-
PDF
- Split View
-
Views
-
Cite
Cite
Iracema Luisa Quintino-de-Carvalho, Marcela Helena Gonçalves-Pereira, Michele Faria Ramos, Bruno Henrique Gonçalves de Aguiar Milhim, Último Libânio Da Costa, Érika Gonçalves Santos, Maurício Lacerda Nogueira, Helton Da Costa Santiago, Type 1 Innate Lymphoid Cell and Natural Killer Cells Are Sources of Interferon-γ and Other Inflammatory Cytokines Associated With Distinct Clinical Presentation in Early Dengue Infection, The Journal of Infectious Diseases, Volume 225, Issue 1, 1 January 2022, Pages 84–93, https://doi.org/10.1093/infdis/jiab312
- Share Icon Share
Abstract
Increased levels of inflammatory cytokines are associated with severe dengue evolution, but the source of such hypercytokinemia is elusive. We investigated the contribution of innate lymphocytes, innate lymphoid cells (ILCs), and natural killer (NK) cells in cytokine production in early dengue infection.
Peripheral blood mononuclear cells of individuals with dengue without warning signs (DWS–) and dengue with warning signs and severe dengue (SD) presentation combined (DWS+) were obtained between 2 and 7 days since fever onset and submitted to flow cytometry without specific antigen stimulation to evaluate cytokines in ILC and NK cell subpopulations.
ILCs and NK cells were found to be important sources of cytokines during dengue. ILCs of the DWS+/SD group displayed higher production of interferon gamma (IFN-γ) and interleukin (IL) 4/IL-13 when compared to DWS– individuals. On the other hand, NK Eomes+ cells of DWS– patients displayed higher IFN-γ production levels compared with the DWS+/SD group. Interestingly, when NK cells were identified by CD56 expression, DWS+/SD displayed higher frequency of IL-17 production compared with the DWS– group.
These results indicate that ILCs and NK cells are important sources of inflammatory cytokines during acute dengue infection and display distinct profiles associated with different clinical forms.
Dengue is a mosquito-borne disease considered ubiquitous among the tropics with an estimated incidence of 390 million cases annually [1] caused by 4 distinct antigenically related serotypes of Denguevirus (DENV-1, DENV-2, DENV-3, and DENV-4). The clinical evolution of the disease varies from asymptomatic or subclinical presentation in the majority of cases, to fatal conditions [1]. Symptomatic dengue can be classified as dengue without warning signs (DWS–), a self-limited illness with flu-like characteristics including headache, fatigue, myalgia, and cutaneous rash; dengue with warning signs (DWS+), which presents with rapid decrease of platelets and minor hemorrhagic manifestations; and severe dengue (SD), a life-threatening manifestation with compromised target organs [2].
The mechanisms underlying different clinical phenotypes of dengue are dependent of multiple viral and host factors. Among these mechanisms, the antibody-dependent enhancement postulates that during a secondary infection by a heterologous DENV serotype, nonneutralizing cross-reactive antibodies can facilitate the infection of FcγR-bearing cells promoting the replication of DENV and development of DWS+ and SD [3]. Additionally, the presence of preexisting cross-reactive memory T cells, the so-called original antigenic sin, resulting from a primary infection was also thought to contribute to immune system hyperactivation and increased “cytokine storm” [4–7]. Among the cytokines found in plasma associated with DWS+ and SD presentation are interferon gamma (IFN-γ), tumor necrosis factor alpha (TNF-α), interleukin (IL) 10, and others, which are usually produced very early during infection [8–14], sometimes detected even before fever onset [12]. Paradoxically, some studies have found that T-cell activation, even preformed memory cells, producing IFN-γ, TNF-α, and IL-10 are associated with asymptomatic or mild dengue cases in humans [15, 16] or protection in murine models of dengue [17–20]. Interestingly, innate immune cell sources of these cytokines in dengue infection have been scarcely studied.
Innate immune cells, like natural killer (NK) cells, play important roles in the rapid response to viral infection producing IFN-γ and promoting cytotoxic elimination of infected cells [21]. Although it is widely speculated that hyperactivation of the immune system can contribute to tissue damage and vascular permeability in SD, most of the studies and attention are focused on T-cell–mediated cytokine storm [7, 22], and few studies have addressed innate sources of cytokines involved in cytokine storm. IFN-γ, TNF-α, and IL-10, for example, can have numerous sources, including T cells, NK cells, innate lymphoid cells (ILCs), monocytes, and dendritic cells [23–25].
From the various innate sources of IFN-γ in dengue, NK cells have been the most studied. NK cells are an important mechanism of rapid pathogen control and IFN-γ production in viral infectious diseases [26]. However, their role in dengue is still controversial. Their activation and ability to migrate to peripheral infection sites have been demonstrated in acute DENV infection [27]. However, while some studies indicate beneficial association of these cells and disease evolution in humans [28–30] and in humanized animal models of the disease [31], others report that activation of these cells is associated with increased viremia and poor prognosis of dengue infection [8, 32, 33].
