24 (20.8%) in the Ad26.RSV.preF group versus 1 of 12 (8.3%) in the placebo group. All were of transient duration (Supplementary Table 10). Fever ≥38.0°C occurred in 14 of 24 (58.3%) participants in the Ad26.RSV.preF group, and 3 of 12 (25%) in the placebo group (Figure 3). Most fever resolved within 2 days. There was 1 grade 3 (≥40.0°C) fever in a participant in the placebo group experiencing intercurrent enterovirus infection; all others were grade 1 or 2.
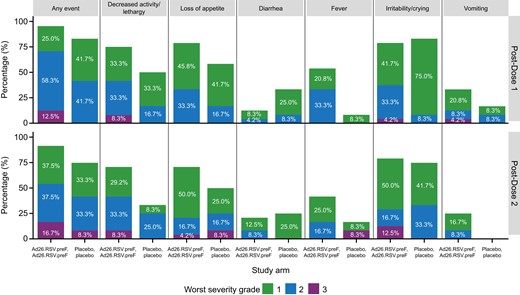
Solicited systemic adverse events in days 0–7 postvaccination by worst severity grade, dose, and study arm in children (full analysis set, Ad26.RSV.preF n = 24, placebo n = 12). Ad26.RSV.preF, adenovirus serotype 26 prefusion conformation-stabilized F protein.
Unsolicited AEs within 28 days of any vaccination were reported in 19 of 24 (79.2%) participants in the Ad26.RSV.preF group and in 10 of 12 (83.3%) in the placebo group. The most frequent events were upper respiratory tract infection, respiratory tract infection, and otitis media. All were grade 1 or 2 severity, except for 1 grade 3 AE relating to enterovirus infection in the placebo group post dose 2. Three SAEs were reported after day 28 postvaccination: a case of wheezing and a case of syncope in the placebo group, and a case of cellulitis in the Ad26.RSV.preF group. None was considered related to the study interventions, and all resolved without sequelae.
Immunogenicity: Secondary Endpoints
Adults
In adults, an increase in RSV A2 neutralizing, pre-F and post-F protein binding antibody titers were observed 28 days after the first dose with Ad26.RSV.preF. After a second dose, titers remained stable for 28 days and modestly decreased at 6 months (Supplementary Figure 1). Total cytokine response to RSV F-protein for CD4+ and CD8+ T cells and Th1 and Th2 responses are presented in Supplementary Figure 2 and 3.
Children
Immunogenicity data for children were available for n = 7–26 per assay due to no sample availability and/or low sample quality. In children, the geometric mean titer (GMT) of RSV A2-neutralizing antibody increased from 121 (95% CI, 76–191) at baseline to 1608 (95% CI, 730–3544) on day 29 after the first dose with Ad26.RSV.preF. After the second dose, GMT further increased in the Ad26.RSV.preF group to 2235 at day 57 (95% CI, 1586–3150). In the placebo group, no increase was seen from the baseline GMT of 187 (95% CI, 68–358) (Figure 4).

Neutralizing antibody titers over time in children (per-protocol immunogenicity set*, n = 26). (A) Respiratory syncytial virus (RSV) A2 strain. (B) Pre-fusion (pre-F) antibodies. (C) Post-fusion (post-F) antibodies, (D) neutralizing antibodies over time, by participant. Geometric mean with 95% confidence interval shown in the figure. N, number of participants with data at baseline. *Data for some subjects are not available due to no sample availability and/or low sample quality for the planned immunogenicity analyses. Ad26.RSV.preF, adenovirus serotype 26 prefusion conformation-stabilized F protein; ELISA, enzyme-linked immunosorbent assays; EU, ELISA units; GMT, geometric mean titer; vp, viral particles.
Pre-F and post-F-specific antibodies increased substantially from baseline post-vaccination with Ad26.RSV.preF, with no increase in placebo recipients (Figure 4). After the second vaccination with Ad26.RSV.preF, pre-F and post-F antibody titers increased modestly, with GMT reaching 1918 (95% CI, 1497–2456) and 863 (95% CI, 630–1184) on day 57, respectively.
Six months after the second vaccination (day 211), GMT of RSV A2-neutralizing antibody titers, pre-F protein antibody titers, and post-F-protein antibody titers decreased from peak responses to 1164 (95% CI, 734–1847), 924 (95% CI, 639–1334), and 420 (95% CI, 306–576), respectively, but they remained well above baseline (Figure 4).
