-
PDF
- Split View
-
Views
-
Cite
Cite
Sujata Balasubramanian, Rifat S Rahman, Chanthap Lon, Christian Parobek, Ratawan Ubalee, Nicholas Hathaway, Worachet Kuntawunginn, Mok My, Dav Vy, Jeremy Saxe, Charlotte Lanteri, Feng-Chang Lin, Michele Spring, Steven R Meshnick, Jonathan J Juliano, David L Saunders, Jessica T Lin, Efficient Transmission of Mixed Plasmodium falciparum/vivax Infections From Humans to Mosquitoes, The Journal of Infectious Diseases, Volume 221, Issue 3, 1 February 2020, Pages 428–437, https://doi.org/10.1093/infdis/jiz388
- Share Icon Share
Abstract
In Southeast Asia, people are often coinfected with different species of malaria (Plasmodium falciparum [Pf] and Plasmodium vivax [Pv]) as well as with multiple clones of the same species. Whether particular species or clones within mixed infections are more readily transmitted to mosquitoes remains unknown.
Laboratory-reared Anopheles dirus were fed on blood from 119 Pf-infected Cambodian adults, with 5950 dissected to evaluate for transmitted infection. Among 12 persons who infected mosquitoes, polymerase chain reaction and amplicon deep sequencing were used to track species and clone-specific transmission to mosquitoes.
Seven of 12 persons that infected mosquitoes harbored mixed Pf/Pv infection. Among these 7 persons, all transmitted Pv with 2 transmitting both Pf and Pv, leading to Pf/Pv coinfection in 21% of infected mosquitoes. Up to 4 clones of each species were detected within persons. Shifts in clone frequency were detected during transmission. However, in general, all parasite clones in humans were transmitted to mosquitoes, with individual mosquitoes frequently carrying multiple transmitted clones.
Malaria diversity in human hosts was maintained in the parasite populations recovered from mosquitoes fed on their blood. However, in persons with mixed Pf/Pv malaria, Pv appears to be transmitted more readily, in association with more prevalent patent gametocytemia.
In Southeast Asia, it is not uncommon for persons with malaria to be simultaneously coinfected with Plasmodium falciparum and Plasmodium vivax (Pf/Pv). In addition, those with Pv infection frequently harbor more than 1 genetically distinct strain or clone [1, 2]. However, finding wild mosquitoes coinfected with Pf/Pv is rare [3, 4], and limited study of genetic diversity in mosquitoes suggests a propensity for clonal transmission and inbreeding [5]. These findings raise the question of whether certain species or clones are favored during transmission.
Humans transmit malaria to Anopheles mosquitoes when the latter ingest a blood meal containing gametocytes. Gametocytes are sexual stage parasites that once activated, form oocysts in the mosquito midgut, which later rupture to release thousands of sporozoites that are delivered to another human host in the mosquito’s next blood meal. After replicating to great numbers in the human host, parasite numbers reach a nadir at the mosquito oocyst stage [6, 7]. Thus, human-to-mosquito transmission represents a bottleneck in the Plasmodium life cycle that may influence how parasite genetic diversity is transmitted through a population.
We hypothesized that a selective bottleneck exists in the mosquito that affects the transmission of species and clones within mixed species infections. To explore this question, we studied 7 persons with Pf/Pv coinfection and 5 persons with Pf monoinfection who infected Anopheles dirus mosquitoes as part of membrane feeding studies in Cambodia. Polymerase chain reaction (PCR) of individual mosquitoes was used to find the proportion of mosquitoes infected with each species after blood feeding. Amplicon deep sequencing was used to track the relative composition of individual parasite clones in human blood versus mosquitoes. We found that when transmission occurred, it was remarkably efficient, with the majority of clones from each species maintained through human-to-mosquito transmission. However, Pv appeared to be transmitted more readily from Pf/Pv-infected hosts, owing to frequent patent gametocytemia.
