-
PDF
- Split View
-
Views
-
Cite
Cite
Liyun Liu, Liqiong Song, Rong Deng, Ruiting Lan, Wenjie Jin, Guy Tran Van Nhieu, Huifang Cao, Qin Liu, Yuchun Xiao, Xianping Li, Guangxun Meng, Zhihong Ren, Citrobacter freundii Activation of NLRP3 Inflammasome via the Type VI Secretion System, The Journal of Infectious Diseases, Volume 223, Issue 12, 15 June 2021, Pages 2174–2185, https://doi.org/10.1093/infdis/jiaa692
- Share Icon Share
Abstract
Citrobacter freundii is a significant cause of human infections, responsible for food poisoning, diarrhea, and urinary tract infections. We previously identified a highly cytotoxic and adhesive C. freundii strain CF74 expressing a type VI secretion system (T6SS). In this study, we showed that in mice-derived macrophages, C. freundii CF74 activated the Nucleotide Oligomerization Domain -Like Receptor Family, Pyrin Domain Containing 3(NLRP3) inflammasomes in a T6SS-dependent manner. The C. freundii T6SS activated the inflammasomes mainly through caspase 1 and mediated pyroptosis of macrophages by releasing the cleaved gasdermin-N domain. The CF74 T6SS was required for flagellin-induced interleukin 1β release by macrophages. We further show that the T6SS tail component and effector, hemolysin co-regulation protein-2 (Hcp-2), was necessary and sufficient to trigger NLRP3 inflammasome activation. In vivo, the T6SS played a key role in mediating interleukin 1β secretion and the survival of mice during C. freundii infection in mice. These findings provide novel insights into the role of T6SS in the pathogenesis of C. freundii.
Type VI secretion systems (T6SSs) are highly sophisticated protein-injection nanomachines that deliver effectors into a variety of target cells [1]. On cell contact, the T6SSs deliver toxic effectors into the target cells during interbacterial competition or eukaryotic host cell infections [1]. They have also been shown to be involved in regulating inflammasome activation [2]. The Burkholderia cenocepacia T6SS effector T6SS effector protein affecting cytoskeletal architecture disrupts the host actin cytoskeleton by deamidating Rho guanosine triphosphatases and activates the pyrin inflammasome [3]. In Edwardsiella tarda, the T6SS effector E. tarda virulent protein P inhibits NLRP3 inflammasome activation by preventing cytoplasmic calcium ion increase and suppressing c-Jun N-terminal kinase (Jnk) activation to impair oligomerization of the inflammasome adaptor apoptosis-associated speck-like protein containing acaspase-recruitment domain (ASC) [2].
Inflammasomes are intracellular protein complexes assembled by cytosolic pattern recognition receptors from the nucleotide-binding oligomerization domain (NOD)-like receptor and the pyrin and HIN domain (PYHIN) protein family, on recognition of microbe-associated molecular patterns or danger-associated molecular patterns [4, 5]. Assembly of an inflammasome triggers proteolytic cleavage of dormant pro–caspase 1 into active caspase 1, which converts the cytokine precursors pro–interleukin 1β (pro-IL-1β) and pro–interleukin 18 (pro-IL-18) into mature and biologically active IL-1β and IL-18, respectively [6–8]. To date, 5 inflammasomes, Nuclear localization leucine-rich-repeat protein 1 (NLRP1), Nucleotide Oligomerization Domain–Like Receptor Family, Pyrin Domain Containing 3 (NLRP3), NLR Family, CARD domain containing 4 (NLRC4), pyrin, and the absent in melanoma 2 (AIM2), have been reported [8].
Activation of the NLRP3 inflammasome is mediated by 2 signals [8]. The first signal, referred as priming, is provided by microbial or endogenous molecules that induce NLRP3 and pro–IL-1β expression through the activation of Nuclear factor kappa B (NF-kB); the second signal activates NLRP3 and is induced by nigericin, adenosine triphosphate (ATP), bacterial pore-forming toxins, viral RNA, or particulate matter [8–12]. However, it remains unclear how these structurally unrelated stimuli activate NLRP3 [12]. Multiple signals have been reported to trigger NLRP3 inflammasome activation, including the production of reactive oxygen species (ROS), mitochondrial damage, lysosomal damage, formation of large nonspecific pores in the cell membrane, and cytosolic potassium ion (K+) efflux [13, 14].
Citrobacter freundii is a commensal of the intestinal tract of humans and animals [15]. However, some C. freundii strains can cause a range of infections in humans [16–19]. Our group’s previous studies showed that isolates from human diarrheal cases were cytotoxic to mammalian cells in vitro [15, 20] and that a cytotoxic and adhesive strain, CF74, carries a type VI secretion system (T6SS) [21]. In the current study, we investigated the roles and the mechanisms of the T6SS in the pathogenesis of C. freundii.
MATERIALS AND METHODS
Bacterial Strains and Plasmids
The wide-type (WT) cytotoxic C. freundii strain CF74 was isolated from a goat fecal sample. Noncytotoxic WT C. freundii strain CF72 was isolated from a pig fecal sample [21]. CF74 mutants were constructed by homologous recombination as described elsewhere [15]. Plasmid pBAD24- and pBAD33-based constructs were used for the complementation of the deletion mutants (Supplementary Table 1).
