-
PDF
- Split View
-
Views
-
Cite
Cite
Paulin Sonon, Maria Lúcia Brito Ferreira, Renata Santos Almeida, Neifi Hassan Saloum Deghaide, Glauco Henrique Willcox, Elizabeth Lima Guimarães, Antônio Fernando da Purificação Júnior, Marli Tenório Cordeiro, Carlos Alexandre Antunes de Brito, Maria de Fátima Militão de Albuquerque, Roberto D Lins, Eduardo A Donadi, Norma Lucena-Silva, Differential Frequencies of HLA-DRB1, DQA1, and DQB1 Alleles and Haplotypes Are Observed in the Arbovirus-Related Neurological Syndromes, The Journal of Infectious Diseases, Volume 224, Issue 3, 1 August 2021, Pages 517–525, https://doi.org/10.1093/infdis/jiaa764
- Share Icon Share
Abstract
We took advantage of the 2015–2016 Brazilian arbovirus outbreak (Zika [ZIKV]/dengue/chikungunya viruses) associated with neurological complications to type HLA-DRB1/DQA1/DQB1 variants in patients exhibiting neurological complications and in bone marrow donors from the same endemic geographical region.
DRB1/DQA1/DQB1 loci were typed using sequence-specific oligonucleotides. In silico studies were performed using X-ray resolved dimer constructions.
The DQA1*01, DQA1*05, DQB1*02, or DQB1*06 genotypes/haplotypes and DQA1/DQB1 haplotypes that encode the putative DQA1/DQB1 dimers were overrepresented in the whole group of patients and in patients exhibiting peripheral neurological spectrum disorders (PSD) or encephalitis spectrum disorders (ESD). The DRB1*04, DRB1*13, and DQA1*03 allele groups protected against arbovirus neurological manifestation, being underrepresented in whole group of patients and ESD and PSD groups. Genetic and in silico studies revealed that DQA1/DQB1 dimers (1) were primarily associated with susceptibility to arbovirus infections; (2) can bind to a broad range of ZIKV peptides (235 of 1878 peptides, primarily prM and NS2A); and (3) exhibited hydrophilic and highly positively charged grooves when compared to the DRA1/DRB1 cleft. The protective dimer (DRA1/DRB1*04) bound a limited number of ZIKV peptides (40 of 1878 peptides, primarily prM).
Protective haplotypes may recognize arbovirus peptides more specifically than susceptible haplotypes.
Arbovirus infections are primarily transmitted by mosquito-borne viruses. In Brazil, the Aedes aegypti mosquito is responsible for the transmission of dengue (DENV), Zika (ZIKV) and chikungunya (CHIKV) viruses [1]. In 2015–2016, a unique outbreak encompassing ZIKV, CHIKV, and the endemic DENV caused severe neurological complications primarily observed in infants producing an encephaly [2, 3], and among adults causing several types of neurological disorders, particularly peripheral neurological spectrum disorders (PSD) and encephalitis spectrum disorders (ESD) [3, 4].
Arbovirus gene variability may contribute to mosquito virus adaptation, enhancing infectivity, pathogenicity, fitness, and host transmissibility. In particular, residue exchange in structural or nonstructural (NS) viral proteins [5], including mutations of the NS1 (ZIKV, DENV) and envelope E-1/E-2 (CHIKV) proteins, contribute to virus adaptation to the vectors, facilitating rapid transmission [6]. In addition, a subgenomic flaviviral RNA fragment of DENV-2, which is important for viral replication, may accumulate in the mosquito salivary gland, enhancing virus transmission to the host [7].
Single nucleotide variations in human genes responsible for innate immunity have been associated with susceptibility to or severity of arboviral infections. For instance, mutations at IFNA [8], TLR (Toll-like receptors) [9], and other genes important for immune response [5] have been associated with severe arbovirus infections. Regarding adaptive immunity, histocompatibility genes are remarkably variable, encoding molecules that play a major role in virus peptide presentation as well as in virus elimination. HLA class I and II genes have been evaluated in dengue and chikungunya infections [10–13] but have not been evaluated in Zika infection.
The heterodimers encoded by HLA class II genes (DPA1/B1, DQA1/B1, and DRA1/B1) are primarily involved in antigen presentation to CD4 T cells and exhibit folded α and β chains that form the peptide binding groove. In contrast to the DRA1 chain that presents negligible residue variation, the other HLA class II α and β chains are highly variable, primarily at the peptide binding groove, as an adaptation to recognize pathogen-derived antigens to be presented to T-cell receptors. HLA-DQA1 alleles have been associated with susceptibility to flavivirus infections in Greek and Brazilian patients [12, 14]. The HLA-DQB1*03:02 and DQB1*02:02 genotypes are associated with dengue hemorrhagic fever and dengue fever, respectively [11]. HLA-A*31 and HLA-DRB1*08 allele groups conferred risk to dengue shock syndrome during the secondary infection, while patients with the HLA-A*24 and HLA-DRB1*12 allele groups are susceptible to dengue hemorrhagic fever during the primary dengue infection [15].
We took advantage of the 2015–2016 arbovirus outbreak that occurred in the northeastern state of Pernambuco to (1) follow up patients exhibiting neurological complications for at least 2 years; (2) type HLA-DQA1/DQB1/DRB1 allele groups, genotypes, and haplotypes; and (3) study in silico the interaction of arbovirus peptides with the putative DQA1/DQB1 and DRA1/DRB1 dimers formed by some of the differentially observed DRA1/DRB1 and DQA1/DQB1 haplotypes.
METHODS
Patients and Controls
Patients (n = 90, 43 men) with average age 45.75 years (SD ±16.90), presenting with neurological symptoms during the arbovirus outbreak, were recruited at Hospital da Restauração of Recife, State of Pernambuco, Brazil. Patient sera or plasma and cerebrospinal fluid were used for etiological infection diagnosis using enzyme-linked immunosorbent assay (ELISA; immunoglobulin M and immunoglobulin G), plaque reduction neutralization test, and quantitative real-time polymerase chain reaction (qRT-PCR) for ZIKV, CHIKV, and DENV [16, 17].