METHODS
Human Clinical Samples
Samples were collected in those presenting with uncomplicated malaria as part of clinical studies carried out in northwestern Cambodia [8–10]. Participants were diagnosed with mono-Pf or mixed Pf/Pv infection by microscopy, with confirmation by PCR. Blood drawn before treatment was passed through a plasmodipur filter to collect leukocyte depleted blood or passed through a magnetic MACs (Miltenyi Biotec) system for gametocyte enrichment based on hemozoin content [11]. Deoxyribonucleic acid (DNA) was extracted using the QIAGEN Mini Blood DNA extraction kit. Molecular studies were approved by the Institutional Review Board at the University of North Carolina, the Walter Reed Army Institute of Research Institutional Review Board, and the Cambodian National Ethical Committee for Health Research.
Membrane Feeding Assays
Mosquito infectivity was measured by membrane feeding assay. Insectary-reared 5- to 8-day-old female A dirus mosquitoes were membrane-fed patient whole blood, maintained on 10% sucrose, and harvested 9 and 16 days after feeding, to isolate parasite oocyst and sporozoite stages, respectively. At day 9 after feeding, 50 mosquitoes per patient were dissected for oocyst counting and another 30–50 were preserved in 95% ethanol, individually for mixed infections and in groups of 10 for monoinfections (Supplementary Figure S1). Mosquitoes harvested at day 9 were preserved whole, whereas only heads and thoraces of mosquitoes harvested at day 16 were preserved in 95% ethanol. The DNA extraction from mosquitoes was performed using a simplified chelex protocol [12]. Species-specific Pf and Pv real-time PCR (qPCR), targeting the 18s ribosomal ribonucleic acid gene, was performed as previously described [13, 14]. In a given subject, successful human-to-mosquito transmission was defined as a positive qPCR result in at least 1 of 30 blood-fed mosquitoes (or 1 of 5 pools of 10 mosquitoes) at both day 9 and day 16 postfeeding, corresponding to the presence of oocysts and sporozoites, respectively.
Amplicon Deep Sequencing
Polymerase chain reaction amplification of Pf apical membrane antigen 1 (PfAMA1) and/or Pv merozoite surface protein 1 (PvMSP1) was carried out in duplicate from each DNA sample using Roche Fast Start Taq DNA Polymerase. Amplified targets were as follows: for PfAMA1, a 170-base pair (bp) fragment corresponding to domain 2 [15]; for PvMSP1, a 117-bp variable portion of the 33-kDa subunit of the 42-kDa region of MSP1 [16]. Deoxyribonucleic acid from leukocyte depleted or MACs-enriched blood from participants and DNA from mosquitoes identified as positive by real-time PCR were pooled or used individually as template for nested PCR using PfAMA1 or PvMSP1 primers (Supplementary Table S1). Most amplicons required nested (in the case of PvMSP1) or heminested (in the case of PfAMA1) PCR amplification to visualize PCR product on ethidium bromide-stained 1% agarose gel. Polymerase chain reaction products were column purified (PureLink Pro 96-well PCR purification kit; Thermo Fisher Scientific), quantified, pooled, then processed using the Bioo Scientific NextFlex Rapid DNA sequencing kit or NEBNext Ultra DNA Library Prep Kit. Sequencing was performed on an Illumina MiSeq using 150-bp paired-end chemistry.
Haplotype Determination From Deep Sequencing
Analysis of sequences was done using SeekDeep version 2.3.4 (http://seekdeep.brown.edu/) [17]. Sequences were initially demultiplexed and filtered by fragment size and base call quality. To avoid false-positive haplotype calls, replicates were clustered, setting thresholds to eliminate variants with lower than 1% frequency for averaged cluster fraction in both replicates and at lower than 0.5% frequency within each replicate. Samples with fewer than 1000 reads in 1 replicate were also eliminated. A leniency of 1 high-quality base mismatch was allowed during clustering between 2 replicates so as to err in favor of stringency in calling haplotypes rather than overreport the multiplicity of infection (MOI). We observed good concordance in haplotype calling between PCR replicates for each sample (Supplementary Table S2).