Besides NK cells, type 1 ILC (ILC1) is considered another major innate source of IFN-γ. ILCs are novel lymphocytes that mirror T-helper cell polarization profiles, which are ILC1 that produce IFN-γ; ILC2 that produce type 2 cytokines, such as IL-5 and IL-13; ILC3 producing IL-17 and/or IL-22; and ILC10 (or ILCreg) that produce IL-10 [34]. Interestingly, it has been reported that TNF-α can be produced by human ILC1, ILC2, and ILC3, while IL-10 can also be produced by ILC1 and ILC2 [24]. ILCs are mostly tissue-resident cells, being detected in peripheral blood at low frequencies [34]. In response to local tissue damage, inflammation, dysbiosis and pathogenic infection, these cells rapidly produce proinflammatory or regulatory mediators, actively contributing to the development of an appropriate immune response and potentiating the formation of the adaptive immune response [35]. Importantly, uncontrolled activation of ILCs can contribute to pathology development and to tissue-damaging inflammation [36], but its role in DENV infection has never been evaluated.
In this report, we evaluate the role of innate sources in peripheral blood of IFN-γ, TNF-α, IL-10, and other inflammatory cytokines during the first 7 days of fever onset in dengue patients with focus on ILCs and NK cells, which were found to be important sources of these cytokines. However, although individuals with DWS+ and SD presented a high level of activation of ILC1, especially in production of IFN-γ, patients with DWS– displayed higher activation of NK cells.
MATERIALS AND METHODS
Ethical Statement
The study was reviewed and approved by the Research Ethics Committee of Universidade Federal de Minas Gerais (CAAE: 24832513.4.0000.5149). All participants signed a consent form prior to their enrollment in the study. Outpatients were recruited at Primary Care Center Jardim Montanhês in Belo Horizonte, Minas Gerais, and at Hospital de Base of Faculdade de Medicina de São José do Rio Preto (FAMERP), in São José do Rio Preto, São Paulo. Inpatients were recruited in Hospital de Base of FAMERP. Healthy volunteers were recruited in the community.
Clinical Information, Sample Collection, and Processing
Clinical classification between different clinical forms of dengue was performed according to World Health Organization (WHO) guidelines, at the time of consent, by the attending physician, who was also responsible for collecting symptoms and reporting evolution. Dengue cases were classified in this study into 2 groups: (1) dengue without warning signs (outpatients) (DWS–), cases of mild dengue treated at home; and (2) dengue with warning signs (DWS+) combined with severe dengue (SD) patients, who were treated at the hospital. Volunteers donated blood (40–60 mL) in heparinized vacuum tubes in the period of 2–7 days after fever onset. On the same day of collection, plasma, and peripheral blood mononuclear cells (PBMCs) were separated by centrifugation using Ficoll-Hypaque gradient (Sigma, St Louis, Missouri). Plasma samples were stored at –80°C until use. Cells were frozen in fetal bovine serum (FBS; Cultilab, Campinas, Brazil) in the presence of 10% dimethyl sulfoxide (Sigma) at –80°C for 24 hours, and then transferred to liquid nitrogen (–196°C) until use.
Dengue Diagnostics, Inclusion Criteria, and Secondary Infection
Dengue infections were investigated using the SERION ELISA classic dengue virus immunoglobulin M (IgM) and immunoglobulin G (IgG) assays, which were kindly donated by Serion Brasil (Pinhais, Brazil) and Institute Virion/Serion GmbH (Wurzburg, Germany). All the tests were performed according to the manufacturer’s instructions, including classification of primary/secondary dengue infection. Individuals were included in this study if IgM positive for dengue. Healthy controls were symptoms-free and negative for dengue-specific IgM. Individuals with negative or indeterminate/low (according to manufacturer’s instructions) IgG levels in the first 7 days of infection were considered as having primary dengue and individuals with high dengue-specific IgG during the first 7 days of symptoms were considered to be preexposed and as having secondary infection.
Immunophenotyping and Intracellular Cytokine Assessment
PBMCs were thawed in RPMI 1640 (Sigma-Aldrich) containing 10% FBS and 20 U/mL benzonase nuclease (Novagen, Madison, Wisconsin), washed in phosphate-buffered saline (PBS), and cultured at the concentration of 1 × 106 cells/well. After a period of ambience of 2 hours in a humidified incubator with 5% CO2 at 37°C, cells were incubated with brefeldin A (1 µg/mL; BioLegend) for 10 hours. Positive control wells were stimulated with phorbol myristate acetate (PMA) and ionomycin at a final concentration of 50 nM and 1 µM, respectively. After the incubation period, cells were washed in PBS and stained with Violet Live/Dead (Invitrogen) according to the manufacturer’s instructions, and subsequently stained with 4 different antibody panels (Supplementary Table 1). After incubation at 4ºC in the dark for 20 minutes, cells were washed, fixed, and permeabilized using FoxP3 staining buffer Set (eBioscience, San Diego, California) according to the manufacturer’s instructions. Cells were then incubated for 45 minutes at 4ºC with intracellular antibodies (Supplementary Table 1). Then they were washed, resuspended in Fluorescence-activated cell sorting buffer (PBS/FBS 1%), and acquired on an LSR-FORTESSA (BD).