At the time of diagnosis, patients exhibited laboratory evidence for flavivirus (ZIKV or DENV) infections (13 patients), or togavirus (CHIKV) infection (23 patients), or flavivirus plus togavirus infections (28 patients). In the remaining 26 patients it was not possible to discriminate ZIKV from DENV infection due to the well-known serologic cross-reactivity between flaviviruses (ZIKV and DENV); however, they exhibited similar neurological manifestation as seen in confirmed ZIKV-infected patients. All patients were also categorized according to the major neurological syndrome into ESD (29 patients) and PSD (46 patients). Few cases (15 patients) exhibited other neurological complications, that is optic neuritis, peripheral facial paralysis, and brain stroke. These patients were included in the analysis of the association between HLA class II alleles with the arbovirus-induced neurological complications; however, they were excluded when we investigated the allele frequencies between the major clinical presentation forms (ESD/PSD). In parallel, we studied 200 healthy bone marrow donors (112 men) with average age 35.74 years (SD ±7.75) from the same geographical and endemic area as the patients (Table 1).
Demographic and Phenotypic Features of Control Population and Patients Infected by Arbovirus in the 2015–2016 Epidemics in the Brazilian Northeastern State of Pernambuco
Demographic and Phenotypic Features . | Patients, No. (%)a . | Controls, No. (%) . |
---|---|---|
Total participants | 90 (31.03) | 200 (68.97) |
Sex | ||
Female | 47 (16.21) | 88 (30.34) |
Male | 43 (14.83) | 112 (38.62) |
Age | ||
< Median, No. | 43 | 97 |
Median, y (No.) | 46 (2) | 34 (11) |
> Median, No. | 43 | 92 |
Patient phenotypes | ||
Infected by flavivirus, ZIKV or DENV | 39 (43.33) | … |
Infected by togavirus, CHIKV | 23 (25.56) | … |
Infected by togavirus + flavivirus | 28 (31.11) | … |
Syndromes caused by arbovirusb | ||
ESD | 29 (32.22) | … |
PSD | 46 (51.11) | … |
ONDc | 15 (16.67) |
Demographic and Phenotypic Features . | Patients, No. (%)a . | Controls, No. (%) . |
---|---|---|
Total participants | 90 (31.03) | 200 (68.97) |
Sex | ||
Female | 47 (16.21) | 88 (30.34) |
Male | 43 (14.83) | 112 (38.62) |
Age | ||
< Median, No. | 43 | 97 |
Median, y (No.) | 46 (2) | 34 (11) |
> Median, No. | 43 | 92 |
Patient phenotypes | ||
Infected by flavivirus, ZIKV or DENV | 39 (43.33) | … |
Infected by togavirus, CHIKV | 23 (25.56) | … |
Infected by togavirus + flavivirus | 28 (31.11) | … |
Syndromes caused by arbovirusb | ||
ESD | 29 (32.22) | … |
PSD | 46 (51.11) | … |
ONDc | 15 (16.67) |
Abbreviations: CHIKV, chikungunya virus; DENV, dengue virus; ESD, encephalitis spectrum disorders; OND, other neurological disorders; PSD, peripheral spectrum disorders; ZIKV, Zika virus.
aPatients infected by arboviruses ZIKV, DENV, and/or CHIKV.
bESD: cerebellitis, encephalitis, meningoencephalitis, rhombencephalitis, myelitis, and myeloradiculitis; and PSD: polyneuropathy, polyradiculoneuropathy, radiculoneuropathy, and Guillain-Barré syndrome.
cA few patients were also diagnosed with OND, including optic neuritis, peripheral facial paralysis, and brain stroke.
Demographic and Phenotypic Features of Control Population and Patients Infected by Arbovirus in the 2015–2016 Epidemics in the Brazilian Northeastern State of Pernambuco
Demographic and Phenotypic Features . | Patients, No. (%)a . | Controls, No. (%) . |
---|---|---|
Total participants | 90 (31.03) | 200 (68.97) |
Sex | ||
Female | 47 (16.21) | 88 (30.34) |
Male | 43 (14.83) | 112 (38.62) |
Age | ||
< Median, No. | 43 | 97 |
Median, y (No.) | 46 (2) | 34 (11) |
> Median, No. | 43 | 92 |
Patient phenotypes | ||
Infected by flavivirus, ZIKV or DENV | 39 (43.33) | … |
Infected by togavirus, CHIKV | 23 (25.56) | … |
Infected by togavirus + flavivirus | 28 (31.11) | … |
Syndromes caused by arbovirusb | ||
ESD | 29 (32.22) | … |
PSD | 46 (51.11) | … |
ONDc | 15 (16.67) |
Demographic and Phenotypic Features . | Patients, No. (%)a . | Controls, No. (%) . |
---|---|---|
Total participants | 90 (31.03) | 200 (68.97) |
Sex | ||
Female | 47 (16.21) | 88 (30.34) |
Male | 43 (14.83) | 112 (38.62) |
Age | ||
< Median, No. | 43 | 97 |
Median, y (No.) | 46 (2) | 34 (11) |
> Median, No. | 43 | 92 |
Patient phenotypes | ||
Infected by flavivirus, ZIKV or DENV | 39 (43.33) | … |
Infected by togavirus, CHIKV | 23 (25.56) | … |
Infected by togavirus + flavivirus | 28 (31.11) | … |
Syndromes caused by arbovirusb | ||
ESD | 29 (32.22) | … |
PSD | 46 (51.11) | … |
ONDc | 15 (16.67) |
Abbreviations: CHIKV, chikungunya virus; DENV, dengue virus; ESD, encephalitis spectrum disorders; OND, other neurological disorders; PSD, peripheral spectrum disorders; ZIKV, Zika virus.
aPatients infected by arboviruses ZIKV, DENV, and/or CHIKV.
bESD: cerebellitis, encephalitis, meningoencephalitis, rhombencephalitis, myelitis, and myeloradiculitis; and PSD: polyneuropathy, polyradiculoneuropathy, radiculoneuropathy, and Guillain-Barré syndrome.
cA few patients were also diagnosed with OND, including optic neuritis, peripheral facial paralysis, and brain stroke.