Data Analysis
Multiplicity of infection was defined as the number of unique PfAMA or PvMSP1 haplotypes detected per isolate. The DNA alignments were generated using Clustal Omega, and figures were made with GenVison software (DNAStar, Madison, WI). Minimum spanning trees, showing the number of single-nucleotide polymorphisms (SNPs) and insertion/deletion events between haplotypes, were generated in SeekDeep and labeled using Inkscape 0.92. For multiclonal Pv infections, the proportion of sequence reads aligned to individual haplotypes were compared between blood and mosquito samples using χ 2 testing. A log base 2 transformation of the number of reads was used to reduce variance before performing a χ 2 test of size (alpha = 0.05). Other graphs and statistical analyses were made using Graph-Pad Prism 6.
RESULTS
Human-to-Mosquito Transmission of Plasmodium falciparum/Plasmodium vivax Malaria
Mixed species Pf/Pv human blood-stage infection was readily transmitted to individual mosquitoes when gametocytes of both species were present. As previously reported, laboratory-reared A dirus fed on blood from 107 Pf and 12 Pf/Pv-infected Cambodian adults yielded positive mosquito infections from 12 persons, including 5 with Pf monoinfection (Supplementary Figure S2) [8]. Among 12 subjects with Pf/Pv, 5 of 12 successfully transmitted Pv infection to mosquitoes, and 2 of 12 transmitted both Pf and Pv (Figure 1). More frequent Pv transmission reflected more prevalent Pv gametocytemia, because all transmitting subjects harbored microscopically patent gametocytemia corresponding to the species transmitted. Of the 5 Pf/Pv subjects who were noninfectious, 2 also harbored patent gametocytemia, but at low densities (<50 gametocytes/µL) (Supplementary Table S3). In general, gametocytemia seemed to correlate with the burden of mosquito infection, in terms of both oocyst prevalence and density (Figure 1 and Supplementary Table S3).
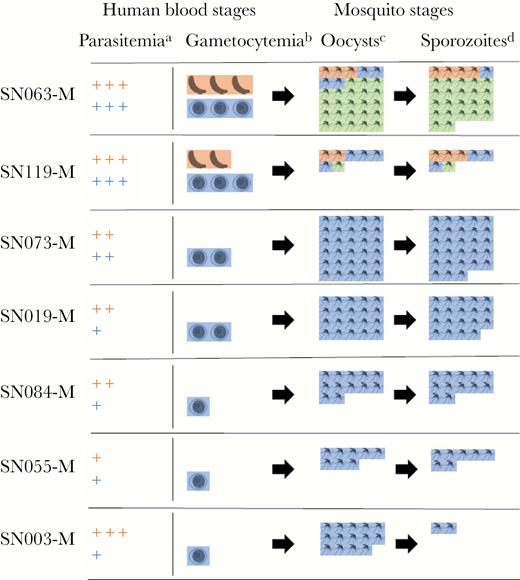
Transmission of mixed Plasmodium falciparum (Pf)/Plasmodium vivax (Pv) malaria from 7 Cambodian adults to membrane-fed Anopheles dirus mosquitoes: schematic of species-specific parasite burden. (a) Parasitemia scale. The number of + signs indicates the number of species-specific parasites/μL: 1–999 (+); 1000–9999 (++); 10 000+ (+++). (b) Gametocytemia scale. The number of gametocyte images indicates the number of species-specific gametocytes/μL: 1–49 (1); 50–149 (2); 150+ (3). (c) Oocyst-positive mosquitoes out of 30, as determined by species-specific quantitative polymerase chain reaction (qPCR) at day 9 postfeeding. (d) Sporozoite-positive mosquitoes out of 30, as determined by species-specific qPCR at day 16 postfeeding. Pf, orange; Pv, blue; Pf/Pv coinfection, green.