Ethics Statement
The animal procedures were approved by Laboratory Animal Welfare & Ethics Committee of the National Institute for Communicable Disease Control and Prevention, Chinese Center for Disease Control and Prevention, Beijing. The study was carried out in accordance with the recommendations of animal management regulations (2017/03) and Measures for the Ethical Review of Biomedical Research Involving Humans (2016/12; no. SYXK [Beijing] 2012-0022).
Mice and Cell Culture
Female C57BL/6J WT mice (7–8 weeks old) weighting 18–20 g were purchased from Beijing Vital River Laboratory Animal Technology. All mouse studies were conducted in accordance with the protocols approved by the Welfare & Ethical Inspection in Animal Experimentation Committee at the Chinese CDC. Casp11−/− and Casp1/11−/− mice were from the Feng Shao and Jackson laboratory, respectively [22]. Nlrp3−/− mice [23, 24] and Nlrc4−/− mice were kindly provided by Vishva Dixit from Genentech. Bone marrow–derived macrophages (BMMs) were generated and cultured as described elsewhere [23]. The macrophages prepared were ≥90% pure, as assessed by expression of F4/80 using flow cytometry [23].
In Vitro Bacterial Infection, Phagocytosis of Bacteria, and Caspase 1 Activation
BMMs were incubated with WT CF74, mutants, or complements at a multiplicity of infection of 10. For colony-forming unit (CFU) count, after 4 hours of infection, cells were treated with gentamicin, washed, lysed. The lysates were diluted and spread on the Luria-Bertani agar plate to quantify the number of intracellular bacteria CFUs. As needed, BMMs were preincubated for 2 hours with CA-074 methyl ester (CA-074 Me) (C5857; Calbiochem), acetyl-tyrosyl-valyl-alanyl-aspartyl chloromethylketone (AC-YVAD-CMK) (Alexis Biochemicals), oATP (Merck), N-acetyl-L-cysteine (A7250 Sigma-Aldrich), or potassium chloride (KCl) at the indicated concentrations. At the indicated time after stimulation, supernatants and cell lysates were collected for immunoblotting. Proteins were incubated with antibodies specific for anti-mouse caspase 1 (AG-20B-0042; Adipogen) 1:1000, anti-mouse IL-1β (AF-401-NA; R&D Systems) 1:1000, anti-rabbit caspase 11 17D9 (NB120-10454; Novus Biologicals) 1:1000, cleaved caspase 8 Asp387 (9429; CST) 1:1000, anti-rabbit gasdermin D (GSDMD) (EPR19828; Abcam) 1:1000, mouse anti–His tag monoclonal antibody (AE003; AB clonal) 1:2000, or anti-mouse β-actin (sc-47778) 1:1000, followed by incubation with horseradish peroxidase–conjugated goat anti-mouse immunoglobulin G (MBL;1:3000); proteins were then detected on enhanced chemiluminescence film (Kodak) using a Protec automatic film processor (Optimax).
Protein Expression, Purification, and Transfection Into Cells
The hcp-2 coding DNA was PCR amplified from C. freundii CF74 DNA using specific primers (Supplementary Table 1) and cloned into the NdeI/HindIII site of pET-30a. Negative control was a Shiga toxin B unit 2 (StxB2) subunit [25]. All recombinant proteins were expressed in E. coli BL21 (DE3), and purified by means of nickel affinity chromatography [26, 27]. To remove the majority of endotoxin contaminants, proteins bound to the nickel–nitrilotriacetic acid column were washed again with 60% isopropanol in the wash buffer (>30× column volume) [28]. BMMs were plated at a density of 1 × 106/mL in 6-well plates, primed with lipopolysaccharide at 100 ng/mL for 3 hours, and then transfected with 1 µg of purified proteins using Lipofectamine 3000 reagent (Invitrogen) or added to the cell culture medium. After 16 hours, the culture supernatants were collected and cytotoxicity and cytokine production were determined by means of lactate dehydrogenase (LDH), enzyme-linked immunosorbent assay (ELISA), or Western blotting, respectively.
LDH and Cytokine ELISAs
LDH release in the culture supernatants was measured using a Cytotox96 assay Kit (Promega). The concentrations of cytokines in cell-free supernatants were quantified using an ELISA kit (BD Biosciences).
Preparation and Analysis of Bacterial Whole-Cell Extracts and Culture Supernatant
Bacterial whole-cell lysates and culture supernatant proteins were collected as described elsewhere [15]. Immobilized proteins were incubated with primary antibodies, namely, rabbit anti-Flagellin (FliC) (Abzome; 1:5000) and mouse anti-Glyceraldehyde-3-phosphate dehydrogenase (GAPDH) (Thermo Fisher Scientific; 1:5000), followed by incubation with horseradish peroxidase–conjugated goat anti-rabbit (MBL; 1:10 000), and goat anti-mouse immunoglobulin G (MBL; 1:3000), respectively, and then detected on enhanced chemiluminescence film (Kodak) using a Protec automatic film processor (Optimax).
