-
PDF
- Split View
-
Views
-
Cite
Cite
Leon G Leanse, Carolina dos Anjos, Ying Wang, Clinton K Murray, David C Hooper, Tianhong Dai, Effective Treatment of Cutaneous Mold Infections by Antimicrobial Blue Light That Is Potentiated by Quinine, The Journal of Infectious Diseases, Volume 224, Issue 6, 15 September 2021, Pages 1069–1076, https://doi.org/10.1093/infdis/jiab058
- Share Icon Share
Abstract
Cutaneous mold infections commonly result from an array of traumatic injuries that involve direct inoculation of contaminated soil into wounds. Here, we explored the use of antimicrobial blue light (aBL; 405 nm wavelength) and the combination of aBL with quinine hydrochloride (aBL + Q-HCL) for the treatment of cutaneous mold infections.
Efficacy of aBL and aBL + Q-HCL in killing clinically important pathogenic molds (Aspergillus fumigatus, Aspergillus flavus, and Fusarium oxyprorum) was investigated. Ultraperformance liquid chromatography identified and quantified endogenous porphyrins in the mold conidia. Finally, a mouse model of dermabrasion wound infected with a bioluminescent variant of A. fumigatus was developed to investigate the efficacy of aBL in treating cutaneous mold infections.
We demonstrated that mold conidia are tolerant to aBL, but Q-HCL enhances efficacy. Transmission electron microscopy revealed intracellular damage by aBL. aBL + Q-HCL resulted in intracellular and cell wall damage. Porphyrins were observed in all mold strains, with A. fumigatus having the highest concentration. aBL and aBL + Q-HCL effectively reduced the burden of A. fumigatus within an established dermabrasion infection and limited recurrence posttreatment.
aBL and aBL + Q-HCL may offer a novel approach for the treatment of mold infections.
Cutaneous mold infections result from traumatic injuries that involve direct inoculation of contaminated soil into wounds [1]. These infections are particularly common with soldiers that have sustained combat-related injuries [1]. However, trauma resulting from automotive or agricultural accidents and those arising from natural disasters are also important predisposing factors for cutaneous mold infections [2, 3]. The appropriate diagnosis of cutaneous mold infections can become challenging as the formation of necrotic tissue and/or a reduced response to conventional therapy can delay appropriate treatment [1]. This is further perpetuated by the presence of antimicrobial resistance phenotypes now emerging in fungal pathogens, which may hinder treatment [4].
Antimicrobial blue light (aBL; 405 nm wavelength) is an innovative strategy that is remarkably effective at killing a wide array of pathogenic microbes [5]. In particular, aBL has demonstrated significant efficacy against both bacterial and fungal (yeasts) species [6, 7]. While aBL has been investigated for its efficacy against a range of mold conidia in vitro, its efficacy appears limited [8]. Previous studies from our group demonstrated the adjuvant effects of the antimalarial drug quinine on aBL [9, 10], showing a significant potentiation of antimicrobial effects against both bacterial infections [9] and fungal yeast infections [10].
We hypothesized that quinine may increase the efficacy of aBL against cutaneous mold infections. Here, we evaluated the efficacy of aBL and the combination of aBL with quinine hydrochloride (aBL + Q-HCL) against mold conidia in vitro and in an established in vivo dermabrasion infection using a bioluminescent variant of Aspergillus fumigatus for real-time efficacy monitoring [11].
METHODS
Blue Light Source
Blue light irradiation was carried out using a single light emitting diode (LED) with a peak emission wavelength of 405 nm (M405L3; Thorlabs) and a full width at half maximum of 25 nm. For in vivo experiments, a collimator (SM2F32; Thorlabs) was attached to focus the light exposure to the wound. Irradiance was adjusted through manipulating the distance between the LED aperture and the target with the use of a PM100D power meter (Thorlabs).
Mold Strains and Culture Conditions
Aspergillus flavus and Fusarium oxysporum species used in the study were clinical isolates derived from infected soldiers deployed in Iraq or Afghanistan. The Aspergillus fumigatus strain used in this study was a recombinant strain transformed with a Photinus pyralis luciferase coding region [11]. Molds were cultured on malt extract agar within a T75 tissue culture flasks and incubated at 30°C for 7 days.