Analyses of Subpopulations and Cytokine Production
Data from cell acquisition were analyzed using Flow Jo X program (LLC, Ashland, Oregon). Subpopulations of NK cells and ILCs were selected according to the panel’s strategy. Panel 1: NK cells were defined as Lin–Eomes+ID2– and ILCs as Lin–Eomes–ID2+CD127+. Panels 2–4: NK cells were defined as Lin–CD56+CD127– and ILCs as Lin–CD56–CD127+. Then from the NK cells and ILCs subpopulations, staining of cytokines (IFN-γ, TNF-α, IL-10, IL-17A, or IL-4/IL-13) or transcription factors (Tbet, GATA3, or RORgT) were determined. Samples with <20 000 events on Lin–ViViD–CD3– gate were excluded from analysis.
Statistical Analysis
Statistical analysis was performed using GraphPad Prism version 6.05 software. The results were analyzed using Kruskal-Wallis and Mann-Whitney tests as appropriate. The differences were considered statistically significant when P ≤ .05.
RESULTS
Study Population
Dengue patients (n = 27) were recruited during the 2019 epidemic (between February and June) in Belo Horizonte and São José do Rio Preto, Brazil, and were grouped into dengue without warning signs (DWS–), that is, patients who were treated at home, and dengue with warning signs (DWS+), which combined SD cases, including inpatient cases that met WHO [2] and Brazilian Ministry of Health [37] criteria for hospitalization. The blood was collected 2–7 days after symptoms onset. Demographic characteristics, immunological status (primary or secondary dengue), and days after symptoms onset are shown in Table 1. Groups were comparable for age and sex distribution. The proportion of individuals presenting with secondary dengue infection was significantly different between the DWS– and DWS+ groups (61% vs 87%, P ≤ .001). Mean days after symptoms onset was similar between the groups (5.1 and 6.3 days for DWS– and DWS+, respectively).
Demographics and Laboratory Characteristics of Study Population From Control, Mild Dengue (Outpatients), and Hemorrhagic Dengue Groups During Seasonal Transmission, 2019
Characteristic . | Control (n = 10) . | Mild Dengue (DWS–) (n = 13) . | Hemorrhagic Dengue (DWS+) (n = 14) . |
---|---|---|---|
Agea | 36.5 (23–58) | 44.6 (23–70) | 39.6 (25–53) |
Female sex | 60% (6/10) | 54% (7/13) | 57% (8/14) |
Anti-DENV ELISA (IgM) | 0% (0/10) | 100% (13/13) | 100% (14/14) |
Anti-DENV ELISA (IgG) | NA | 61% (8/13) | 87% (12/14) |
Days after symptom onseta | NA | 5.1 (2–7) | 6.2 (3–7) |
Characteristic . | Control (n = 10) . | Mild Dengue (DWS–) (n = 13) . | Hemorrhagic Dengue (DWS+) (n = 14) . |
---|---|---|---|
Agea | 36.5 (23–58) | 44.6 (23–70) | 39.6 (25–53) |
Female sex | 60% (6/10) | 54% (7/13) | 57% (8/14) |
Anti-DENV ELISA (IgM) | 0% (0/10) | 100% (13/13) | 100% (14/14) |
Anti-DENV ELISA (IgG) | NA | 61% (8/13) | 87% (12/14) |
Days after symptom onseta | NA | 5.1 (2–7) | 6.2 (3–7) |
Abbreviations: DENV, dengue virus; DWS–, mild dengue without warning signs; DWS+, dengue with warning signs or severe clinical presentation; ELISA, enzyme-linked immunosorbent assay; IgG, immunoglobulin G; IgM, immunoglobulin M; NA, not available.
aGeometric mean (min–max).