Total cytokine response to RSV F-protein for CD4+ and CD8+ T cells in children is presented in Figure 5. In the Ad26.RSV.preF group, group median values increased from 0.030 (interquartile range [IQR], 0.013–0.095) at baseline to 0.089 (IQR, 0.062–0.131) on day 29 for CD4+ T cells. One participant did not show an increase in response over baseline. Group median values modestly increased from 0.033 (IQR, 0.024–0.049) at baseline to 0.077 (IQR, 0.024–0.116) on day 29 for CD8+ T cells; some participants did not show an increase in response (Figure 5). The ICS data indicated a total cytokine response for both CD4+ and CD8+ T cells and a predominant Th1 response 28 days after the first vaccination in the Ad26.RSV.preF group (Figure 6).
![Total cytokine response (mock subtracted intracellular cytokine staining) over time in children (per-protocol immunogenicity set*, n = 22). (A) CD4+ T-cell response. (B) CD8+ T-cell response. Values below 0.001% were imputed with 0.0005%. Total cytokine response after in vitro respiratory syncytial virus (RSV) F-protein peptide stimulation was reported as the percentage of CD4+ and CD8+ T cells that produce at least 1 of 3 cytokines (interferon γ [IFNγ], tumor necrosis factor α, and/or interleukin-2). Th1-type response was defined as an increase in the percentage of CD4+IFNγ+ T cells. *Data for some subjects are not available due to no sample availability and/or low sample quality for the planned immunogenicity analyses. †n = 13 with sample available after day 1. Ad26.RSV.preF, adenovirus serotype 26 prefusion conformation-stabilized F-protein; F-protein, fusion protein; vp, viral particles.](https://oup.silverchair-cdn.com/oup/backfile/Content_public/Journal/jid/227/1/10.1093_infdis_jiac407/1/m_jiac407f5.jpeg?Expires=1750194635&Signature=lMrChLXVtD88omZG4im0RmMs5ONeSe5fyC7Nz~2GzyBcKKVrurSYt8WoGw-5zZWBN56GfwTXvkHkr~164ZWR137SStq-I~MWq3YTsJGrLe~~OO6dhoq5qhtuOacsRVpoddgXgsCgPnUcyykqwUn~OB-O3XWcZXMvZYC4UjQONaQiHNi1Dc4NVN5EQFn0a5po--q7Z9z~ip702CDbcX~MR1YZqEJW6Ti-zRdgQlpNSvkyIS5EJhr6AfZq0Ekn5k2srJlYEOXQ2mblIFIU-gNNz69fvSOU5LJBKa1qhA0LqcW9HE0DIWHuXlAWrzMTyB1W9nhOwd-VR5bGzP58fJhEjw__&Key-Pair-Id=APKAIE5G5CRDK6RD3PGA)
Total cytokine response (mock subtracted intracellular cytokine staining) over time in children (per-protocol immunogenicity set*, n = 22). (A) CD4+ T-cell response. (B) CD8+ T-cell response. Values below 0.001% were imputed with 0.0005%. Total cytokine response after in vitro respiratory syncytial virus (RSV) F-protein peptide stimulation was reported as the percentage of CD4+ and CD8+ T cells that produce at least 1 of 3 cytokines (interferon γ [IFNγ], tumor necrosis factor α, and/or interleukin-2). Th1-type response was defined as an increase in the percentage of CD4+IFNγ+ T cells. *Data for some subjects are not available due to no sample availability and/or low sample quality for the planned immunogenicity analyses. †n = 13 with sample available after day 1. Ad26.RSV.preF, adenovirus serotype 26 prefusion conformation-stabilized F-protein; F-protein, fusion protein; vp, viral particles.
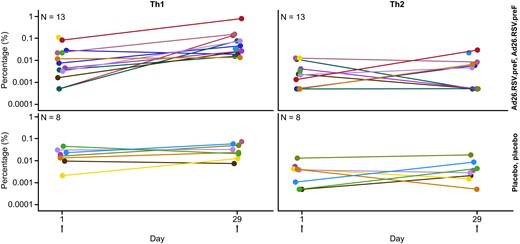
T-helper cell type 1 (Th1) and Th2 profiles in children (per-protocol immunogenicity set*, n = 21). Values below 0.001% were imputed with 0.0005%. *Data for some subjects are not available due to no sample availability and/or low sample quality for the planned immunogenicity analyses. Ad26.RSV.preF, adenovirus serotype 26 prefusion conformation-stabilized F protein.
Exploratory Endpoints
The RSV-B strain cross-neutralization assay was performed for the pediatric cohort. In the Ad26.RSV.preF group, GMT increased from 907 (95% CI, 542–1517) at baseline to 25 306 (95% CI, 19 028–33 656) at day 57. There was no change from baseline in the placebo group (Supplementary Figure 4).
In the adult cohort, Ad26-seropositive participants increased from 2 of 8 (25%) at baseline to 5 of 6 (83.3%) at day 29 in the Ad26.RSV.preF group. In children, Ad26-seropositive participants increased from 2 of 17 (11.8%) at baseline to 10 of 16 (62.5%) at day 29 in the Ad26.RSV.preF group. All participants in placebo groups in both cohorts were Ad26-seronegative at baseline and remained seronegative on day 29.