The project protocol was approved by the ethics committee of the Hospital da Restauração (protocol No. CAAE63883517.4.3001.5198), and all the participants signed the informed consent forms.
DNA Extraction and HLA-II-DRB1/DQA1/DQB1 Typing
Genomic DNA was obtained from peripheral blood mononuclear cells using the DNAzol reagent (Thermo Fisher Scientific, Invitrogen), following the supplier’s instructions. The HLA-DRB1/DQA1/DQB1 loci were typed using sequence-specific oligonucleotides (LABType SSO kit, One Lambda) and named in accordance with the National Marrow Donor Program code (https://hml.nmdp.org/MacUI/).
Statistical Analysis
Genetic Association Studies
Allele, genotype, haplotype frequencies, and Hardy-Weinberg equilibrium (HWE) were performed using ARLEQUIN version 3.5.2 software [18]. HWE was tested by the exact test of Guo and Thompson [19]. Haplotypes were inferred with the same ARLEQUIN software using the expectation-maximization algorithm. The univariate analyses were performed by 2 × 2 contingency tables (binary variables), using R software (R version 3.4.2) [20]. The strength of the association was evaluated by the odds ratio (OR and 95% confidence interval [CI]), and P values were obtained using the 2-tailed Fisher exact test.
Association analyses were performed using only variants that exhibited a frequency > 1% (Supplementary Table 1A–1D). The following analyses were performed: (1) whole group of arbovirus infected patients versus controls, (2) ESD plus PSD patients versus control, (3) ESD syndrome versus controls, and (4) PSD syndrome versus controls. To limit the false positivity and enhance the accuracy of the results, we adopted a P value significance threshold of .005 [21] for univariate analysis followed by the Bonferroni correction (Pc). We included in multivariable analysis only univariate associations (Supplementary Tables 2 and 3) that reached the stringent P ≤ .005 and/or Pc ≤ .05 threshold. The multivariable analyses were done using the multiple logistic regression model, including the dichotomous dependent variables (infected/controls, ESD plus PSD/controls, ESD/controls, or PSD/controls) and the independent variables (HLA-DR/DQ variants), adjusted for age and sex (covariables; Supplementary Table 4). The model was determined by using the step procedure and the choice of the final model was made on the basis of the lowest Akaike information criterion [22]. Multivariate adjusted P values ≤ .05 were considered significant.
Binding Affinity of Virus Peptides to HLA-II Dimers
Because ZIKV is the prototype arbovirus associated with neurological complications, we used the complete ZIKV polyprotein, divided into 15-residue peptides (same size as the native peptides in the experimentally devised structures) with an overlap of 12 residues between adjacent peptides, for in silico studies. Variations among the 15 ZIKV virus strains were also considered, according to sequences deposited at National Center for Biotechnology Information (http://www.ncbi.nlm.nih.gov/). The binding free energy (ΔΔG) of the coupled peptide into the resolved native X-ray crystallographic structure (PDB ID: 5KSV; 5NI9) [23, 24] was compared to the values obtained for the virus peptides when coupled (replacing the native γ-chain) into the α- and β-beta chains of HLA-II dimers. Once the structure of each 15-mer peptide was computed, the binding free energy was compared to the value of the native peptide. The ΔΔG was calculated using Rosetta version 3.10.2 software [25, 26]. Geometry optimizations were performed using the Broyden-Fletcher-Goldfarb-Shanno algorithm, complying with the Armijo-Goldstein condition and the binding free energy was computed using the atomistic energy function REF2015 [27]. Sampling of the peptide backbone was enhanced by the backrub protocol. REF2015 has been parameterized against high-resolution structural data and its energy unit was expressed in kcal/mol [27, 28]. Electrostatic potential surfaces were calculated using the adaptive Poisson-Boltzmann solver [29] and plotted onto the HLA van der Walls surface, as rendered by visual molecular dynamics [30]. To estimate the cross-reactivity among virus peptides and HLA dimers, peptide homology between DENV1-4, CHIKV, and ZIKV was evaluated applying BLASTp [31], and using polyproteins retrieved from UNIPROT [32].
RESULTS
HLA-DRB1/DQA1/DQB1 Alleles, Genotypes, and Haplotypes
Besides comparing the frequencies of the DRB1/DQA1/DQB1 alleles, genotypes, and haplotypes between patients and controls, we also evaluated some of the putative dimers formed by HLA class II haplotypes that exhibited differential frequencies between patients and controls. To perform dimer analyses, we considered (1) only DRB1 allele groups for the DRA1/DRB1 haplotypes because the DRA1 locus has a negligible polymorphism, and (2) both cis and trans combinations for DQA1/DQB1 haplotypes. The terms DRA1/DRB1 and DQA1/DQB1 haplotypes refer to genetic studies and the putative molecules encoded by these haplotypes are DRA1/DRB1 and DQA1/DQB1 dimers. For genetic analyses, only cis haplotypes (cis dimers) were computed. The HLA-DRB1/DQA1/DQB1 loci fit the HWE for patients and controls, exhibiting P values > .05 (Supplementary Table 1A).
Several comparisons were performed exhibiting significant P values; however, in the results section, only comparisons that reached significance of P ≤ .005 for univariate analysis and P ≤ .05 for multivariable analysis adjusted for sex and age are shown (Table 2 and Table 3).