Demographics and Laboratory Characteristics of Study Population From Control, Mild Dengue (Outpatients), and Hemorrhagic Dengue Groups During Seasonal Transmission, 2019
Characteristic . | Control (n = 10) . | Mild Dengue (DWS–) (n = 13) . | Hemorrhagic Dengue (DWS+) (n = 14) . |
---|---|---|---|
Agea | 36.5 (23–58) | 44.6 (23–70) | 39.6 (25–53) |
Female sex | 60% (6/10) | 54% (7/13) | 57% (8/14) |
Anti-DENV ELISA (IgM) | 0% (0/10) | 100% (13/13) | 100% (14/14) |
Anti-DENV ELISA (IgG) | NA | 61% (8/13) | 87% (12/14) |
Days after symptom onseta | NA | 5.1 (2–7) | 6.2 (3–7) |
Characteristic . | Control (n = 10) . | Mild Dengue (DWS–) (n = 13) . | Hemorrhagic Dengue (DWS+) (n = 14) . |
---|---|---|---|
Agea | 36.5 (23–58) | 44.6 (23–70) | 39.6 (25–53) |
Female sex | 60% (6/10) | 54% (7/13) | 57% (8/14) |
Anti-DENV ELISA (IgM) | 0% (0/10) | 100% (13/13) | 100% (14/14) |
Anti-DENV ELISA (IgG) | NA | 61% (8/13) | 87% (12/14) |
Days after symptom onseta | NA | 5.1 (2–7) | 6.2 (3–7) |
Abbreviations: DENV, dengue virus; DWS–, mild dengue without warning signs; DWS+, dengue with warning signs or severe clinical presentation; ELISA, enzyme-linked immunosorbent assay; IgG, immunoglobulin G; IgM, immunoglobulin M; NA, not available.
aGeometric mean (min–max).
Individuals with dengue presented similar levels of classical symptoms such as fever, body pain, headache, retro-orbital pain, exanthema, asthenia, vomiting, and diarrhea (Table 2). Patients with DWS+ presented higher frequencies of mucosal bleeding (53% vs 0%, P = .005) and (abrupt) drop in platelets/thrombocytopenia (84% vs 15%, P = .001) compared with DWS– (Table 2).
Symptoms . | DWS– (n = 13) . | DWS+ (n = 14) . |
---|---|---|
Fever | 84% (11/13) | 79% (11/14) |
Body pain | 92% (12/13) | 71% (10/14) |
Headache | 23% (3/13) | 36% (5/14) |
Retro-orbital pain | 23% (3/13) | 29% (4/14) |
Exanthema | 38% (5/13) | 21% (3/14) |
Asthenia | 38% (5/13) | 21% (3/14) |
Vomiting/diarrhea | 23% (3/13) | 14% (2/14) |
Pleural effusion | 0% (0/13) | 21% (3/14) |
Abdominal pain | 7% (1/13) | 36% (5/14) |
Vertigo/fainting | 7% (1/13) | 36% (5/14) |
Mucosal bleeding | 0% (0/13) | 50% (7/14)a |
Thrombocytopenia (including abrupt drop in platelets) | 15% (2/13) | 86% (12/14)a |
Symptoms . | DWS– (n = 13) . | DWS+ (n = 14) . |
---|---|---|
Fever | 84% (11/13) | 79% (11/14) |
Body pain | 92% (12/13) | 71% (10/14) |
Headache | 23% (3/13) | 36% (5/14) |
Retro-orbital pain | 23% (3/13) | 29% (4/14) |
Exanthema | 38% (5/13) | 21% (3/14) |
Asthenia | 38% (5/13) | 21% (3/14) |
Vomiting/diarrhea | 23% (3/13) | 14% (2/14) |
Pleural effusion | 0% (0/13) | 21% (3/14) |
Abdominal pain | 7% (1/13) | 36% (5/14) |
Vertigo/fainting | 7% (1/13) | 36% (5/14) |
Mucosal bleeding | 0% (0/13) | 50% (7/14)a |
Thrombocytopenia (including abrupt drop in platelets) | 15% (2/13) | 86% (12/14)a |
Abbreviations: DWS–, mild dengue without warning signs; DWS+, dengue with warning signs or severe clinical presentation.
aFisher test, P < .005.
Symptoms . | DWS– (n = 13) . | DWS+ (n = 14) . |
---|---|---|
Fever | 84% (11/13) | 79% (11/14) |
Body pain | 92% (12/13) | 71% (10/14) |
Headache | 23% (3/13) | 36% (5/14) |
Retro-orbital pain | 23% (3/13) | 29% (4/14) |
Exanthema | 38% (5/13) | 21% (3/14) |
Asthenia | 38% (5/13) | 21% (3/14) |
Vomiting/diarrhea | 23% (3/13) | 14% (2/14) |
Pleural effusion | 0% (0/13) | 21% (3/14) |
Abdominal pain | 7% (1/13) | 36% (5/14) |
Vertigo/fainting | 7% (1/13) | 36% (5/14) |
Mucosal bleeding | 0% (0/13) | 50% (7/14)a |
Thrombocytopenia (including abrupt drop in platelets) | 15% (2/13) | 86% (12/14)a |
Symptoms . | DWS– (n = 13) . | DWS+ (n = 14) . |
---|---|---|
Fever | 84% (11/13) | 79% (11/14) |
Body pain | 92% (12/13) | 71% (10/14) |
Headache | 23% (3/13) | 36% (5/14) |
Retro-orbital pain | 23% (3/13) | 29% (4/14) |
Exanthema | 38% (5/13) | 21% (3/14) |
Asthenia | 38% (5/13) | 21% (3/14) |
Vomiting/diarrhea | 23% (3/13) | 14% (2/14) |
Pleural effusion | 0% (0/13) | 21% (3/14) |
Abdominal pain | 7% (1/13) | 36% (5/14) |
Vertigo/fainting | 7% (1/13) | 36% (5/14) |
Mucosal bleeding | 0% (0/13) | 50% (7/14)a |
Thrombocytopenia (including abrupt drop in platelets) | 15% (2/13) | 86% (12/14)a |
Abbreviations: DWS–, mild dengue without warning signs; DWS+, dengue with warning signs or severe clinical presentation.
aFisher test, P < .005.