In 1 of the 2 subjects who transmitted both Pf and Pv (SN119), approximately one quarter of mosquitoes became infected (14 of 60, 23%), with most mosquitoes infected by either Pf or Pv (12 of 14), although 2 of 14 were infected with both species. In the other subject (SN063), almost all mosquitoes showed PCR evidence of infection (57 of 60), and the majority (45 of 57) were doubly infected (Figure 1). In total, the 12 subjects with mixed Pf/Pv malaria infected one third of mosquitoes fed on the cohort (223 of 709). Plasmodium vivax was more efficiently transmitted by these patients (211 of 709 or 30% Pv-infected mosquitoes vs 59 of 709 or 8.3% Pf-infected mosquitoes), corresponding to the more frequent presence of microscopic Pv gametocytemia (8 of 12 patients with patent Pv gametocytes (Pvg) vs 3 of 12 patients with patent Pf gametocytes). When both Pf and Pv gametocytes were present, doubly infected mosquitoes were not uncommon, making up 21% (47 of 223) of all infected mosquitoes in the cohort.
Amplicon Deep Sequencing of Blood-Stage Infection
To compare MOI (number of coinfecting clones) in those with mixed Pf/Pv infection versus single-species infection, we applied amplicon deep sequencing to leukodepleted blood samples from 14 subjects with Pf or Pv monoinfection, in addition to the 12 subjects with mixed Pf/Pv. A median of 23 411× coverage and 33 541× coverage was achieved for PfAMA1 and PvMSP1 amplicons, respectively (Supplementary Table S2). Only those haplotypes that were present in duplicate PCRs, existed at ≥1.0% frequency within each sample, and differed by >1 SNP across replicates were counted as individual clones. This led to detection of 5 unique PfAMA1 haplotypes among 21 Pf-infected subjects and 12 PvMSP1 haplotypes among 17 Pv-infected subjects (Supplementary Figures S3 and S4). All haplotypes matched sequences in GenBank. All differed by at least 2 SNPs in their sequences, with a median of 4.5 SNPs different from the next most similar haplotype (Supplementary Figure S5). One PfAMA1 haplotype and 3 PvMSP1 haplotypes were detected only as minor variants (present at in-host frequency <20%). At least 2 of these were unlikely to be false haplotypes because they were found in multiple samples as well as in the blood-fed mosquitoes.
The presence of coinfecting species did not appear to restrict the genetic complexity of mixed infections (Figure 2). The majority of Pf/Pv subjects simultaneously harbored multiple PfAMA1 haplotypes and PvMSP1 haplotypes. Overall, the mean Pf MOI was 2.5 in the mixed Pf/Pv infections vs 2.4 in the mono-Pf infections, whereas the mean Pv MOI was 2.4 in the mixed Pf/Pv infections vs 2.4 in the mono-Pv infections. Haplotypes occurring within individual subjects were usually differentiated by several SNPs, making it unlikely that PCR error, sequencing error, or single mutations falsely elevated the measured complexity of infection (Figure 3).
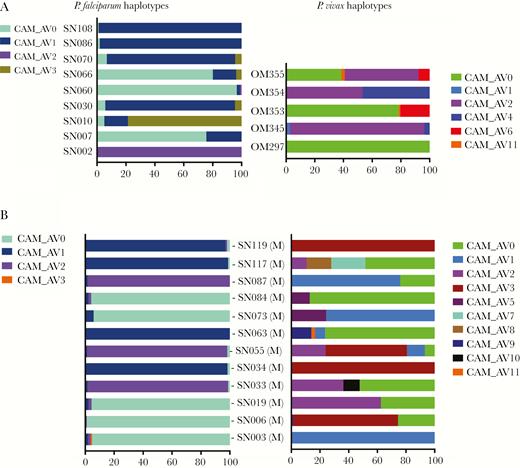
Percentage distribution of PfAMA1 and PvMSP1 haplotypes in the blood among mono-Plasmodium falciparum (Pf) and Plasmodium vivax (Pv) infections (A) and mixed Pf/Pv infections (B). Individual haplotypes (CAM_AV numbers) are represented by different colors, with width of bars corresponding to their within-host frequency (%) on the x-axis. Blood from 9 Pf, 5 Pv, and 12 Pf/Pv infections are shown. CAM_AV numbers refer to Cambodia_AnlongVeng, with number indicating the population prevalence of the haplotype (CAM_AV0 is the most common haplotype in the cohort, CAM_AV1 the next most common, etc).