Multivariate Analyses for Comparisons of HLA-DRB1, DQA1, and DQB1 Alleles, Genotypes, Dimers, and Extended Haplotypes, Exhibiting P Value ≤ .005 in Univariate Analysis Between the Whole Group of Patients With Arbovirus (ZIKV, DENV, and CHIKV) Infections Versus Controls
. | Univariate (Unadjusted) . | Multivariate (Adjusted) . | ||||||||
---|---|---|---|---|---|---|---|---|---|---|
Variables . | Patient, % . | Control, % . | OR . | 95% CI . | P . | OR . | 95% CI . | Z Value . | Model Dispersion Coefficienta . | P . |
DRB1*04 allele/dimer | 15.70 | 36.50 | 0.33 | .16–.63 | .0003 | 0.49 | .23–.96 | −2.010 | 1.06 | .044 |
DRB1*15 allele/dimer | 36.00 | 18.50 | 2.47 | 1.35–4.49 | .002 | 1.66 | .88–3.11 | 1.570 | 1.06 | .116 |
DRB1*04/DRB1*13 | 0.00 | 9.00 | 0.00 | .00–.46 | .001 | 0.00 | 0–1 × 10+20 | −0.018 | 1.06 | .986 |
DRB1*03/DQA1*01/DQB1*02 | 11.10 | 1.00 | 12.26 | 2.53–117.64 | .0002 | 8.51 | 1.91–60.75 | 2.540 | 1.06 | .011 |
. | Univariate (Unadjusted) . | Multivariate (Adjusted) . | ||||||||
---|---|---|---|---|---|---|---|---|---|---|
Variables . | Patient, % . | Control, % . | OR . | 95% CI . | P . | OR . | 95% CI . | Z Value . | Model Dispersion Coefficienta . | P . |
DRB1*04 allele/dimer | 15.70 | 36.50 | 0.33 | .16–.63 | .0003 | 0.49 | .23–.96 | −2.010 | 1.06 | .044 |
DRB1*15 allele/dimer | 36.00 | 18.50 | 2.47 | 1.35–4.49 | .002 | 1.66 | .88–3.11 | 1.570 | 1.06 | .116 |
DRB1*04/DRB1*13 | 0.00 | 9.00 | 0.00 | .00–.46 | .001 | 0.00 | 0–1 × 10+20 | −0.018 | 1.06 | .986 |
DRB1*03/DQA1*01/DQB1*02 | 11.10 | 1.00 | 12.26 | 2.53–117.64 | .0002 | 8.51 | 1.91–60.75 | 2.540 | 1.06 | .011 |
Significant multivariate P values adjusted by sex and age are shown in bold.
Abbreviations: CHIKV, chikungunya virus; CI, confidence interval; DENV, dengue virus; OR, odds ratio; ZIKV, Zika virus.
aModel dispersion is the summary(model.final)$deviance/summary(model.final)$df.residual ratio. The model is dispersed when this ratio exceeds 1.5.
Multivariate Analyses for Comparisons of HLA-DRB1, DQA1, and DQB1 Alleles, Genotypes, Dimers, and Extended Haplotypes, Exhibiting P Value ≤ .005 in Univariate Analysis Between the Whole Group of Patients With Arbovirus (ZIKV, DENV, and CHIKV) Infections Versus Controls
. | Univariate (Unadjusted) . | Multivariate (Adjusted) . | ||||||||
---|---|---|---|---|---|---|---|---|---|---|
Variables . | Patient, % . | Control, % . | OR . | 95% CI . | P . | OR . | 95% CI . | Z Value . | Model Dispersion Coefficienta . | P . |
DRB1*04 allele/dimer | 15.70 | 36.50 | 0.33 | .16–.63 | .0003 | 0.49 | .23–.96 | −2.010 | 1.06 | .044 |
DRB1*15 allele/dimer | 36.00 | 18.50 | 2.47 | 1.35–4.49 | .002 | 1.66 | .88–3.11 | 1.570 | 1.06 | .116 |
DRB1*04/DRB1*13 | 0.00 | 9.00 | 0.00 | .00–.46 | .001 | 0.00 | 0–1 × 10+20 | −0.018 | 1.06 | .986 |
DRB1*03/DQA1*01/DQB1*02 | 11.10 | 1.00 | 12.26 | 2.53–117.64 | .0002 | 8.51 | 1.91–60.75 | 2.540 | 1.06 | .011 |
. | Univariate (Unadjusted) . | Multivariate (Adjusted) . | ||||||||
---|---|---|---|---|---|---|---|---|---|---|
Variables . | Patient, % . | Control, % . | OR . | 95% CI . | P . | OR . | 95% CI . | Z Value . | Model Dispersion Coefficienta . | P . |
DRB1*04 allele/dimer | 15.70 | 36.50 | 0.33 | .16–.63 | .0003 | 0.49 | .23–.96 | −2.010 | 1.06 | .044 |
DRB1*15 allele/dimer | 36.00 | 18.50 | 2.47 | 1.35–4.49 | .002 | 1.66 | .88–3.11 | 1.570 | 1.06 | .116 |
DRB1*04/DRB1*13 | 0.00 | 9.00 | 0.00 | .00–.46 | .001 | 0.00 | 0–1 × 10+20 | −0.018 | 1.06 | .986 |
DRB1*03/DQA1*01/DQB1*02 | 11.10 | 1.00 | 12.26 | 2.53–117.64 | .0002 | 8.51 | 1.91–60.75 | 2.540 | 1.06 | .011 |
Significant multivariate P values adjusted by sex and age are shown in bold.
Abbreviations: CHIKV, chikungunya virus; CI, confidence interval; DENV, dengue virus; OR, odds ratio; ZIKV, Zika virus.
aModel dispersion is the summary(model.final)$deviance/summary(model.final)$df.residual ratio. The model is dispersed when this ratio exceeds 1.5.