Innate Lymphocytes Compose an Important Source of IFN-γ in Early Stages of DENV Infection
IFN-γ is considered a key cytokine in antiviral immunity [38]. Many studies have investigated T lymphocytes as cellular sources of IFN-γ during dengue infection, but curiously, this cytokine can be found in circulation very early during DENV infection, even before the onset of clinical symptoms [12], suggesting an innate source for its production. We investigated innate sources of IFN-γ production during the first week of DENV infection in the DWS– and DWS+ groups. Cells were cultured in medium only to evaluate spontaneous production of IFN-γ and other inflammatory cytokines, or stimulated with PMA/ionomycin as positive controls. Although not stimulated with specific antigen, we found small levels of spontaneous IFN-γ production by lymphocytes in PBMCs of both controls and DENV-infected individuals, which showed 2–3 times higher frequencies of cytokine production than the controls, independent of the clinical phenotype (Figure 1A and 1B). Interestingly, most of the nonstimulated spontaneous IFN-γ–producing lymphocytes in DENV-infected groups were CD3– cells, as opposed to healthy individuals, which showed a background level of IFN-γ–positive population mostly composed of CD3+ T cells (Figure 1A and 1C). We then evaluated the expression of Eomes and ID2, transcription factors that are used to differentiate NK cells from ILCs [39, 40]. While mature NK cells will display the expression of Eomes, ILCs express ID2, which is negative for Eomes expression [41, 42]. We noticed that expression of Eomes and ID2 was mostly exclusive between circulating CD3– IFN-γ + lymphocytes (Figure 1A). Surprisingly, most of the Lin– (ViVid, CD11c, FcεR1, CD19, CD14) CD3– IFN-γ + population in the lymphocyte gate was negative for Eomes and ID2 (Figure 1A). Between Eomes+ ID2– NK cells and Eomes–ID2+ ILC1s, we noticed that that IFN-γ–producing ILCs were more frequent in the DWS+ group (Figure 1D) compared with DWS– patients, whereas individuals who developed DWS– presented higher frequencies of IFN-γ + NK cells (Figure 1D) compared with their DWS+ counterparts. There was no difference in unstimulated spontaneous IFN-γ production in CD3+ lymphocytes between DWS– and DWS+, as we have previously observed [16].
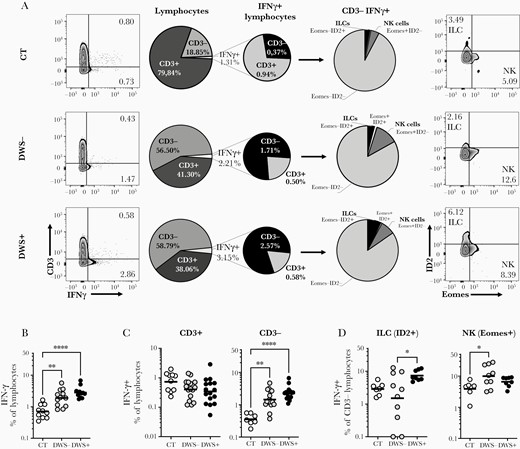
Innate lymphocytes compose an important source of interferon gamma (IFN-γ) in early stages of dengue virus (DENV) infection. Peripheral blood mononuclear cells from healthy volunteers (CT) (n = 10) and DENV-infected patients without warning signs (DWS–; n = 13) or with warning signs or severe clinical presentation (DWS+; n = 14) were evaluated by flow cytometry for spontaneous IFN-γ production during the early phase of infection (2–7 days after fever onset). Frequencies of IFN-γ–producing lymphocytes were evaluated in Lin–CD45+ gated cells (A and B) (antibody panel 1). Evaluation of IFN-γ production by CD3+ and CD3– cells was performed in lymphocyte gated cells (A and C). Production of IFN-γ by innate lymphoid cells (ILCs) and natural killer (NK) cells was evaluated in CD3– gated cells (A and D). B–D, Symbols represent single individuals and horizontal bars the geometric mean. C, Bars represent Geometric Means. Differences between groups were analyzed by Kruskal-Wallis test: *P ≤ .05; **P ≤ .01; ***P ≤ .001.