Killing Planktonic Conidia Using aBL or aBL + Q-HCL to Assess the Efficacy of the Treatment In Vitro
Initially, we retrieved the conidia from the T75 flask by introducing 10 mL of sterile phosphate-buffered saline (PBS) into the flask and mixing the culture with the solution using a 10-µL inoculation loop. The suspension was mixed by gentle pipetting. To separate the conidia from the hyphae, the suspension was poured through a Falcon cell strainer (40 µm pore size; Fisher Scientific) into a 50-mL Falcon tube (Fisher Scientific). Subsequently, the suspension was introduced into a hemocytometer and the numbers of conidia were quantified by light microscopy. Conidia were then adjusted to approximately 108 colony forming units (CFU)/mL in PBS. Three mL of the suspension was then transferred to a 35 × 12 mm petri dish immediately prior to aBL illumination. To achieve uniform illumination of conidia, a 3-mm magnetic stir bar was added to the suspension and spun at 20 rotations per minute. Where appropriate, Q-HCL (1 mg/mL) was added to the suspension just before aBL illumination. Different aliquots of aBL (100 mW/cm2) were exposed onto conidia: 0, 180, 360, 540, and 720 J/cm2, reflecting 0, 30, 60, 90, and 120 minutes treatment time, respectively. Following each aliquot, 40 µL of the suspension was transferred to a 96-well microtiter plate for serial dilution and plating for CFU enumeration [12].
Porphyrin Extraction for Ultraperformance Liquid Chromatography
Molds were cultured and 2 × 1010 CFU/mL of conidia were isolated as described above. The mold conidial suspension was then pelleted by centrifugation (at 13 500g for 10 minutes), the supernatant was discarded, the pellet was suspended in 1 mL of extraction solution (ethanol, dimethyl sulfoxide, acetic acid, 80:20:1; vol/vol/vol), and stored at −80°C. After 24 hours the cells were disrupted by sonication and the supernatant (containing the porphyrin extract) was recovered by centrifugation (at 13 500g for 10 minutes). For ultraperformance liquid chromatography (UPLC) analyses, the Chromatographic Marker kits (CMK-1A, Frontier Scientific and PPIX, Sigma Aldrich), which are composed of uroporphyrin I, 7-carboxylporphyrin I, 6-carboxylporphyrin I, 5-carboxylporphyrin I, coproporphyrin, mesoporphyrin IX, and mesoporphyrin IX dihydrochloride, were used as the reference compounds. Results were compared with standard porphyrins to identify the presence intermediate porphyrins. Quantitation of porphyrins by UPLC was performed on conidial porphyrin extract and carried out using a Waters Acquity UPLC system [13].
Transmission Electron Microscopy of Conidia Following aBL or aBL + Q-HCL Treatment to Identify Ultrastructural Changes
A. fumigatus was used as a representative pathogen for transmission electron microscopy (TEM). Conidia (108 CFU/mL) were treated with aBL alone (720 J/cm2), aBL + Q-HCL (720 J/cm2; 1 mg/mL), Q-HCL (1 mg/mL; 200 minutes) alone, or left untreated. These were then fixed immediately in a 2% paraformaldehyde/2.5% glutaraldehyde solution and stored for approximately 18 hours at 4°C. Subsequently, a fixed suspension was centrifuged at 4000g for 5 minutes, before washing 3 times with 0.1 M cacodylate buffer (pH 7) and suspending the pellet in the same buffer. Following fixation of the conidia, hot agar (2% in distilled water) was added to the pellets, and once solidified they were processed for TEM [9]. Sections were cut on a microtome (Reichert-Jung Ultracut E), collected on copper grids (200 mesh), and uranyl acetate and lead citrate were used as a stain. Examination of the sections was performed on a Philips CM-10 TEM.
Mouse Model of Dermabrasion Wounds Infected With A. fumigatus Conidia
We infected dermabrasion wounds [9, 10] with a bioluminescent variant of A. fumigatus to establish a cutaneous infection. We used 6-week-old female BALB/c mice weighing approximately 18 g that were procured from Charles River Laboratories. All procedures performed on animals were approved by the Institutional Animal Care and Use Committees of Massachusetts General Hospital (protocol number, 2016N000084), all of which are in accordance with National Institute of Health protocols.
Dermabrasions were produced immediately following intraperitoneal injections with a ketamine/xylazine cocktail (20–100 mg/kg). Mice were then shaved, and using a No. 15 sterile scalpel blade, a defined 1 cm × 1 cm area was carefully abraded. Subsequently, a 100-µL conidial suspension (in PBS) containing 1.7 × 109 CFU was applied to the abraded area. Mice were kept anaesthetized and housed separately in different cages until the inoculum dried. Mice were placed in animal housing for 24 hours to permit the establishment of infection.