Mouse Infections
To determine the in vivo inflammasome response to C. freundii infection, 6-week-old female C57BL/6 mice were infected intraperitoneally with 2 × 107 CFUs per mouse. Mice were euthanized at day 2 after infection, and cytokine production in peritoneal lavage fluid (PLF) was measured. To determine mouse survival, 6-week-old female C57BL/6 mice were infected intraperitoneally with 1 × 108 CFUs per mouse, and survival was recorded every 15 hours from 15 to 90 hours after infection.
Statistical Analysis
Statistical analysis was performed using GraphPad Prism (version 5.00; GraphPad Software). Student t test and analysis of variance were used for comparison of mean values between 2 groups and mean values of >2 groups, respectively.
RESULTS
C. freundii CF74 Activation of Inflammasomes in Macrophages
We first tested the ability of 2 C. freundii strains that differ in their virulence properties to induce inflammasome activation. The highly cytotoxic (virulent) strain CF74 and the noncytotoxic (nonvirulent) C. freundii strain CF72 [21] were used to infect BMMs derived from C57BL/6 mice. CF74 induced significantly more caspase 1 activation, IL-1β secretion, and higher cytotoxicity in BMMs, compared with CF72 (Figure 1A–1D). In contrast, BMMs infected with CF74 and CF72 released similar levels of IL-6 and tumor necrosis factor (TNF) α (Supplementary Figure 1A and 1B). Thus, the virulent strain CF74 was a more potent activator of inflammasome than the nonvirulent strain CF72.
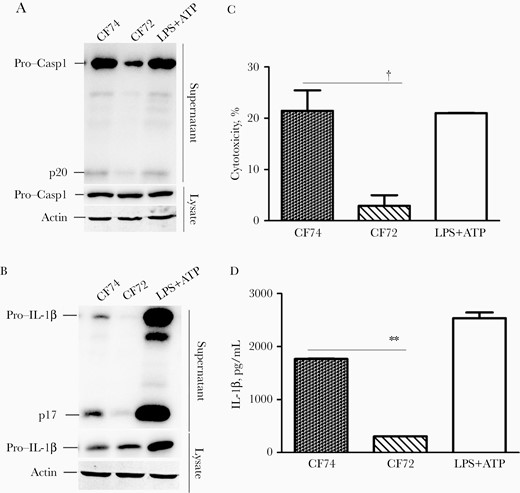
CF74 activates the inflammasomes. Macrophages from the indicated C57BL/6 mice were infected with Citrobacter freundii CF74 or CF72 for 4 hours at a multiplicity of infection of 10. A, B, Caspase 1 activation and interleukin 1β (IL-1β) secretion in the bone marrow–derived macrophages infected with C. freundii were analyzed by immunoblotting. Abbreviations: ATP, adenosine triphosphate; LPS, lipopolysaccharide; lysate, whole-cell lysates; p17, IL-1β secretion; p20, activated caspase 1; Pro-Casp1, pro–caspase 1. C, D, Quantification of lactate dehydrogenase release (C) and IL-1β secretion (D); error bars represent standard deviations from ≥3 determinations. †P < .01 (analysis of variance). LPS + ATP served as the positive control.
To determine which inflammasome was induced by CF74, macrophages from Casp1/11−/−, Nlrp3−/−, Nlrc4−/−, and WT C57BL/6 mice were subjected to bacterial infection. IL-1β secretion could not be detected in Casp1/11−/− BMMs, as shown by the normalized quantification of the IL-1β–cleaved product p17 (Figure 2A and 2B). Consistently, caspase 1 activation was abolished in Casp1/11−/− BMMs, confirming specific activation of inflammasome during CF74 infection (Supplementary Figure 2). Caspase 1 activation and IL-1β secretion were strongly reduced in BMMs derived from Nlrp3−/− mice, suggesting a major role for the NLRP3 inflammasome during CF74 infection (Figure 2A, 2B, and 2D and Supplementary Figure 2). In addition, CF74-induced cytotoxicity was distinctly also reduced in BMMs derived from Casp1/1−/− and Nlrp3−/− mice (Figure 2C). As expected, no difference in the levels of TNF-α were detected in macrophages derived from the different mouse backgrounds (Supplementary Figure 3D). Thus, CF74 mainly activates the NLRP3 inflammasome during infection of macrophages.
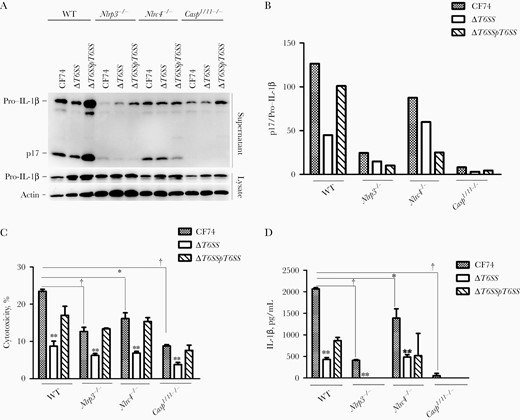
CF74 type VI secretion system (T6SS) contributed to interleukin 1β (IL-1β) secretion by macrophages. Bone marrow–derived macrophages (BMMs) from the indicated C57BL/6 mice were infected with the CF74, isogenic mutants, and complements for 4 hours at a multiplicity of infection of 10. A, IL-1β secretion in the BMMs infected with the CF74, isogenic ΔT6SS mutant, and complement ΔT6SS/pT6SS were analyzed by immunoblotting. Abbreviations: lysate, whole cell lysates; p17, IL-1β secretion; WT, wide type. B, Relative quantification of IL-1β secretion in supernatants; numbers represent densitometric quantifications of relative band intensities of p17 normalized to pro–IL-1β in the lysis. C, D, Quantification of lactate dehydrogenase release (C) and IL-1β secretion (D). Error bars represent standard deviations from ≥3 determinations. *P < .05; †P < .01 (analysis of variance).