Only 1 of 24 children (4.2%) Ad26.RSV.preF recipients had evidence of virologically confirmed RSV-RTI compared with 5 of 12 placebo recipients (41.7%) (Supplementary Table 11). The confirmed RSV infection in the Ad26.RSV.preF group was due to RSV-B strain; in the placebo group, 2 participants had RSV-A strain infection, and 3 participants had RSV-B strain. No confirmed RSV-RTI in either group met criteria for LRTI.
DISCUSSION
In this study, we demonstrated that administration of Ad26.RSV.preF to RSV-seropositive children aged 12–24 months generated a humoral and cellular immune response and had an acceptable reactogenicity profile. There was also evidence of humoral immunogenicity in adults receiving Ad26.RSV.preF, with an acceptable reactogenicity profile, consistent with the results of prior adult studies [14, 15].
No safety concerns were raised in adults or children receiving Ad26.RSV.preF. Most local and systemic solicited reactogenicity in adults and children was below grade 3 and of short duration [15]. Transient fevers after vaccination were commonly observed in RSV-seropositive children and adults (58.3% and 50%, respectively). Based on these results, a dose reduction was implemented in an ongoing study of Ad26.RSV.preF in RSV-seronegative children [17].
Immunization with Ad26.RSV.preF induced humoral immune responses including RSV A2 neutralizing, pre-F-protein, and post-F-protein binding antibodies 28 days after first dose in both adults and children. Immunization with this vaccine also induced cross-neutralizing antibodies against the RSV-B strain in children, as seen in the phase 1 trial in adults >60 years, and with other F-protein based vaccines [15, 18]. This is encouraging in suggesting potential cross RSV-A and RSV-B protection in RSV pre-exposed individuals. In children, a second vaccination with Ad26.RSV.preF resulted in only a modest increase in humoral immune responses in the short term. Although this raises the possibility of using a single dose in a known seropositive population, pragmatically, distinguishing serostatus before immunization would be challenging. Two children showed no increase in humoral immune responses after first dose, both responded to the second dose; given both were Ad26 seronegative at baseline, the lack of initial response did not appear to be related to antivector immunity.
Responses appeared durable to at least 6 months after second dose, consistent with previous trials using the Ad26 viral vector—in the phase 1 study of Ad26.RSV.preF, responses were maintained at 2 years, and an Ebola vaccine schedule with Ad26.ZEBOV prime and MVA-BN-Filo boost showed persistent responses 2 and a half years postimmunization [15, 19]. The burden of pediatric RSV is mainly in the first 2 years of life, with peak risk of severe disease in the first 6 months [20]; a vaccine that can induce protection in infancy and sustain protection over the early years is critical.
Recent RSV vaccine candidates using F-protein have incorporated the post-F form, delivered variously through protein-adjuvanted, recombinant protein nanoparticle, and adenoviral-vectored platforms [21–23]. These have all been shown to be immunogenic, generating both neutralizing and post-F binding antibodies [21–23]. However, recent data have shown neutralizing activity in human sera to predominantly derive from pre-F binding antibodies [12, 13]. This may account for one of these RSV vaccine candidates failing to provide protection against RSV in adults aged >60, or why another did not meet the primary endpoint for protection of infants via maternal immunization, although it did show protective efficacy against severe disease, particularly in the South African setting [5, 21]. Epitopes exposed on the RSV F-protein stabilized in its pre-F conformation may be critical for developing potent neutralizing antibodies; a potential advantage for this vaccine.
The potential for ERD is of critical importance in RSV vaccine development for the RSV-naive pediatric population; therefore, it is reassuring that Ad26.RSV.preF generated a predominant Th1 type CD4+ T-cell response in both RSV-seropositive adults and children, consistent with prior results [15], rather than an ERD-associated Th2-skewed response [24, 25]. Similarly encouraging is direct evidence of protection from a single dose of Ad26.RSV.pre-F against symptomatic RSV infection in a recent human challenge study of intranasal RSV in healthy adults aged 18–50 years [14], supported by a promising signal from the exploratory objective in this study suggesting lower rates of RSV in the actively immunized group compared with placebo recipients.
Within the broader RSV field, there remain significant advantages in continuing to target an active immunization strategy. Until recently, passive immunization through monoclonal antibodies has been limited by cost and the need for 5-weekly dosing during RSV season to high-risk infants in high-income settings [26]. An extended half-life monoclonal antibody (nirsevimab), given as a single dose before RSV season, has been recently demonstrated to be efficacious in healthy preterm infants, and trials in term infants are ongoing [27, 28] and could represent a significant improvement over existing monoclonal antibody prophylaxis. However, this approach is likely to be most useful in infants <6 months of age, whereas active vaccination has the most potential to develop multiseason durable responses in children with more mature immune systems [29]. Older children are often responsible for transmitting RSV to younger, more vulnerable siblings, and an active vaccination strategy in this age-group may provide indirect protection to the most at-risk group who also have the least mature immune responses [30].