Efficacy of aBL and aBL + Q-HCL Against Established A. fumigatus Wound Infections
Following infection, mice were subjected to the following treatments: aBL alone, aBL + Q-HCL, Q-HCL alone, a PBS vehicle control, or voriconazole. aBL (120 mW/cm2 irradiance) was exposed with radiant exposures of 0, 72, 144, 216, 288, 360, 432, 504, and 576 J/cm2. This reflected treatment times of 0, 10, 20, 30, 40, 50, 60, 70, and 80 minutes, respectively. Mice were treated with aBL in the presence or absence of topically applied Q-HCL that was applied at 0 minutes (100 μL; 0.3 mg/cm2) and at 30 minutes (100 μL; 0.3 mg/cm2), thus a total concentration of 0.6 mg/cm2 was applied. Following each treatment aliquot, mouse wounds were visualized by real-time bioluminescence imaging, which was preceded by the addition of 20 μL of d-luciferin (from a 15-mM stock solution) to catalyze bioluminescence emission. A vehicle control treated only with PBS and a positive control treated intraperitoneally with 20 mg/kg voriconazol [14] were also included.
Mice were monitored for 3 days postinfection to measure recurrence of fungal burden within wounds. Voriconazole treatment was applied once per day [14] when measuring recurrence. A total of 12 mice per group were included in the study, except for the voriconazole control, which only included 10 mice.
Bioluminescence Imaging In Vivo
The bioluminescence of molds present within mouse dermabrasion wounds was observed on an IVIS Lumina II In Vivo Imaging System (PerkinElmer). The intensity of bioluminescence intensity, released by photon emission by the molds, is indicative of the viability of the molds, which can be measured directly at the site of infection in mice using a region of interest tool. Living Image software was used to analyze bioluminescence imaging and photon counting that allows quantification of the relative luminescence units (RLU), which is in linear correlation with corresponding CFU [11], in real time.
Statistical Analyses
Data were presented as the standard error of the mean (SEM), and differences between means were compared for significance using a paired or unpaired t test. A 1-way or 2-way analysis of variance (ANOVA) with a Benjamini-Hochberg correction was applied where appropriate. P values of < .05 were considered significant.
RESULTS
Efficacy of aBL Against Mold Conidia Is Limited but Is Significantly Potentiated by Q-HCL
We evaluated the effectiveness of both aBL and aBL + Q-HCL against mold conidia from clinically relevant molds. For F. oxysporum, irradiation with 540 J/cm2 of aBL reduced the CFU by 0.94 log10. The addition of 1 mg/mL Q-HCL significantly improved the antimicrobial effects elicited by aBL, with a 6.52 log10 CFU reduction being achieved (P = .0003; Figure 1). Regarding A. flavus, aBL irradiation with 540 J/cm2 only reduced the CFU by 0.86 log10. However, the efficacy was potentiated significantly through the addition of 1 mg/mL Q-HCl, with a 5.91 log10 CFU reduction being achieved (P = .00002; Figure 1). A. fumigatus was also not very susceptible to aBL-mediated killing with a CFU reduction of 0.91 log10 CFU being observed following 540 J/cm2. The increase of illumination to 720 J/cm2, however, did increase the antimicrobial effects, resulting in a 2.44 log10 CFU reduction. The addition of 1 mg/mL Q-HCL also significantly enhanced the effects of aBL, resulting in a 2.65 log10 CFU reduction following 540 J/cm2 (P = .01; Figure 1) and a 4.00 log10 CFU reduction following 720 J/cm2. Q-HCL alone did not influence the viability of conidia.
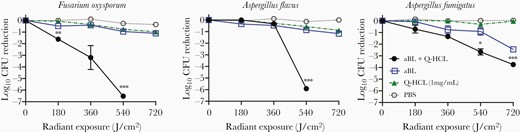
Killing kinetics of fungal conidia (Fusarium oxysporum, Aspergillus flavus, and Aspergillus fumigatus) by aBL or aBL + Q-HCL over radiant exposures of 0, 180, 360, 540, and 720 J/cm2. Statistical analyses performed were paired t test comparing aBL and aBL + Q-HCL groups. *P < .05, **P < .01, ***P < .001. Error bars, standard error of the mean. Abbreviations: aBL, antimicrobial blue light; CFU, colony forming unit; PBS, phosphate-buffered saline; Q-HCL, quinine hydrochloride.