Role of the T6SS in CF74-Induced IL-1β Secretion From Macrophages
In other bacterial species, T6SSs have been reported to activate the pyrin inflammasome or inhibit the NLRP3 inflammasome [2, 3]. Because CF74 expresses a functional T6SS essential for cytotoxicity [15], we analyzed its contribution in inflammasome activation. As shown in Figure 2A, 2B, and 2D and Supplementary Figure 3A and 3B, the isogenic ΔT6SS mutant derivative was impaired in inducing caspase 1 activation and secretion of IL-1β, a defect that was partially transcomplemented. As expected, the ΔT6SS mutant showed reduced cytotoxicity (Figure 2C), while showing no detectable difference in the levels of released TNF-α (Supplementary Figure 3D and 3E). The levels of released IL-1β were reduced in Nlrp3−/− macrophages challenged by CF74 (Figure 2A, 2B, and 2D). However, a further reduction was observed on infection with the ΔT6SS mutant in each cell lines (Figure 2A, 2B, and 2D). Thus, the T6SS was critical for the activation of the NLRP3 inflammasome during CF74 infection. In contrast, CF74 did not induce detectable levels of IL-1β and activation of inflammasomes in the human colon adenocarcinoma cell line HT29 tested (Supplementary Figure 3C).
T6SS Activation of Caspase 1 During CF74 Infection of Macrophages
To further determine the role of caspase 1 and caspase 11 in inflammasome activation induced by the CF74 T6SS, we challenged BMMs from Casp11−/− C57BL/6 mice and BMMs pretreated with caspase 1 inhibitor AC-YVAD-CMK, respectively. In response to CF74 infection, Casp11−/−-deficient-BMMs or BMMS pretreated with AC-YVAD-CMK induced significantly lower levels of caspase 1 activation, IL-1β secretion, and cytotoxicity than WT BMMs without pretreatment (Figures 3A–3C). Consistent with a role of the T6SS independent of caspase 11, the ΔT6SS mutant induced less caspase 1 activation, secretion of IL-1β, and cytotoxicity than CF74 in Casp11−/− relative to WT BMMs (Figure 3A–3C). Inhibition of caspase 1 or challenge with the ΔT6SS mutant led to very low levels of IL-1β secretion (Figure 3B), consistent with the predominant role the T6SS and caspase 1 in inflammasome activation (Figure 3B). In contrast, CF74-induced caspase 11 activation was not affected by treatment with the caspase 1 inhibitor or in the ΔT6SS mutant (Supplementary Figure 3F). These data indicated that CF74 T6SS mainly activated caspase 1 during CF74 infection of macrophages (Figure 3A–3C, and Supplementary Figure 3F).
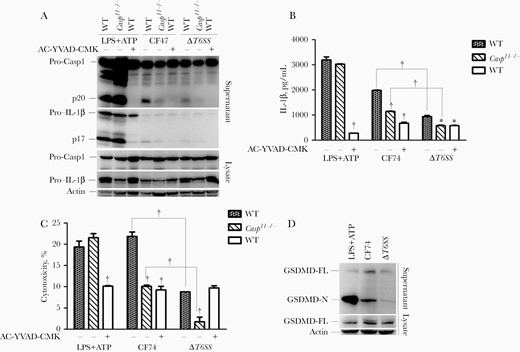
CF74 type VI secretion system (T6SS) mainly activated caspase 1 and released the cleaved gasdermin-N domain by macrophages. Bone marrow–derived macrophages (BMMs) from the indicated C57BL/6 mice were infected with the CF74 and isogenic ΔT6SS mutant for 4 hours at a multiplicity of infection of 10. A, Interleukin 1β (IL-1β) secretion and caspase 1 activation in the BMMs infected with the CF74 and isogenic ΔT6SS mutant were analyzed by immunoblotting. B, C, Quantification of IL-1β secretion (B) and lactate dehydrogenase release (C). Abbreviations: AC-YVAD-CMK, acetyl-tyrosyl-valyl-alanyl-aspartyl chloromethylketone; ATP, adenosine triphosphate; LPS, lipopolysaccharide; lysate, whole-cell lysates; p17, IL-1β secretion; p20, activated caspase 1; Pro-Casp1, pro–caspase 1, WT, wide type-, no AC-YVAD-CMK added; +, AC-YVAD-CMK added. Error bars represent standard deviations from ≥3 determinations. *P < .05; †P < .01 (analysis of variance). LPS + ATP served as positive control. D, Immunoblot analysis of cleaved gasdermin D (GSDMD) in culture supernatants and full-length GSDMD. Abbreviations: GSDMD-FL, full-length GSDMD; GSDMD-N, cleaved gasdermin-N domain.