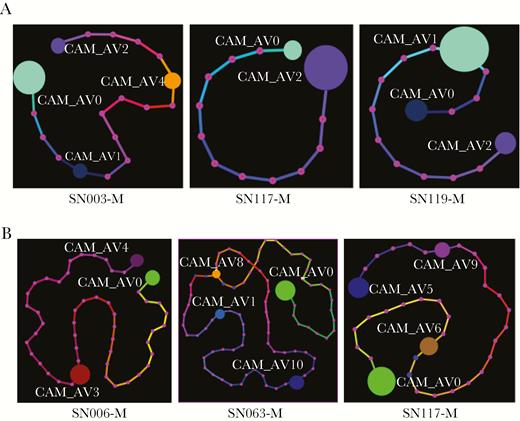
Minimum spanning trees depicting the relationship of individual PfAMA1 (A) and PvMSP1 (B) haplotypes within persons with mixed Plasmodium falciparum/Plasmodium vivax malaria. For each subject, the minimum spanning tree provides a graphical representation of the in-host frequency of each haplotype (denoted by size of colored orbs) and the number of single-nucleotide polymorphisms and insertion/deletions that separate each haplotype from the next most similar one (denoted by number of pink dots along mutational path between haplotypes).
Although MOI was similar between species, multiclonal Pv infections were composed of haplotypes with a wider range of in-host frequencies (Figure 2). The dominant PvMSP1 haplotype variant in each Pv infection accounted for a median of 76% (interquartile range [IQR], 57%–93%) of sequencing reads, whereas the dominant PfAMA1 variant in each Pf infection accounted for a median of 97% (IQR, 94%–98%) of reads.
By increasing the in-host frequency threshold at which SeekDeep called unique haplotypes from 1% up to 30%, we predicted how MOI might be measured by genotyping methods that are less sensitive at detecting minority clones (Figure 4). An increasing threshold cutoff resulted in a steady decrease in diversity and MOI due to elimination of lower frequency haplotypes. At a threshold of 10% in-host frequency, the mean Pf MOI dropped to 1.1, suggesting that most of the minor haplotypes detected would be missed by other genotyping methods.