TEM Illustrated Significant Ultrastructural Damages Following aBL and aBL + Q-HCL Exposure
We evaluated the damages to conidia that were associated with aBL or aBL + Q-HCL exposure. The untreated control section illustrated an intact cell wall, cytoplasm, and discernable organelles (Figure 2, blue arrows). Q-HCL alone resulted in vacuole formation, suggesting cytoplasmic damage (yellow arrow). Illumination with aBL resulted in destruction of the cytoplasmic material and organelles (green asterisk); however, the cell wall was left intact. The application of combined aBL and Q-HCL resulted in damage to the cytoplasmic material and the organelles, and induced significant damage to the cell wall of the conidia (red arrow).

TEM images of Aspergillus fumigatus conidia subjected to no treatment (control), Q-HCL treatment (1 mg/mL), aBL treatment (720 J/cm2), and aBL + Q-HCL treatment (720 J/cm2 + 1 mg/mL). Blue arrow, organelles; yellow arrow, vacuoles; red arrow, cell wall damage; *, organelle/cytoplasmic destruction. Bar, 500 nm. Abbreviations: aBL, antimicrobial blue light; Q-HCL, quinine hydrochloride; TEM, transmission electron microscopy.
UPLC Revealed Differential Porphyrin Concentrations Within Molds
We identified endogenous porphyrins within the different mold conidia. We found that A. fumigatus had the highest concentration of intermediate porphyrins (155.7 nmol/mg) and protoporphyrin IX (PPIX; 41.0 nmol/mg) which is the terminal porphyrin of the heme biosynthesis pathway (Figure 3A). The dominant intermediate porphyrin was uroporphyrin, which comprised 63.9% of all porphyrins, and hexaporphyrin was the only intermediate porphyrin that was not detected (Figure 3B). F. oxysporum contained only 19.5 nmol/mg of intermediate porphyrins and 0.1 nmol/mg of PPIX. Here, the dominant intermediate porphyrin was found to be coproporphyrin, comprising 80.2%. As with A. fumigatus, hexaporphyrin was not detected. A. flavus had extremely low concentration of intermediate porphyrins (2.6 nmol/mg) and the second highest PPIX concentration (26.6 nmol/mg). As with F. oxysporum, the dominant intermediate porphyrin was coproporphyrin (62.8%). As with the other species, hexaporphyrin was not detectable in A. flavus.
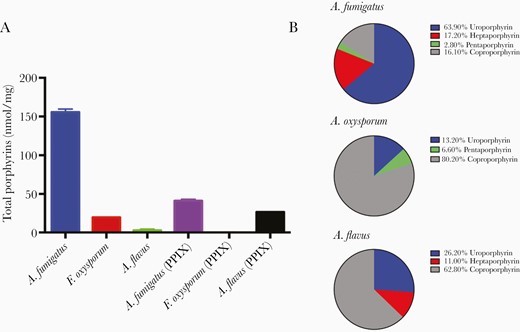
A, Porphyrin concentrations (intermediate porphyrins, PPIX) present within Aspergillus fumigatus, Fusarium oxysporum, and Aspergillus flavus. B, Percentage composition of intermediate porphyrins in A. fumigatus, F. oxysporum, and A. flavus.
aBL and aBL + Q-HCL Reduced the Burden of A. fumigatus Within a Dermabrasion Infection in Mice
We investigated the efficacy of aBL and aBL + Q-HCL for the treatment of an established cutaneous mold infection caused by A. fumigatus. With 144 J/cm2 aBL alone (20 minutes), the bioluminescence signal was reduced by 1.29 log10 RLU, reflecting a >90% reduction in the viability of the mold (Figure 4). The combined aBL + Q-HCL exposure significantly improved the killing following aBL 144 J/cm2 + Q-HCL 0.3 mg/cm2 (20 minutes) treatment, which resulted in a 2.08 log10 RLU reduction (P = .008), reflecting a >99% reduction in the viability of A. fumigatus (Figure 4). For the Q-HCL and voriconazole groups, the maximum exposure (80 minutes) resulted in RLU reductions of 0.56 and 0.91 log10, respectively (P < .03).