Caspase 8 is also a known to modulate IL-1β production via NLRP3 inflammasome activation [29]. We were unable to source caspase 8–deficient mice for BMM experiments and instead performed Western blot analysis to detect activation of caspase 8 using WT BMMs. As shown in Supplementary Figure 3G, comparable and very weak caspase 8 signals could be detected in WT BMMs infected with CF74 or ΔT6SS mutant. These data indicate that caspase 8 does not play a significant role in NLRP3 inflammasome activation induced by the T6SS during CF74 infection of macrophages.
We performed CFU counts of intracellular bacteria inside the WT BMMs infected with CF74 or ΔT6SS mutant to rule out the possibility that the increased inflammasome activation was not due to increased efficiency of infection. After 4 hours of infection, the CFU counts of intracellular bacteria inside BMMs were similar for CF74 and its ΔT6SS mutant (Supplementary Figure 3H).
We further determined difference in cleavage of GSDMD, which acts as a generic substrate for caspase 1–mediated pyroptosis [30]. Western blot on GSDMD was performed in WT BMMs infected with CF74 or ΔT6SS mutant. The ΔT6SS mutant induced significantly less cleaved gasdermin-N domain release than CF74 (Figure 3D), suggesting that GSDMD was involved in the IL-1β release by BMMs and T6SS-mediated cytotoxicity. Together, the results indicated that the T6SS mainly activated caspase 1 and mediated pyroptosis by releasing the cleaved gasdermin-N domain during CF74 infection of macrophages.
CF74 T6SS Contribution to Flagellin Secretion
We previously showed that deletion of the T6SS affected FliC secretion [15]. To confirm this finding, we constructed a ΔT6SSΔfliF/pfliC double mutant in which both T6SS and flagella secretion systems were inactivated but contained a plasmid expressing fliC under the control of an arabinose-inducible promoter. Immunoblotting of lysates of arabinose-induced cells showed that FliC was expressed at similar levels in the ΔT6SSΔfliF/pfliC, ΔT6SSΔfliF/pfliCpT6SS, and ΔfliF/pfliC strains (Figure 4A). However, secretion of FliC was detected in the supernatants of ΔT6SSΔfliF/pfliCpT6SS and ΔfliF/pfliC but not in ΔT6SSΔfliF/pfliC (Figure 4A). The expression and secretion of FliC were lower in ΔT6SS (Figure 4A), confirming previous results [15]. Therefore, in the absence of a functional flagella secretion apparatus, FliC export may still occur via the T6SS.
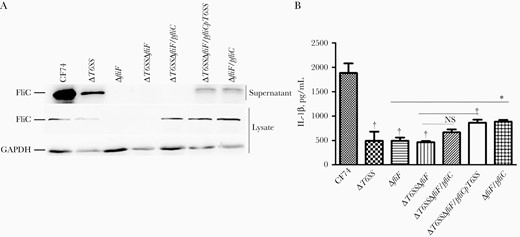
CF74 type VI secretion system (T6SS) contributes to flagellin secretion. A, FliC expression and secretion was analyzed by immunoblotting in supernatants and whole-cell lysates of Citrobacter freundii CF74 wide-type and mutant strains. B, Bone marrow–derived macrophages from the indicated C57BL/6 mice were infected with the CF74, isogenic mutants and complements for 4 hours at a multiplicity of infection of 10. Quantification of interleukin 1β (IL-1β) secretion is shown; error bars represent standard deviations from ≥3 determinations. Abbreviations: GAPDH, glyceraldehyde-3-phosphate dehydrogenase; FliC, flagellin; NS, not significant. *P < .05; †P < .01 (analysis of variance).
WT and mutant CF74 strains were used to infect mice macrophages. The induction of secretion of IL-1β by mice macrophages was significantly reduced in ΔT6SS-, ΔfliF-, and ΔT6SSΔfliF-infected samples (Figure 4B), but we were unable to detect any difference between them, most likely owing to the low levels of released IL-1β. IL-1β secretion was partially restored in ΔT6SSΔfliF/pfliCpT6SS and ΔfliF/pfliC strains (Figure 4B), but not in the ΔT6SSΔfliF/pfliC strain (Figure 4B). As expected, the TNF-α levels released by mice macrophages infected with WT CF74 and the various mutant strains were comparable (Supplementary Figure 3I). Therefore, the T6SS contributed to flagellin secretion and subsequent release of IL-1β by CF74-infected macrophages.