A limitation of this study is that data generated in RSV-seropositive children may not be representative of responses in seronegative infants—the ultimate target population for this vaccine. In addition, assessing the long-term duration of humoral responses is challenging due to limitations in study follow up. Adenoviral-vectored vaccines have been shown to generate robust immune responses; however, questions surrounding antivector immunity and the impact on immunogenicity when used sequentially, for different pathogen targets, remain. In this study, the low number of participants with pre-existing Ad26-neutralizing antibodies limited assessment of the impact of Ad26 seropositivity on vaccine-induced immune responses, although a previous study showed no significant effect [15]. An important question raised is whether there may be potential impact of passively transferred maternal antivector antibodies on infant protection, where an infant is vaccinated with an adenoviral-vectored vaccine. This might be relevant in the context of the current COVID-19 pandemic and the use of Ad26-vectored vaccines in immunization programs.
CONCLUSIONS
Respiratory syncytial virus is a longstanding and ongoing blight for young children, a major cause of hospital admission, and a major cause of childhood mortality in low- and middle-income countries [1]. In this study, Ad26.RSV.preF had an acceptable safety profile, was well tolerated, and elicited humoral and cellular immune responses in adults and RSV-seropositive children. Fewer RSV infections were observed in Ad26.RSV.preF recipients compared with placebo recipients. Generating data in vaccine-naive children and infants is a crucial next step for prevention of this ubiquitous viral infection, and further studies in RSV-seronegative children aged 12–24 months are ongoing [17].
Supplementary Data
Supplementary materials are available at The Journal of Infectious Diseases online. Consisting of data provided by the authors to benefit the reader, the posted materials are not copyedited and are the sole responsibility of the authors, so questions or comments should be addressed to the corresponding author.
Notes
Acknowledgments. The Oxford Vaccine Group acknowledges Emma Plested, Danielle Campbell, Hannah Robinson, Jonathan Gardner, and Katie O’Brien for support of the study delivery. The Southampton NIHR Clinical Research Facility acknowledges Stephen Saich, Carine Fixmer, Diane Gbsemete, Hans De Graaf, Maaike Kusters, Tanya Ogrina, Ruth Ensom, and Emily Cornish for support of study delivery. R. H. acknowledges the Research Team at Antimicrobial Resistance Research. We acknowledge Karin Weber for her involvement in the publication team, including interpretation of the data, and review of the final manuscript.
Financial support. This work was sponsored, designed, and managed by Janssen Pharmaceuticals during all stages of the trial and its analysis.
References
Author notes
Presented in part: ISIRV Virtual Congress 2021, February 2021; ESPID 2020 Virtual Meeting, April 2021.
Potential conflicts of interest. M. D. S. was an NIHR Senior Investigator and receives salary support from the Oxford NIHR Biomedical Research Centre when undertaking this work. S. N. F. is an NIHR Senior Investigator and receives salary support from the NIHR Southampton Clinical Research Facility and Biomedical Research Centre. K. W., A. R. B., W. H., N. S., E. G., E. O., and B. C., and E. H. are employees of Janssen and may be Johnson & Johnson stockholders. M. D. S. received research grants (through The University of Oxford) from the following: GlaxoSmithKline, Pfizer, Janssen, MCM vaccines, MedImmune, and AstraZeneca, vaccine from Novavax for COVID-19 vaccine studies. He received no personal payment for this work. MDS was a member of medical advisory boards for the Meningitis Research Foundation. From September 2022 MDS is employed by Moderna Biotech UK; this work was completed prior to this employment. S. N. F. received research grants (through University Hospital Southampton) from the following: Johnson & Johnson, Pfizer, Sanofi, GlaxoSmithKline, Merck, AstraZeneca, and Valneva; honoraria from Johnson & Johnson and Pfizer; he has been an advisor for AstraZeneca, MedImmune, Sanofi, Pfizer, Seqirus, Merck, Johnson & Johnson, and GlaxoSmithKline (but received no personal payments for this work, all honoraria were paid to University Hospital Southampton NHS Foundation Trust); and he acted as a paid chair of UK NICE Sepsis and Lyme Disease Guidelines. I. S. received expenses from Janssen to cover travel to an investigator meeting. All authors have submitted the ICMJE Form for Disclosure of Potential Conflicts of Interest. Conflicts that the editors consider relevant to the content of the manuscript have been disclosed.