DWS– and DWS+ Patients Present Differences in Cytokine Sources Among Innate Lymphocytes
Since Eomes and ID2 may not fully mark IFN-γ–producing NK cells [43] and ILCs, we decided to use CD127 and CD56 to differentiate these cells (antibody panel 2) and gain further insight about their contribution as a source of IFN-γ during DENV infection. Our data indicate the absence of significant variation in PBMCs of total frequency of Lin–CD56–CD127+ ILCs, or Lin–CD56+CD127– NK cells during dengue infection compared with the healthy control group (Figure 2A and 2B). For example, ILCs showed levels of 5.1% (95% confidence interval [CI], 4.3%–5.9%), 4.4 (CI, 3.6%–5.4%), and 4.7 (CI, 3.7%–6.0%) in the lymphocyte gates in healthy controls, DWS–, and DWS+ individuals, respectively. For NK cells, we observed 2.9% (CI, 1.7%–5.0%), 1.1% (CI, .7%–1.9%), and 1.0% (CI, .6%–1.9%) in healthy controls, DWS–, and DWS+ individuals, respectively (Figure 2B). Because our Lin– in this panel have anti-CD16, which can exclude CD16+ NK cells, we compared these frequencies with those obtained using Eomes+ NK cells (Supplementary Figure 2A). We observed that for ILCs, no major differences were obtained using Lin–CD56–CD127+ cells (Supplementary Figure 2A). However, for NK cells, we obtained 20.3% (CI, 16%–25%), 16.2% (11%–23%), and 11% (CI, 6.9%–17%) for healthy donors, DWS–, and DWS+ patients, respectively (Supplementary Figure 2A). These frequencies are closer to those found by others [27] for blood NK cell levels. Nevertheless, our data show that both Eomes+ NK or CD56+CD16– NK cells, as well as ILCs, are able to produce IFN-γ in early stages of DENV infection when compared to the healthy control group (Figure 2C). However, in contrast to what was observed when NK cells were identified by Eomes expression, CD56+ NK cells showed similar frequencies when comparing the DWS– and DWS+ groups (Figure 2C and 2D). On the other hand, ILCs showed similar results to ID2+ cells with more activated IFN-γ–producing ILCs identified in the DWS+ group (Figure 2C), which also showed higher Tbet+ ILCs (Supplementary Figure 2B). Not only was the frequency of IFN-γ + ILCs increased in DWS+, but this group also displayed higher production per cell when compared to DWS– patients as evaluated by median fluorescence intensity (600.7 vs 801.2, P < .05) (Figure 2C).
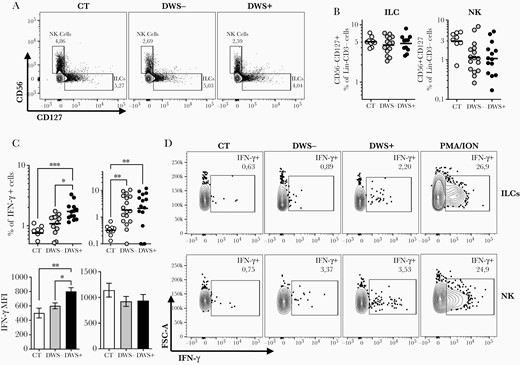
Innate lymphoid cells (ILCs) are an important source of interferon gamma (IFN-γ) in dengue virus (DENV)–infected individuals with warning signs or severe clinical presentation (DWS+) in the early stages of DENV infection. Peripheral blood mononuclear cells from healthy volunteers (n = 10), patients with mild dengue without warning signs (n = 9–13), and DWS+ patients (n = 10–14) were evaluated by flow cytometry for frequency and for spontaneous IFN-γ production (antibody panel 2) during the early phase of infection (2–7 days after fever onset). Frequencies of ILCs and natural killer (NK) cells were evaluated in Lin–CD45+CD3– gated cells according to expression of CD56 and CD127 markers as shown in representative dot plots (A) and graphs (B). C, Frequency of ILCs and NK cells producing IFN-γ and median fluorescence intensity of this cytokine production are shown. Representative dot plots of ILCs and NK cells producing IFN-γ are shown for each group of volunteers and for positive controls stimulated with phorbol myristate acetate (PMA)/ionomycin (ION) (D). Symbols in B and C represent single individuals and the horizontal bars represent the geometric mean. Differences between groups were analyzed by Kruskal-Wallis test: *P ≤ .05; **P ≤ .01; ***P ≤ .001.
To obtain a broader picture of the phenotype of ILCs and NK cells during DENV infection, we evaluated their spontaneous production of other inflammatory signature cytokines such as TNF-α, IL-10, IL-17, and IL-4/IL-13. Interestingly, DWS+ patients also displayed increased frequencies of innate lymphocytes producing other cytokines. For example, ILCs producing IL-4/IL-13 (Figure 3B) or GATA3+ (Supplementary Figure 2B) were also more frequent in DWS+ patients than DWS– individuals, whereas NK cells of the DWS+ group produced higher frequencies of IL-17 when compared to the DWS– group (Figure 3G). Although we were able to identify increased production of TNF-α (DWS– and DWS+) (Figure 3A) and IL-17 (DWS+) (Figure 3C) by ILCs of dengue patients when compared to healthy controls (representative gates of healthy controls are shown in Supplementary Figure 3), their frequencies were similar between the clinical forms. Similarly, frequencies of CD56+CD16– NK cells producing TNF-α, IL-4/IL-13, and IL-10 were not different between the DWS– and DWS+ groups (Figure 3).