Hcp-2 Triggering of IL-1β Secretion From Macrophages
The transenvelope complex of the T6SS consists of stacked Hcp protein hexamers, which are capped by the VgrG protein. Moreover, Hcp acts not only as secreted effector proteins but also as machine components [31]. The CF74 T6SS Hcp-2 substrate induces cytotoxicity of host cells [15]. To determine the contribution of Hcp-2 to T6SS-mediated IL-1β release, CF74 wide-type and hcp-2 isogenic mutant were used to infect WT BMMs. The Δhcp-2 mutant showed a decreased ability of activating caspase 1 and induced less secretion of IL-1β and cytotoxicity in BMMs (Figure 5A). This defect was partially transcomplemented in the Δhcp-2/phcp-2 strain (Figure 5A). In control experiments, the levels of TNF-α released by BMMs infected with the various strains were comparable (Supplementary Figure 4). These results indicated that the Hcp-2 contributed to CF74-induced IL-1β secretion and caspase 1 activation in BMMs.
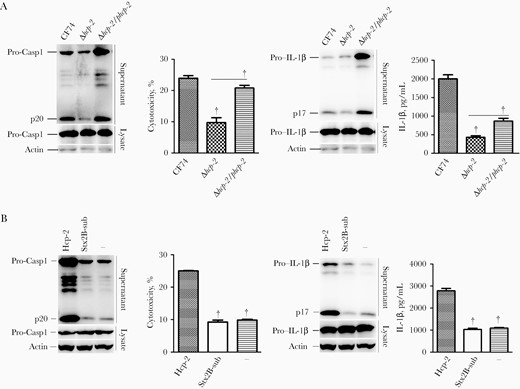
Hcp-2 triggers interleukin 1β (IL-1β) secretion from macrophages. Bone marrow–derived macrophages from the indicated C57BL/6 mice were infected with Citrobacter freundii CF74 or isogenic mutants and complements for 4 hours at a multiplicity of infection of 10 or were transfected with Hcp-2 or Shiga toxin B unit 2 subunit (StxB2-sub) proteins, or Lipofectamine 3000 reagent alone for 16 hours. Immunoblotting was used to analyze caspase 1 activation and IL-1β secretion. Abbreviations: Lysate, whole-cell lysates; p17, IL-1β secretion; p20, activated caspase 1; Pro-Casp1, pro–caspase 1. Quantification of lactate dehydrogenase release and IL-1β secretion is shown; error bars represented standard deviations from ≥3 determinations. †P < .01 (analysis of variance)
To determine whether Hcp-2 directly activated caspase 1, BMMs were transfected with the purified Hcp-2 protein (Materials and Methods). As shown in Figure 5B, purified Hcp-2 protein induced significantly higher levels of IL-1β release and cytotoxicity compared with the StxB2 subunit and added Lipofectamine 3000 reagent alone, used as negative controls. Caspase 1 activation was also detected in BMMs transfected with Hcp-2 (Figure 5B). Therefore, Hcp-2 triggered IL-1β secretion and cell death.
Hcp-2 Triggering of IL-1β Secretion via NLRP3 Activation
Furthermore, caspase 1 activation and IL-1β secretion as well as cytotoxicity induced by Hcp-2 but not StxB2 were significantly reduced in Casp1/11−/− and Nlrp3−/−, but not in Nlrc4−/− cells (Figure 6A). The results suggest that Hcp-2 induced NLRP3 activation to trigger IL-1β release and cytotoxicity.
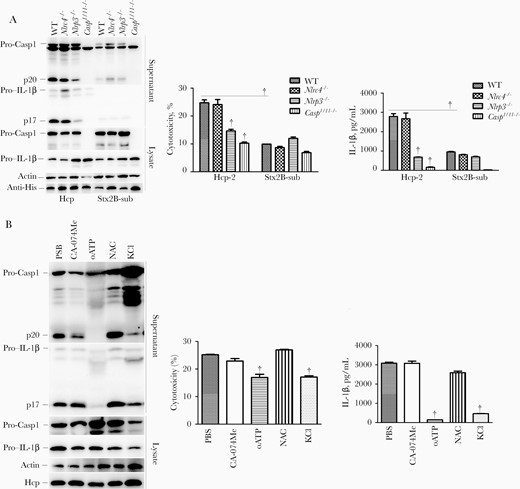
Hcp-2 triggers induction of interleukin 1β (IL-1β) secretion via activation of NLRP3. Bone marrow–derived macrophages from the indicated C57BL/6 mice were transfected with Hcp-2 or Shiga toxin B unit 2 subunit (StxB2-sub) protein for 16 hours. Immunoblotting was used to analyze caspase 1 activation and IL-1β, and anti–His tag antibody (anti-His) was used check the amount of Hcp-2 or StxB2-sub proteins in the cells. Quantification of lactate dehydrogenase release and IL-1β secretion is displayed; error bars represent standard deviations from ≥3 determinations. Abbreviations: CA-074 Me, CA-074 methyl ester; KCl, potassium chloride; lysate, whole-cell lysates; NAC, N-acetyl-L-cysteine; oATP, organic anion transporting polypeptide (OATP); p17, IL-1β secretion; p20, activated caspase 1; PBS, phosphate-buffered saline; Pro-Casp1, pro–caspase 1. †P < .01 (analysis of variance).