Multivariate Analyses for Comparisons of HLA-DRB1, DQA1, and DQB1 Alleles, Genotypes, Dimers, and Extended Haplotypes, Exhibiting P Value ≤ .005 in Univariate Analysis Between Patients Presenting With ESD Plus PSD, Only ESD, and Only PSD Versus Controls
. | Univariate (Unadjusted) . | Multivariate (Adjusted) . | ||||||||
---|---|---|---|---|---|---|---|---|---|---|
Variables . | ESD + PSD, ESD, or PSD, % . | Control, % . | OR . | 95% CI . | P . | OR . | 95% CI . | Z Value . | Model Dispersion Coefficienta . | P . |
Patients presenting with ESD plus PSD | ||||||||||
DQA1*03 | 20.00 | 41.50 | 0.35 | .17–.68 | .001 | 0.48 | .24–.92 | −2.129 | 1.03 | .033 |
DRB1*04/DRB1*13 | 0.00 | 9.00 | 0.00 | .00–.57 | .005 | 0.00 | 0–3 × 10+19 | −0.017 | 1.03 | .986 |
DRB1*03/DQA1*01/DQB1*02 | 12.00 | 1.00 | 13.35 | 2.67–130.25 | .0002 | 10.30 | 2.38–72.23 | 2.809 | 1.03 | .005 |
Patients presenting with only ESD | ||||||||||
DQA1*03 | 13.80 | 41.50 | 0.23 | .06–.69 | .004 | 0.30 | .08–.89 | −2.016 | 0.69 | .044 |
DQA1*01/DQB1*06 | 41.40 | 17.00 | 3.42 | 1.36–8.43 | .005 | 2.17 | .88–5.30 | 1.709 | 0.69 | .087 |
Patients presenting with only PSD | ||||||||||
DRB1*03 allele/dimer | 28.30 | 8.00 | 4.49 | 1.81–11.05 | .0005 | 3.34 | 1.27–8.69 | 2.473 | 0.81 | .013 |
DRB1*13 allele/dimer | 10.90 | 33.00 | 0.25 | .07–.67 | .002 | 0.25 | .08–.67 | −2.566 | 0.81 | .010 |
DQA1*01/DQA1*05 | 34.80 | 12.50 | 3.71 | 1.65–8.24 | .0007 | 3.56 | 1.48–8.53 | 2.863 | 0.81 | .004 |
. | Univariate (Unadjusted) . | Multivariate (Adjusted) . | ||||||||
---|---|---|---|---|---|---|---|---|---|---|
Variables . | ESD + PSD, ESD, or PSD, % . | Control, % . | OR . | 95% CI . | P . | OR . | 95% CI . | Z Value . | Model Dispersion Coefficienta . | P . |
Patients presenting with ESD plus PSD | ||||||||||
DQA1*03 | 20.00 | 41.50 | 0.35 | .17–.68 | .001 | 0.48 | .24–.92 | −2.129 | 1.03 | .033 |
DRB1*04/DRB1*13 | 0.00 | 9.00 | 0.00 | .00–.57 | .005 | 0.00 | 0–3 × 10+19 | −0.017 | 1.03 | .986 |
DRB1*03/DQA1*01/DQB1*02 | 12.00 | 1.00 | 13.35 | 2.67–130.25 | .0002 | 10.30 | 2.38–72.23 | 2.809 | 1.03 | .005 |
Patients presenting with only ESD | ||||||||||
DQA1*03 | 13.80 | 41.50 | 0.23 | .06–.69 | .004 | 0.30 | .08–.89 | −2.016 | 0.69 | .044 |
DQA1*01/DQB1*06 | 41.40 | 17.00 | 3.42 | 1.36–8.43 | .005 | 2.17 | .88–5.30 | 1.709 | 0.69 | .087 |
Patients presenting with only PSD | ||||||||||
DRB1*03 allele/dimer | 28.30 | 8.00 | 4.49 | 1.81–11.05 | .0005 | 3.34 | 1.27–8.69 | 2.473 | 0.81 | .013 |
DRB1*13 allele/dimer | 10.90 | 33.00 | 0.25 | .07–.67 | .002 | 0.25 | .08–.67 | −2.566 | 0.81 | .010 |
DQA1*01/DQA1*05 | 34.80 | 12.50 | 3.71 | 1.65–8.24 | .0007 | 3.56 | 1.48–8.53 | 2.863 | 0.81 | .004 |
Significant multivariate P values adjusted by sex and age are shown in bold.
Abbreviations: CI, confidence interval; ESD, neurological encephalitis spectrum; OR, odds ratio; PSD, peripheral spectrum disorders.
aModel dispersion is summary(model.final)$deviance / summary(model.final)$df.residual ratio. The model is dispersed when this ratio exceeds 1.5.
Multivariate Analyses for Comparisons of HLA-DRB1, DQA1, and DQB1 Alleles, Genotypes, Dimers, and Extended Haplotypes, Exhibiting P Value ≤ .005 in Univariate Analysis Between Patients Presenting With ESD Plus PSD, Only ESD, and Only PSD Versus Controls
. | Univariate (Unadjusted) . | Multivariate (Adjusted) . | ||||||||
---|---|---|---|---|---|---|---|---|---|---|
Variables . | ESD + PSD, ESD, or PSD, % . | Control, % . | OR . | 95% CI . | P . | OR . | 95% CI . | Z Value . | Model Dispersion Coefficienta . | P . |
Patients presenting with ESD plus PSD | ||||||||||
DQA1*03 | 20.00 | 41.50 | 0.35 | .17–.68 | .001 | 0.48 | .24–.92 | −2.129 | 1.03 | .033 |
DRB1*04/DRB1*13 | 0.00 | 9.00 | 0.00 | .00–.57 | .005 | 0.00 | 0–3 × 10+19 | −0.017 | 1.03 | .986 |
DRB1*03/DQA1*01/DQB1*02 | 12.00 | 1.00 | 13.35 | 2.67–130.25 | .0002 | 10.30 | 2.38–72.23 | 2.809 | 1.03 | .005 |
Patients presenting with only ESD | ||||||||||
DQA1*03 | 13.80 | 41.50 | 0.23 | .06–.69 | .004 | 0.30 | .08–.89 | −2.016 | 0.69 | .044 |
DQA1*01/DQB1*06 | 41.40 | 17.00 | 3.42 | 1.36–8.43 | .005 | 2.17 | .88–5.30 | 1.709 | 0.69 | .087 |
Patients presenting with only PSD | ||||||||||
DRB1*03 allele/dimer | 28.30 | 8.00 | 4.49 | 1.81–11.05 | .0005 | 3.34 | 1.27–8.69 | 2.473 | 0.81 | .013 |
DRB1*13 allele/dimer | 10.90 | 33.00 | 0.25 | .07–.67 | .002 | 0.25 | .08–.67 | −2.566 | 0.81 | .010 |
DQA1*01/DQA1*05 | 34.80 | 12.50 | 3.71 | 1.65–8.24 | .0007 | 3.56 | 1.48–8.53 | 2.863 | 0.81 | .004 |
. | Univariate (Unadjusted) . | Multivariate (Adjusted) . | ||||||||
---|---|---|---|---|---|---|---|---|---|---|
Variables . | ESD + PSD, ESD, or PSD, % . | Control, % . | OR . | 95% CI . | P . | OR . | 95% CI . | Z Value . | Model Dispersion Coefficienta . | P . |
Patients presenting with ESD plus PSD | ||||||||||
DQA1*03 | 20.00 | 41.50 | 0.35 | .17–.68 | .001 | 0.48 | .24–.92 | −2.129 | 1.03 | .033 |
DRB1*04/DRB1*13 | 0.00 | 9.00 | 0.00 | .00–.57 | .005 | 0.00 | 0–3 × 10+19 | −0.017 | 1.03 | .986 |
DRB1*03/DQA1*01/DQB1*02 | 12.00 | 1.00 | 13.35 | 2.67–130.25 | .0002 | 10.30 | 2.38–72.23 | 2.809 | 1.03 | .005 |
Patients presenting with only ESD | ||||||||||
DQA1*03 | 13.80 | 41.50 | 0.23 | .06–.69 | .004 | 0.30 | .08–.89 | −2.016 | 0.69 | .044 |
DQA1*01/DQB1*06 | 41.40 | 17.00 | 3.42 | 1.36–8.43 | .005 | 2.17 | .88–5.30 | 1.709 | 0.69 | .087 |