A, Representative bioluminescent images showing the real-time viability of Aspergillus fumigatus. B, Killing kinetics of aBL, aBL + Q-HCL, Q-HCL, voriconazole, and PBS (untreated) over 0, 72, 144, 216, 288, 360, 432, 504, and 576 J/cm2. Statistical analyses performed were the paired t test to compare aBL and aBL + Q-HCL groups. Error bars, standard error of the mean. *P < .05, **P < .01. Abbreviations: aBL, antimicrobial blue light; PBS, phosphate-buffered saline; Q-HCL, quinine hydrochloride; RLU, relative luminescence unit.
Both aBL and aBL + Q-HCL Limited Recurrence of the Cutaneous A. fumigatus Infection Following Treatment
We explored the longevity of a single exposure of aBL (576 J/cm2) and aBL (576 J/cm2) + Q-HCL (1 mg/mL) in sustaining a reduced mold burden within A. fumigatus-infected wounds over a period of 72 hours. In addition, the standard treatment, voriconazole (20 mg/kg), was administered once daily to evaluate the translatability of using aBL or aBL + Q-HCL as alternate therapeutic strategies. For the aBL-alone group, at 0 hours posttreatment the bioluminescence signal (Figure 5A) was 1.85 log10 RLU lower than the PBS control (P = .028; Figure 5B). At 24 hours posttreatment, the signal was 1.75 log10 RLU lower than the PBS control (P = .008; Figure 5B). At 48-hours post-aBL exposure, the bioluminescence signal was 0.52 log10 RLU lower than the PBS control (P = .052; Figure 5B). At 72 hours, the bioluminescent signal was like the PBS control (P = .747; Figure 5B). For the aBL + Q-HCL–treated group, at 0 hours posttreatment the bioluminescence was 2.09 log10 RLU lower than the PBS control (P = .028; Figure 5B). At 24 hours postinfection, the RLU was 2.10 log10 lower than the PBS control (P = .008; Figure 5B). Following 48 hours, the signal was 0.72 log10 RLU lower than the PBS control (P = .032; Figure 5B). As with aBL alone, there was no significant difference in the bioluminescence signal relative to the PBS control (P = .709; Figure 5B). The bioluminescence signal in the voriconazole control was not different from the PBS control at 0 hours (P = .334). Surprisingly, following 24 hours, the bioluminescence signal in the voriconazole-treated group increased by 0.94 log10 RLU (P < .0001; Figure 5B). Following 48 hours, there was a further increase in the bioluminescence signal by 0.18 log10 RLU (P < .005; Figure 5B). At 72 hours, the signal in the voriconazole group was still significantly higher than the PBS control group (P = .036; Figure 5B). With respect to the Q-HCL versus PBS, there was no significant difference at any time point tested (P > .34; Figure 5B).
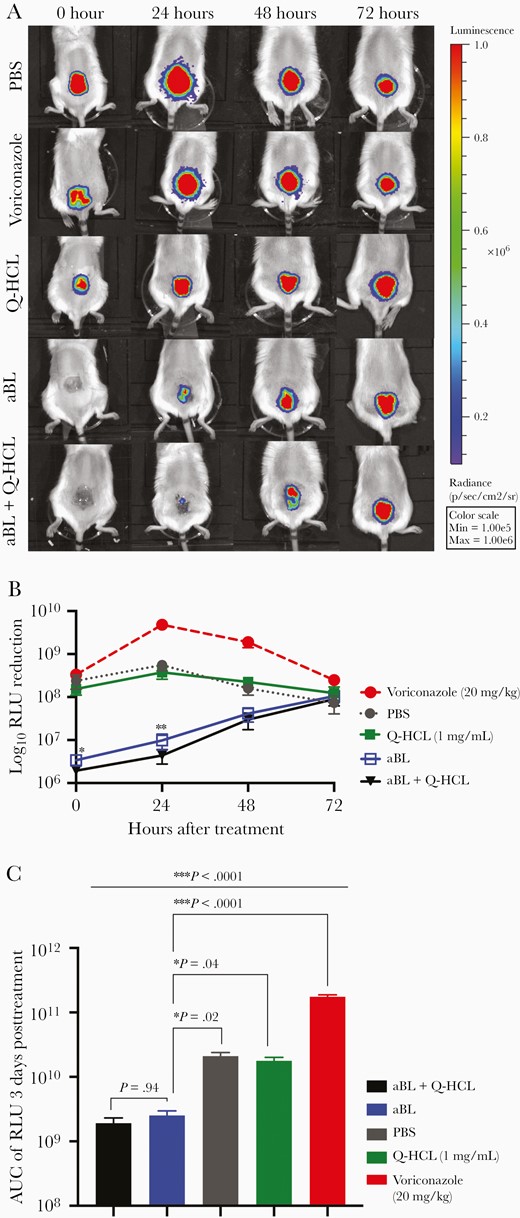
A, Representative bioluminescent images showing the real-time viability of Aspergillus fumigatus over 72 hours posttreatmen. B, Kinetics of A. fumigatus recurrence following treatment with aBL (576 J/cm2), aBL (576 J/cm2) + Q-HCL (0.6 mg/cm2), Q-HCL, voriconazole, and PBS (untreated). Statistical analyses were a 2-way ANOVA with a Benjamini-Hochberg correction that compared aBL vs aBL + Q-HCL. C, AUC of the RLU over 72 hours following treatment with aBL, aBL + Q-HCL, Q-HCL, voriconazole, and PBS (untreated). Comparison aBL with all other groups was by a 1-way ANOVA and Benjamini-Hochberg correction. Error bars, standard error of the mean. * P < .05, ** P < .01, ***P < .001. Abbreviations: aBL, antimicrobial blue light; ANOVA, analysis of variance; AUC, area under the curve; PBS, phosphate-buffered saline; Q-HCL, quinine hydrochloride; RLU, relative luminescence unit.