Multiple signals have been reported to trigger NLRP3 inflammasome activation, including K+ efflux, extracellular ATP, and ROS [8]. To investigate the role of K+ efflux, we added KCl to the cell culture medium to block K+ efflux before transfection with Hcp-2 protein. KCl significantly inhibited IL-1β section and caspase 1 activation (Figure 6B). The P2X7R inhibitor oATP almost completely inhibited Hcp-2–mediated IL-1β release and caspase 1 activation (Figure 6B). However, pretreatment with the ROS inhibitor N-acetyl-L-cysteine and cathepsin B inhibitor CA-074 Me did not impair Hcp-2–induced IL-1β release and caspase 1 activation (Figure 6B). These results indicated that Hcp-2 induced the NLRP3 inflammasome activation through K+ efflux and extracellular ATP signaling pathways.
T6SS Induction of IL-1β Secretion and Promotion of Bacterial Survival in Mice In Vivo
To investigate the inflammasome response induced by T6SS of C. freundii infection in vivo, we challenged C57BL/6 mice with various bacterial strains by intraperitoneal injection. As shown in Figure 7A, CF74ΔT6SS induced significantly lower levels of IL-1β release in PLF than WT CF74. In contrast, IL-1β release was comparable for CF74ΔT6SS/pT6SS- and CF74-infected mice (Figure 7A). As expected, TNF-α levels were comparable in PLF in mice infected with the various bacterial strains (Supplementary Figure 5).
![Type VI secretion system (T6SS) induces interleukin 1β (IL-1β) secretion and contributes to Citrobacter freundii CF74 virulence in mouse model in vivo. Six-week-old age- and sex-matched wide type (WT) C57BL/6 mice were injected intraperitoneally with phosphate-buffered saline (PBS), CF74 wide-type, ΔT6SS, and ΔT6SS/pT6SS at the indicated dose of bacteria (2 × 107 colony-forming units [CFUs] per mouse). A, IL-1β secretion. Groups of 10 C57BL/6 mice were infected by intraperitoneal injection with CF74 wide type, ΔT6SS, and ΔT6SS/pT6SS at the indicated dose of bacteria (1 × 108 CFUs per mouse) and monitored for survival over a 90-hour period. *P < .05; †P < .01 (analysis of variance). B, ΔT6SS has lower virulence in the mice model compared with WT. The percentage of surviving animals (10 mice per group) was calculated every 15 hours. *P < .05 (log-rank analysis).](https://oup.silverchair-cdn.com/oup/backfile/Content_public/Journal/jid/223/12/10.1093_infdis_jiaa692/1/m_jiaa692f0007.jpeg?Expires=1750269082&Signature=PaBAhBf263DO4XvSBaYoYu~Op1Zv1HRS~BRcn4abiPrkh61SlJF6qhso4bVB03i7ykXNB02wxGb07aCIsTGFtW6geQTSE3hXObl7U326Qzz7Gk0UP3FpbH6U3dXjFW64BxUGTapGGhK5JccydnmU-JF~9nC0KiSfpyI7Cjjy-TqDmmkPjzxUpfR9~lov6dLn5XKXaUFITksK2rQwefQqjzWnZeqfpq-Ng~~e8NlqZqh9UUgDvYr5QupTy3NlYBHocholnPAv8EpbbL6agpcaVaYRabALAcgBG5ldCr5i~C4Bd22CmU5LtTpuRCDfVxwdnCRaSxIivROqYtD5btRDNA__&Key-Pair-Id=APKAIE5G5CRDK6RD3PGA)
Type VI secretion system (T6SS) induces interleukin 1β (IL-1β) secretion and contributes to Citrobacter freundii CF74 virulence in mouse model in vivo. Six-week-old age- and sex-matched wide type (WT) C57BL/6 mice were injected intraperitoneally with phosphate-buffered saline (PBS), CF74 wide-type, ΔT6SS, and ΔT6SS/pT6SS at the indicated dose of bacteria (2 × 107 colony-forming units [CFUs] per mouse). A, IL-1β secretion. Groups of 10 C57BL/6 mice were infected by intraperitoneal injection with CF74 wide type, ΔT6SS, and ΔT6SS/pT6SS at the indicated dose of bacteria (1 × 108 CFUs per mouse) and monitored for survival over a 90-hour period. *P < .05; †P < .01 (analysis of variance). B, ΔT6SS has lower virulence in the mice model compared with WT. The percentage of surviving animals (10 mice per group) was calculated every 15 hours. *P < .05 (log-rank analysis).
To determine the contribution of T6SS to C. freundii virulence in the mouse model, the WT C. freundii CF74, CF74ΔT6SS, or CF74ΔT6SS/pT6SS strains were used to infect mice. Groups of 10 C57BL/6 mice were infected by intraperitoneal injection with 1 × 108 CFUs of bacteria and monitored for survival over a 90-hour period. We observed a significant difference in the survival of mice infected with CF74 versus its ΔT6SS mutant as early as 15 hours after infection (Figure 7B).
DISCUSSION
T6SS has been reported to regulate inflammasome activation [32–34]. In the current study, we found that the T6SS triggered NLRP3-dependent activation of caspase 1 and mediated pyroptosis by releasing the cleaved gasdermin-N domain during CF74 infection of macrophages. The functions of T6SS effectors remain poorly characterized. In addition to the Hcp and Vgr family effectors, only 5 T6SS effectors have been reported [3, 35–39]. When delivered into host cells, Hcp and Vgr orthologs were shown to promote apoptosis or prevent phagocytosis by macrophages, highlighting the importance of T6SS in virulence [32, 40–42]. As opposed to the E. tarda T6SS effector EvpP, to our knowledge, our study is the first to report that the T6SS effector Hcp-2 of C. freundii activates the NLRP3 inflammasome. How these multifaceted functions are coordinated and whether other C. freundii T6SS effectors also contribute to inflamasomme activation demand further study.