Patients presenting with only PSD | ||||||||||
DRB1*03 allele/dimer | 28.30 | 8.00 | 4.49 | 1.81–11.05 | .0005 | 3.34 | 1.27–8.69 | 2.473 | 0.81 | .013 |
DRB1*13 allele/dimer | 10.90 | 33.00 | 0.25 | .07–.67 | .002 | 0.25 | .08–.67 | −2.566 | 0.81 | .010 |
DQA1*01/DQA1*05 | 34.80 | 12.50 | 3.71 | 1.65–8.24 | .0007 | 3.56 | 1.48–8.53 | 2.863 | 0.81 | .004 |
Significant multivariate P values adjusted by sex and age are shown in bold.
Abbreviations: CI, confidence interval; ESD, neurological encephalitis spectrum; OR, odds ratio; PSD, peripheral spectrum disorders.
aModel dispersion is summary(model.final)$deviance / summary(model.final)$df.residual ratio. The model is dispersed when this ratio exceeds 1.5.
HLA-DRB1/DQA1/DQB1 Variants Are Associated With Susceptibility/Protection to Arbovirus Neurological Complications
Compared to controls, the DRB1*04 allele group or the DRB1*04 dimer was overrepresented in controls compared to whole group of patients (univariate analysis P = .0003; multivariable P = .044; Table 2), while the DRB1*03/DQA1*01/DQB1*02 extended haplotype was overrepresented in the whole group of patients (univariate analysis P = .0002; multivariable P = .011; Table 2).
Considering the stratified ESD plus PSD or ESD or PSD patient groups in comparison to controls: (1) the DQA1*03 allele group exhibited higher frequency in controls than in both the ESD plus PSD patient group (univariate analysis P = .001; multivariable P = .033; Table 3) and the ESD patient group (univariate analysis P = .004; multivariable P = .044; Table 3); (2) the DRB1*03/DQA1*01/DQB1*02 extended haplotype was overrepresented in the ESD plus PSD patient group (univariate analysis P = .0002; multivariable P = .005; Table 3); and (3) the DRB1*03 allele group or dimer (univariate analysis P = .0005; multivariable P = .013; Table 3) and the DQA1*01/DQA1*05 genotype (univariate analysis P = .0007; multivariable P = .004; Table 3) were found in higher frequency in PSD patients. In addition, the DRB1*13 allele group or dimer was overrepresented in controls compared to PSD patients (univariate analysis P = .002; multivariable P = .010; Table 3).
Binding Affinity of Arbovirus-Derived Peptides to DRA1/DRB1 and DQA1/DQB1 Dimers
Considering that (1) some protein structures of the DRA1/DRB1 and DQA1/DQB1 dimers coupled with 15-residue peptides have been resolved by X-ray crystallography [23, 24], (2) some dimers are available for in silico studies [23, 33], and (3) ZIKV is the major agent associated with neurological manifestations, we used a library of ZIKV polyproteome peptides to evaluate their interaction with dimers. We selected available dimer constructions associated with protection (DRA1/DRB1*04) or susceptibility (DQA1*05/DQB1*02) to arbovirus-related neurological complications. Both dimers were evaluated for their binding affinities for 1878 15-mer peptides covering the entire of ZIKV polyprotein of the 15 strains. Binding free energy (ΔΔG) values for the native structures of the DQA1*05/DQB1*02 and DRA1/DRB1*04:01 complexes were −69 and −54 kcal/mol, respectively. Accordingly, peptides that presented binding free energy values lower than the values for the native peptides would have higher affinity and, therefore, were able to bind to HLA dimers. The DQA1*05/DQB1*02 dimer can bind with high affinity to a remarkable number of hypothetical ZIKV peptides. A total of 235 15-mer ZIKV polyprotein-derived peptides could be presented by the DQA1*05/DQB1*02 dimer (Supplementary Table 5), whereas only 40 peptides were bound to DRA1/DRB1*04:01 dimer with higher affinity than the native counterpart (Supplementary Table 6). The distribution of the high-affinity peptides across the ZIKV proteins that can bind to the dimers is shown in Figure 1A. To assess statistical relevance regarding the ZIKV proteins presented by HLA dimers, protein length should be considered. The relative coverage (percentage of ZIKV 15-mer peptides that can bind to a dimer with higher affinity than the dimer’s native peptide) of the high-affinity peptides on each ZIKV protein for both HLA molecules is presented in Figure 1B. Notably, among peptides exhibiting highest affinities to bind to the DQA1*05/DQB1*02 dimer, the ZIKV proteins prM and NS2A were highly represented, whereas no peptide from NS2B protein was able to bind to the DRA1/DRB1*04:01 dimer (Figure 1A and 1B). There was little overlap between the peptides that bind to each HLA dimer (Supplementary Tables 5 and 6). The discrepancy in the ZIKV peptide repertoire that can bind to each HLA can be understood by inspecting the differences in charge surface of each binding site. Plotting of the electrostatic potential onto the protein van der Walls surface revealed that DQA1*05/DQB1*02 dimer has a highly positively charged groove (Figure 1C), while HLA/DRB1*04:01 dimer displays a more amphipathic and yet less hydrophilic site (Figure 1D). In addition, 24, 27, 5, and 38 ZIKV peptides predicted to bind to the DQA1*05/DQB1*02 dimer were homologous to DENV1–4 peptides, respectively (Supplementary Tables 7). Interestingly, only a few ZIKV peptides, which were similar to each other and homologous to DENV1 (6 peptides), DENV2 (6 peptides), and DENV4 (8 peptides), were predicted to bind to DRA1/DRB1*04 dimer (Supplementary Tables 8).