Area under a curve (AUC; area under the time-course curve of RLU representing the overall bioburden) analyses of the aBL-treated group revealed that over 72 hours posttreatment there was a 0.92 log10 lower AUC relative to the PBS control (P < .015; Figure 5C). With respect to aBL + Q-HCL, the AUC over 72 hours was 1.04 log10 lower than the PBS control, reflecting a 10-fold lower A. fumigatus burden relative to the PBS control (P < .013; Figure 5C). There was, however, no significant difference in AUC of the RLU over 72 hours when comparing the aBL and Q-HCL groups (P = 0.934; Figure 5C). The voriconazole-treated group had a significantly higher AUC over 72 hours when compared to the PBS control (P < .0001; Figure 5C). The AUC of the RLU over 72 hours in the Q-HCL–treated group was not significantly different from the PBS control (P = .664).
DISCUSSION
Our study investigated, for the first time, the efficacy of using aBL or aBL + Q-HCL to kill molds in vitro, and their applicability for the treatment of cutaneous infections caused by molds, using a bioluminescent variant of A. fumigatus. We found both A. flavus and F. oxysporum conidia to be highly tolerant of aBL-mediated killing, with A. fumigatus being comparatively more sensitive. Previous studies have illustrated that both bacteria [6, 13, 15] and yeasts such as Candida spp. [7, 16] are comparatively more sensitive to aBL-mediated killing than are mold conidia [8]. This is not surprising, given that fungal conidia have low metabolic activity and are extremely resilient [17]. For example, the cell wall of A. fumigatus conidia contains dihydroxynaphthalene-melanin, which contributes to its rigidity and in turn protects it from exogenous stressors, including heat and UV light [18], and thus it is intrinsically more resistant to stress-induced damage, as might be inflicted by reactive oxygen species (ROS). The reduced efficacy may additionally be explained by the porphyrin content within mold conidia. Porphyrins are key intermediate chemicals produced during heme biosynthesis and possess photosensitizing properties. When porphyrins are excited by aBL, they will lead to formation of singlet oxygen, a molecule that causes significant cellular damage [19].
While we found all species to have detectable porphyrins; the concentrations were low in both A. flavus (2.6 nmol/mg) and F. oxysporum (19.5 nmol/mg). In contrast, the concentration of porphyrins within A. fumigatus mold conidia was considerably higher (155.7 nmol/mg), which might explain why it was more susceptible to aBL killing than the other molds. However, even with respect to A. fumigatus, the concentration of porphyrins was still found to be up to 142-fold lower than the concentration detected in a highly aBL-susceptible species Neisseria gonorrhoeae [13]. More work looking at numerous mold strains is required to correlate sensitivity to aBL-mediated killing to porphyrin concentration and metabolic activity.
We found that the presence of Q-HCL significantly potentiated aBL-mediated killing in all mold species tested. This is in accordance with our previous studies, which found Q-HCL to be a powerful aBL adjuvant in gram-negative bacteria [9] and the yeast Candida albicans [10].