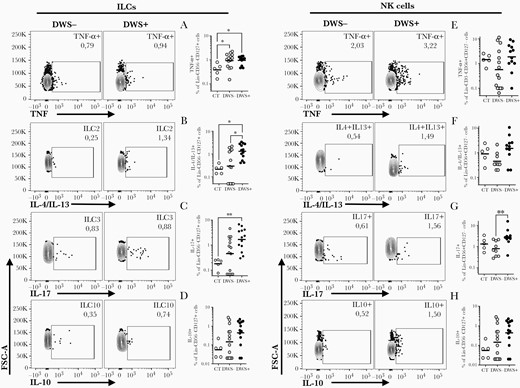
Innate lymphocytes are an important source of proinflammatory cytokines during dengue virus infection. Peripheral blood mononuclear cells from healthy volunteers (CT) (n = 5), patients with mild dengue without warning signs (DWS–; n = 9–13), and patients with dengue with warning signs or severe clinical presentation (DWS+; n = 10–14) were evaluated by flow cytometry for spontaneous tumor necrosis factor alpha (TNF-α) (A and E), interleukin (IL) 4/IL-13 (B and F), IL-17 (C and G), and IL-10 (D and H) production during the early phase of infection (2–7 days after fever onset). Frequency of innate lymphoid cell (ILC) cytokine production was evaluated in Lin–CD45+CD3–CD56–CD127+ gated cells (A–D). Frequency of natural killer (NK) cells producing cytokines was evaluated in Lin–CD45+CD3–CD56+CD127– gated cells (E–H). Bars represent the geometric mean with geometric standard deviation. Differences between groups were analyzed by Mann-Whitney test: *P ≤ .05.
DISCUSSION
The source of proinflammatory cytokines found in the plasma of dengue patients has been a matter of debate. A cytokine storm in plasma of patients is commonly found in acute dengue infections [8–14], but although some maintain that T cells can be involved in the cytokine storm associated with development of severe dengue infection [4, 7], others, including us, have found that early T-cell–derived cytokines, such as IFN-γ, TNF-α, and IL-10, are associated with mild dengue [15, 16]. However, there are few reports on the role of innate immune cells on cytokine production during acute dengue infection.
The most studied innate source of IFN-γ in dengue infection is NK cells, which express high levels of proliferation marker CD69, cytotoxicity markers (CD107b), and receptors able to drive the migration of these cells to peripheral tissues during DENV infection [27, 28, 44]. We found that during acute dengue infection, the frequencies of ILCs or NK cells (ie, Lin–CD56+ CD127– or Lin–Eomes+ID2– lymphocytes) do not vary when compared to healthy controls, despite NK cells showing a trend toward decreased levels in peripheral blood, which we speculate might be associated to peripheral tissue migration. More importantly, we found that NK cells are activated during acute DENV infection as assessed by IFN-γ production regardless of the strategy used to identify these cells. It has been shown that NK cells from DENV patients are able to produce IFN-γ when directly stimulated with IL-12 and IL-18 [27, 44] or by antibody-dependent cell cytotoxicity mechanisms [45] or when stimulated by DENV-infected monocyte-derived dendritic cells [31].
Because it is apparent that cytokines like IFN-γ, TNF-α, and IL-10, and other inflammatory markers, are increased during DENV infection even before fever onset (ie, probably earlier than T-cell activation [12]), we investigated additional sources of IFN-γ, TNF-α, and IL-10 during DENV infection in the innate immune system, focusing on ILCs and NK cells. Although it is expected that most of the spontaneous production of cytokines by innate immune cells will be in the infected tissues, we could observe an important increase in IFN-γ–producing ILC and NK cells in the peripheral blood of individuals acutely infected with DENV.