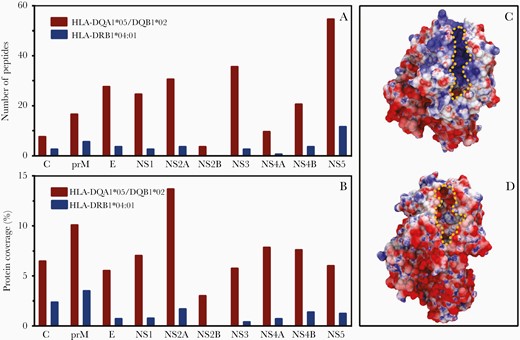
Distribution of 15-mer peptides derived from the Zika virus polyprotein capable of binding with high affinity to HLA-DQA1*05/DQB1*02 and HLA-DRA1/DRB1*04:01 dimers and HLA electrostatic surface potential. A, Number of peptides from each protein. B, Protein coverage, defined as the ratio of the number of peptides over the length of each protein. C and D, Electrostatic potential (from −6 to +6 kJ/mol/e) plotted onto the van der Walls surface of the DQA1*05/DQB1*02 dimer (C) and DRA1/DRB1*04:01 dimer (D). Negatively charged areas are represented in red, positively charged areas are represented in blue, and neutral areas are represented in white; peptide binding clefts are highlighted by a dotted yellow line.
DISCUSSION
HLA-I molecules have a pivotal role in viral infection control [34]; however, the class II presentation of viral peptides to T-cell CD4+ is the first step of the adaptive immunity that may impact on viral disease outcome [35], and HLA-II gene variants are expected to modulate host immune response against viruses, depending on residue features of the binding groove. Mutations in HLA-II genes have been associated with susceptibility/protection for many arbovirus infections, including DENV, West Nile virus, and other flaviviruses [11, 12, 14, 15, 36]. We evaluated the association between HLA-II alleles, genotypes, haplotypes, and their encoded dimers on the susceptibility to arbovirus-related neurological complications.
Although DRB1 alleles (DRB1*15 and DRB1*03 considered as alleles and haplotypes) were associated with susceptibility to arbovirus infections (Supplementary Tables 2 and 3), the most striking associations were observed with DQA1*05 (genotype and haplotype), DQA1*01 (genotype and haplotype), DQB1*02 (genotype and haplotype), and DQB1*06 (genotype and haplotype), which were overrepresented in arbovirus-infected patients, considered as the whole group and as major neurological syndromes (ESD and PSD). Accordingly, the haplotypes that encoded the DQA1*01/DQB1*02 and DQA1*01/DQB1*06 dimers showed strong associations with susceptibility to arbovirus infections and ESD or PSD. Notably, a strong linkage disequilibrium (LD) has been reported for DRB1 and DQB1 alleles, and in the Brazilian population the DRB1*15 allele group is primarily in LD with the DQB1*06 group, whereas the DRB1*03 group is primarily associated with the DQB1*02, 03, and 04 groups [37, 38]. In addition to DRB1*15, the DRB1*11 group, which exhibited a frequency closely similar to controls, also exhibit strong LD with DQB1*06 alleles. Therefore, LD between DRB1/DQB1 alleles may also contribute to the increased frequency of DQB1*06 alleles, and the participation of DRB1 alleles (particularly DRB1*15 and DRB1*03) may be due to a hitchhiking LD with DQB1 alleles. Besides DRB1/DQB1 LD, some DRB1/DQA1/DQB1 extended haplotypes were also associated with susceptibility to arbovirus neurological infections. The increased frequency of the haplotypes DRB1*03/DQA1*01/DQB1*02 and DRB1*15/DQA1*05/DQB1*06 (Supplementary Tables 2 and 3) may yield several cis dimers (DQA1*01/DQB1*02 and DQA1*05/DQB1*06). In parallel, several trans dimers may also be formed, including several combinations of DQA1*01 and DQA1*05 at one haplotype with DQB1*02 and DQB*06 at the other haplotype. To further understand the role of DQA1/DQB1 dimers we performed an in silico study to evaluate the peptide profile that can be presented by such dimers, and we illustrated these studies by coupling ZIKV peptides to dimers. Surprisingly, a single dimer (DQA1*05/DQB1*02) presented hundreds of virus peptides. Considering that a great number of cis and trans DQA1/DQB1 dimers can be generated by the susceptibility haplotypes, the number of virus peptides to be presented to T cells may drastically increase. If, on the one hand, the increased number of peptides may result in a diverse immune response against virus peptides, but on the other hand, the association of several haplotypes with susceptibility to arbovirus may indicate that peptides may be redundant and/or cross-reacting, impairing the effective immune response against the virus.
In contrast to susceptibility alleles, the most striking association with protection was conferred by the DRB1*04 allele group, which was underrepresented in the whole group of patients. The in silico studies showed that only dozens of Zika virus peptides were presented by the DRA1/DRB1*04 dimer, contrasting to hundreds of peptides that can be presented by DQA1/DQB1 dimers. The reduced number of virus peptides may indicate that a more robust and more specific immune response may be mounted by DRB1 molecules.