Hcp family proteins (including Hcp-1 and Hcp-2) are localized in the bacterial outer membrane [43]. In our previous studies, we showed that C. freundii CF74 Hcp-2 was involved in cell adhesion to host cells and induced cytotoxicity [2]. Hcp1 of E. coli strain RS218 induced interleukin 6 and 8 release, cytoskeletal rearrangement, and apoptosis in human brain microvascular endothelial cells [44]. In Aeromonas hydrophila, Hcp is translocated into the host cytoplasm, induces apoptosis via caspase 3 activation [43] and prevents phagocytosis by macrophages [45]. We show here that C. freundii Hcp-2 activates the NLRP3 inflammasome and induces cytotoxicity in macrophages, suggesting that Hcp-2 triggers IL-1β secretion via NLRP3 inflammasome activation.
Different bacterial secretion systems have been reported for the translocation of extracellular flagellin into the host cell cytoplasm. In Salmonella and Legionella, extracellular flagellin is translocated into the host cell cytoplasm to activate the NLRC4 inflammasome through the SPI-1 type III and the Dot/Icmf type IV secretion systems, respectively [46]. In this study, we found that the flagellin of C. freundii was secreted by T6SS and triggered subsequent release of IL-1β by CF74-infected macrophages.
K+ efflux has emerged as a common denominator in the activation of the NLRP3 inflammasome. Previous studies showed that K+ efflux is critical for activation of the NLRP3 inflammasome induced by ATP, nigericin, pore-forming toxin, particulate matter, and lowering cytosolic K+ concentration [12, 14]. Furthermore, a high concentration of extracellular K+ blocks NLRP3 inflammasome activation [14], and sensing of double-stranded RNA in the cytosol induces a signaling pathway that activates the NLRP3 inflammasome by inducing membrane permeabilization and K+ efflux [12]. In this study, Hcp-2 protein induced the NLRP3 inflammasome activation through K+ efflux.
It has been proposed that the opening of an unspecific pore formed by the hemichannel pannexin 1 after P2X7R stimulation by ATP is necessary for NLRP3 activation [15, 47, 48]. Exogenous ATP is also involved in the intracellular delivery of bacterial molecules through a pore formed by P2X7R and the hemichannel protein pannexin 1, resulting in caspase 1 activation [12, 14]. In our study, Hcp-2 protein induced NLRP3 inflammasome activation through the extracellular ATP signaling pathway.
We show that the CF74 T6SS induced inflammasome-dependent cytotoxicity was due to pyroptosis mediated by caspase 1. Caspase 1, as well as caspase 4/5/11, is known to mediate pyroptosis through the cleavage of GSDMD [30, 49]. Furthermore, cleaved GSDMD triggers NLRP3-dependent activation of caspase 1 through a cell-intrinsic pathway [30, 49, 50]. Pyroptosis plays an important role in antibacterial innate immune defense and lethal endotoxemia [49]. Our study elucidated an important mechanism of C. freundii infection and pathogenesis through the T6SS and its effectors.
In summary, we found that the cytotoxic and aggregative C. freundii strain CF74 activated the NLRP3 inflammasomes via T6SS, causing pyroptosis, and the T6SS effector Hcp-2 triggers IL-1β secretion via NLRP3 inflammasome activation through K+ efflux and extracellular ATP signaling pathways. The T6SS promoted the survival of C. freundii in mice and induced IL-1β secretion in vivo. Our study has shed light on the role of T6SS in the pathogenesis of the C. freundii infection.
Supplementary Data
Supplementary materials are available at The Journal of Infectious Diseases online. Consisting of data provided by the authors to benefit the reader, the posted materials are not copyedited and are the sole responsibility of the authors, so questions or comments should be addressed to the corresponding author.
Notes
Acknowledgments. We are grateful to Feng Shao for providing the Casp11−/− mice, Vishva M. Dixit for providing the Nlrc4−/− mice, and Warren Strober for providing the Nlrp3−/− mice.
Disclaimer. The funders had no role in study design, data collection and analysis, interpretation of data, the decision to publish, or preparation of the manuscript.
Financial support. This work was supported by the National Natural Science Foundation of China (grants 81301401 to L. L. and 81371761 to Z. R.), the State Key R&D Program of China (grants 2019YFC 1200500 and 1200505 to L. L.), and the Shanghai Municipal Science and Technology Major Project (grant 2019SHZDZX02 to G. M.).
Potential conflicts of interest. All authors: No potential conflicts. All authors have submitted the ICMJE Form for Disclosure of Potential Conflicts of Interest. Conflicts that the editors consider relevant to the content of the manuscript have been disclosed.
References
Author notes
These authors L. L., L. S., R., D., and R. L. contributed equally to this work.