We initially used the transcription factors Eomes and ID2 to identify NK cells and ILCs, respectively. It has been reported that although both cells rely on ID2 for differentiation [42], only NK cells express Eomes [41, 43]. We found that ID2 was not expressed in mature human circulating Eomes+ NK cells, but the expression of ID2 and Eomes seemed mutually exclusive in CD3– lymphocytes. Congruently, Male et al have found that mature NK cells express messenger RNA for ID2 at very low levels when compared to Eomes [46]. CD3–Eomes+ cells producing IFN-γ were found to be increased in individuals with DWS– in our cohort. Interestingly, this result could not be replicated when NK cells were identified in the CD56+CD127– population, perhaps associated with differences in gating and panels strategies used in these 2 datasets. While in CD56+CD127– NK cells, CD16+ NK cells were excluded because it was one of the Lin– markers (antibody panel 2), the Eomes+ NK cells included CD16+ and CD16– NK subpopulations (antibody panel 1). This limitation might have caused a difference in our percentages, which are close to those found by Zimmer et al for blood of total NK cells (when we used Eomes marker) or those to CD56bright NK cells (when we used CD56 marker in the Lin– subpopulation) [27]. Interestingly, both CD56dimCD16+ and CD56brightCD16– are activated similarly during DENV infection [27], which is in line with our findings. In addition, variances in functionality associated with different levels of CD56 and Eomes by NK cells may also help explain our findings in IFN-γ production between DWS– and DWS+ patients. For example, Eomes can be poorly expressed in NK cells expressing high levels of Tbet, especially in the CD56dim population [47]. Additionally, the use of CD56 to label NK cells might contaminate the analysis with possible transitioning NK to ILC1 cells, which decreased Eomes expression while still expressing some CD56 [48]. Most important, the finding that Eomes+ NK cells are associated with favorable evolution of DENV infection is in agreement with previous findings that showed that NK cells are more activated in mild cases of dengue [28] and that they are key early producers of IFN-γ in a humanized mouse model of DENV infection [31].
We also described an important production of IFN-γ by ILCs, especially in individuals with DWS+, that also expressed higher frequencies of Tbet+ ILCs, which is consistent with the findings that this cytokine may be increased in patients with severe dengue [9, 10, 12]. Early IFN-γ production by ILCs have been implicated as an important viral control mechanism until the adaptive immune system is properly activated in mouse cytomegalovirus infection [49]. Interestingly, both DWS– and DWS+ are able to control DENV replication; however, the adaptive immunity seems less active during DENV infection in DWS+, as measured by DENV-specific IFN-γ production [15, 16]. This observation led us to speculate that innate immunity might be hyperactivated for longer periods in DWS+ to counter the infection. Similarly, we found that not only ILC1s are more activated in patients with DWS+, but also ILC2 (both IL-4/IL-13+ and GATA3+ ILCs) and NK cells producing IL-17, which can indicate higher weight on innate immune responses when compared to individuals with DWS–. It does not mean that T cells may not play a role in cytokine storm or severe dengue evolution, but our data highlight an underappreciated role to NK cells and ILCs as cytokine sources in acute dengue, especially in DWS+ or SD cases.
Our findings suggest that different innate immune sources of IFN-γ might be associated with different clinical evolution of DENV infection. We have found that individuals developing DWS– have Eomes+ NK cells as important source of early IFN-γ. On the other hand, individuals developing DWS+ present ILCs as a critical innate immune IFN-γ source, but also present signs of immune dysregulation, such as increased peripheral levels of ILC2 and NK cells producing IL-17. These results provide important insights to understand the dynamics of the immune response in dengue physiopathology.
Supplementary Data
Supplementary materials are available at The Journal of Infectious Diseases online. Supplementary materials consist of data provided by the author that are published to benefit the reader. The posted materials are not copyedited. The contents of all supplementary data are the sole responsibility of the authors. Questions or messages regarding errors should be addressed to the author.
Table S1: Antibody panels combination.
Figure S1. Flow Cytometry Gate Strategy.
Figure S2: ILC1 (Tbet+) and ILC2 (GATA3+) is increased in frequency in DWS+ when compared to DWS- patients.
Figure S3: Representative gates of healthy control donors of unstimulated cultures and PMA/Ionomycin stimulated cultures.
Notes
Acknowledgments. We are grateful to study participants and healthy volunteers for their participation. We thank Ana Beatriz Ribeiro de Queiroz, Lorena Junia de Souza Santos, and Adriano Sabino for flow cytometry technical assistance. I. L. Q.-C. is a graduate student of the Programa de Pós-Graduação em Microbiologia, and M. H. G.-P. and M. F. R. are graduate students of the Programa de Pós-Graduação em Bioquímica e Imunologia from Universidade Federal de Minas Gerais. H. C. S. and M. L. N. are Conselho Nacional de Desenvolvimento Científico e Tecnológico (CNPq) fellows. We are thankful to Ester Roffe for critical reading of the manuscript.
Financial support. This work was supported by the Conselho Nacional de Desenvolvimento Científico e Tecnológico (404138/2012-8), Fundação de Amparo a Pesquisa de Minas Gerais (APQ-03452-13); Fundação de Apoio a Pesquisa do Estado de São Paulo (13/21719-3); Instituto Nacional de Ciência e Tecnologia em Vacinas (INCT-V); the Program for Technological Development in Tools for Health (PDTIS-FIOCRUZ, Flow Cytometry Platform); and the Flow Cytometry core facility of the Pharmacy School of Universidade Federal de Minas Gerais.
Potential conflicts of interest. All authors: No reported conflicts of interest.
All authors have submitted the ICMJE Form for Disclosure of Potential Conflicts of Interest. Conflicts that the editors consider relevant to the content of the manuscript have been disclosed.