The chemical nature of the clefts formed by DQA1*05/DQB1*02 or DRB1/DRB1*04 are quite distinct. While the former is mainly hydrophilic and positively charged (Figure 1C), the latter is less hydrophilic and exhibits an amphipathic character (Figure 1D). In addition, little overlap is seen for the repertoire of ZIKV peptides that can bind to each dimer. As shown in Figure 1, the DQA1*05/DQB1*02 dimer is highly promiscuous to ZIKV hypothetical peptides. Among those, it is reported that prM and NS2A proteins share a role in the modulation of virion assembly [39], and prM has been associated with neurovirulence [39]. In contrast, HLA-DRB1*04 is more specific (binds to fewer peptides) and prM was the most represented protein (Figure 1B). Notably, immune response against prM is likely to be essential for protection because DNA-based Zika vaccines that did not code for prM did not achieve appropriate level of protection [40].
Peptide promiscuity among HLA class I and II molecules is a widely discussed subject; however, molecules that present larger number of peptides promote immune response against a broad range of pathogens [41, 42], but may not be able to respond to new virulent pathogens [41]. In contrast, particular dimers may provide protection against highly virulent pathogens, such as human immunodeficiency virus [43], hepatitis C virus [44, 45], and Leishmania species [42]. These observations are consistent with our findings, in which some DQA1/DQB1 dimers (for instance, DQA1*05/DQB1*02) act as generalist molecules, while others (DRA1/DRB1*04) act as specialist molecules towards ZIKV peptides. It has also been proposed that pathogen diversity drives the evolution of promiscuous HLA-II alleles in regions of high pathogen diversity [46]. Indeed, a higher prevalence of the 4 DENV serotypes in the northeastern region of Brazil [47] may account for the higher number of ESD and PSD cases compared to other regions in the country. Interestingly, our results show that ZIKV NS2A protein is highly covered by the DQA1*05/DQB1*02 dimer, and the cross-reactivity between ZIKV and DENV T-cell responses [48, 49] may account for the difficulty in discriminating between these infections.
One of the limitations of this exploratory report is the size of our cohort (90 patients). Few studies have addressed the association of HLA-DR-DQ genes with arbovirus infections, particularly focusing on dengue [11, 12, 50] and chikungunya [10, 13], encompassing sample sizes ranging from 39 to 110 patients; however, no studies have been conducted to evaluate the effect of these genes in neurological complications. In the present study, all 90 cases analyzed exhibited neurological complication of arbovirus disorders, diagnosed in a defined time in a restricted geographical area of Brazil, possibly presenting with the same virus strains. Despite the sample size, in this series we detected several HLA-II associations that (1) reached the stringent P values (P ≤ .005 and/or Bonferroni corrected Pc ≤ .05) threshold fixed for univariate analyses, (2) reached the stringent the P value .05 threshold for the multivariable analysis following correction for sex and age, and (3) exhibited a biological relevance in in silico studies, explaining at least in part the increased frequency of neurological complications following the arbovirus outbreak that occurred in the state of Pernambuco, Brazil. Another limitation was the controls. The ideal control group would be healthy individuals who presented positive serology or previous history of dengue, chikungunya, and Zika infections; however, the patients exhibited these infections in several combinations, impairing the availability of controls with the same viral serologic profile but without neurological manifestations. To circumvent these limitations, we chose healthy bone marrow donors because (1) they are from the same geographical and endemic region as the patients, (2) they represent the allele frequency of the studied population, and (3) the frequency deviation from that of the patients gives a clue to the differential alleles implicated in disease pathogenesis, satisfying the objectives of the study.
In conclusion, the ensemble of genetic and in silico studies indicate that DQA1 and DQB1 alleles primarily considered as haplotypes are associated with susceptibility to arbovirus infection, possibly due to (1) the large number of DQA1/DQB1 molecules that can be generated by the haplotypes exhibiting differential frequencies between patients and controls, (2) broad peptide specificity, as shown by the large number of arbovirus peptides that these dimers can present, and (3) the distinct chemical nature of the DQA1/DQB1 dimer cleft when compared to that formed by DRA1/DRB1 molecules. In contrast, the protection conferred by the specific DRA1/DRB1 dimer may associated with the presentation of a limited number of arbovirus peptides, suggesting that protective molecules may recognize arbovirus peptides more specifically and more efficiently.
Supplementary Data
Supplementary materials are available at The Journal of Infectious Diseases online. Consisting of data provided by the authors to benefit the reader, the posted materials are not copyedited and are the sole responsibility of the authors, so questions or comments should be addressed to the corresponding author.
Notes
Acknowledgments. The authors thank patients and relatives for their free consent, and the health professionals of the neurology service in the Hospital da Restauração for their great collaboration.
Author contributions. E. A. D. and N. L.-S. conceived, designed, and applied for grants to perform the study. M. L. B. F., C. A. A. B., M. F. M. A., M. T. C., and R. S. A. collected samples and provided clinical and laboratory information. N. H. S. D., G. H. W., and E. L. G. performed HLA-II gene typing. P. S. processed sequenced data and wrote the original manuscript draft. P. S. and R. S. A. performed statistical analyses. A. F. P. J. and R. D. L. performed the computer simulations. P. S., R. S. A., M. T. C., C. A. A. B., M. F. M., R. D. L., E. A. D., and N. L.-S. performed data curation. All authors have critically read and edited the manuscript.
Financial support. This work was supported by Conselho Nacional de Desenvolvimento Científico e Tecnológico (CNPq; grant numbers 440760/2016-0 and 302060/2019-7); Coordenação de Aperfeiçoamento de Pessoal de Nível Superior (grant number 88881.130769/2016-01); and the Aggeu Magalhães Institute/Fundação Oswaldo Cruz. P. S. was supported by Fundação Oswaldo Cruz (grant number INOVA FIOCRUZ/02/2019 PDJ); N. L.-S. and R. D. L. are CNPq scholarship holders (grant numbers 310364/2015-9 and 310892/2019-8); and A. F. P. J. was sponsored by Fundação de Amparo a Ciência e Tecnologia do Estado de Pernambuco. Computer allocation was provided by Laboratório Nacional de Computação Científica.
Potential conflicts of interest. All authors: No reported conflict of interest. All authors have submitted the ICMJE Form for Disclosure of Potential Conflicts of Interest. Conflicts that the editors consider relevant to the content of the manuscript have been disclosed.