TEM illustrated that illumination with 720 J/cm2 of aBL against A. fumigatus conidia destroyed the cytoplasmic material as well as organelles within the conidia; however, this left the cell wall intact. Concurrent exposure of aBL and Q-HCL resulted in similar destruction of the endogenous structures and induced significant damage to the cell wall. Interestingly, the Q-HCL–only treated conidia resulted in the formation of vacuoles within the cytoplasmic materials, which may have primed further damage elicited by aBL. In our previous studies we hypothesized that the interaction of Q-HCL with heme may result in porphyrin overproduction in microbial cells, leading to increased sensitization of microbes to aBL [9]. Another study found that perturbation of the cell wall in fungi sensitized them to chloroquine [20]. It is feasible that the interaction of Q-HCL with the components of the heme biosynthesis pathway, coupled with some perturbation of the cell wall by aBL, may be responsible for this potentiation, although more work is required.
Additionally, we found that in a mouse model of cutaneous A. fumigatus infection, both aBL and aBL + Q-HCL exposure effectively reduced the burden of A. fumigatus following up to 576 J/cm2 aBL exposure, a dose previously found to not induce apoptosis in vivo [6]. Interestingly, the presence of Q-HCL potentiated killing following 144 J/cm2 (almost 10-fold improvement); however, continued exposure with aBL resulted in similar efficacy as seen with combined aBL + Q-HCL. In addition, we found both aBL and aBL + Q-HCL to limit recurrence of the infection over 24 hours and 48 hours; however, the signal was then indistinguishable from the PBS control. This suggests that, for clinical translation, frequent treatment with aBL and Q-HCL might be indicated to reduce recurrence.
Antifungal efficacy of aBL in vivo was surprisingly better than in vitro. A possible explanation is germinated conidia may be more sensitive than conidia due to the production of filaments (hyphae), which may be more susceptible to oxidative stress, thus reducing the intrinsic aBL tolerance properties of the conidia. Additionally, as conidia begin to germinate their metabolic activity increases, which may be more susceptible to ROS-mediated apoptosis [21]. More work looking at the role of metabolism in the efficacy of aBL is required.
Another surprising finding was that daily treatment with voriconazole, which is the standard treatment for A. fumigatus infection, resulted in a 10-fold increase in the A. fumigatus burden over 72 hours. Hassan et al found that utilization of clinical doses of antibiotics against Pseudomonas aeruginosa biofilms (which are tolerant to antibiotic activity) induced increased expression of virulence factors, suggesting that if the dose or duration of treatment was not sufficient, it might increase pathogenicity [22]. It is feasible that more frequent treatment with voriconazole would be required to mitigate disease progression.
CONCLUSIONS
The efficacy of aBL against mold conidia is limited; however, it can be significantly potentiated by the concurrent application of Q-HCL. Both aBL and aBL + Q-HCL effectively reduced the viability of A. fumigatus within a mature wound infection and inhibited recurrence, illustrating that this may be a novel and suitable approach to treat infections caused by molds.
Notes
Acknowledgments. We thank Drs Mattias Brock (University of Nottingham, UK) and Michael Ramm (Leibniz Institute for Natural Product Research and Infection Biology, Germany) for providing us with the bioluminescent A. fumigatus strain; and Dr Michael Mansour, Massachusetts General Hospital for his helpful suggestions relating to the establishment of cutaneous mold infections in mice.
Author contributions. L. G. L. designed the study, performed in vitro and in vivo studies, and wrote the manuscript. C. A. contributed to experimental design, performed in vitro and in vivo studies, and edited the manuscript. Y. W. contributed to in vitro studies. C. K. M. and D. C. H. contributed to the study design. T. D. designed the study and wrote the manuscript.
Financial support. The work was supported in part by the National Institute of Health (grant number R01AI123312 to T. D.); the Department of Defense (grant number FA9550-17-1-0277 to T. D.); American Society for Laser Medicine and Surgery (grant number BS.F04.18 Research Grant to L. G. L.); FAPESP—São Paulo Research Foundation (grant number 2019/10851-4 to C. A.); National Natural Science Foundation of China (grant number 62075244 to Y. W.); and China Scholarship Council (grant number 201603170004 to Y. W.).
Potential conflicts of interests. All authors: No reported conflicts of interest. All authors have submitted the ICMJE Form for Disclosure of Potential Conflicts of Interest. Conflicts that the editors consider relevant to the content of the manuscript have been disclosed.
References
Author notes
L. G. L. and C. D. A. contributed equally to the study.