![Measured genetic diversity in the cohort according to sensitivity of detection of minority haplotypes for Plasmodium falciparum (Pf ) (A) and Plasmodium vivax (Pv ) (B). For infections of each species (n = 21 Pf and 17 Pv), the change in the mean multiplicity of infection ([MOI] left axis) and total number of haplotypes (right axis) in the cohort is shown to decrease as the threshold frequency below which haplotypes are not called in SeekDeep is increased. Although the mean MOI of each species is the same when minority haplotypes at 1% frequency are detected, the number of Pv haplotypes is greater and the measured Pv MOI is more robust to less sensitive genotype detection.](https://oup.silverchair-cdn.com/oup/backfile/Content_public/Journal/jid/221/3/10.1093_infdis_jiz388/2/m_jiz388f0004.jpeg?Expires=1750842639&Signature=oIohwznwxy3AF0r649FnHV0phGuDUom9-a8i1GqpLpyRz24CCb-J8eRFyVmVtkpfQhhcQCHZ4sX~VPgAtkFHqplWI~lzDNmEWGyYUAyPmEL1Asxrd7qw4uiTW~lfUSDAIsuNYDwxObfu3Le2OYMs1RwIJr9Ujqz7hSmJ0fpcDCLuuQAluYCnh-sZWZP8CnecXn2Pk2~HZp0HjkPrH86F3d7dHVU8CSq8YDhMqM9vxwaAutHuT7a1SPimLe0aHB4WpwUFm6mBYfMzz-xSoE97dXhrrGAhsmptpiFz8RrR1PJ0KdT6Xy63HwHYFNygixARJVb78JlYNqt1t9TuG8kXbQ__&Key-Pair-Id=APKAIE5G5CRDK6RD3PGA)
Measured genetic diversity in the cohort according to sensitivity of detection of minority haplotypes for Plasmodium falciparum (Pf ) (A) and Plasmodium vivax (Pv ) (B). For infections of each species (n = 21 Pf and 17 Pv), the change in the mean multiplicity of infection ([MOI] left axis) and total number of haplotypes (right axis) in the cohort is shown to decrease as the threshold frequency below which haplotypes are not called in SeekDeep is increased. Although the mean MOI of each species is the same when minority haplotypes at 1% frequency are detected, the number of Pv haplotypes is greater and the measured Pv MOI is more robust to less sensitive genotype detection.
Human-to-Mosquito Transmission of Plasmodium falciparum Clones
Seven Pf-infected subjects in the cohort infected mosquitoes, including 5 with Pf monoinfection and 2 with Pf/Pv infection. In both groups, PfAMA1 haplotypes detected in the blood were generally also detected in mosquitoes. Haplotypes that were dominant in blood remained dominant in mosquitoes at both oocyst and sporozoite stages, and minority haplotypes maintained a similar frequency through transmission (Figure 5). Instances of low-level minority haplotypes (<2% frequency) disappearing in the mosquito were observed in 2 subjects but were likely due to sampling limitations. For SN119-M, low-level mosquito infection (1–2 oocysts were found in each of the 3 of 20 Pf-infected mosquitoes) resulted in only 1 infected mosquito being successfully deep-sequenced (Figure 1 and Supplementary Table S3). In contrast, for SN108, there was heavy mosquito infection (49 of 50 mosquitoes infected, each with median 149 oocysts), and all exhibited the same dominant AMA1 haplotype found in the blood (SN108).
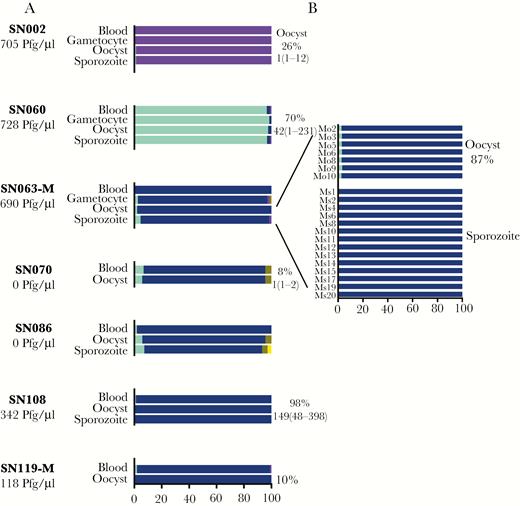
PfAMA1 haplotypes in patient blood through transmission to mosquitoes. For each subject, the composition of haplotypes and their in-host frequencies are depicted in each stage of the parasite life cycle—blood-stage in human, gametocytes isolated from blood (when available), oocysts in mosquito, and sporozoites in head and thorax of mosquito (A). Haplotypes were detected in pooled mosquito samples, except for subject SN063-M, where individual mosquitoes (Mo2 through Mo20) at oocyst and sporozoite stages were also analyzed (B). On the left, the number of gametocytes/µL of blood detected by microscopy is shown. On the right, the percentage of mosquitoes with oocyst-positive midguts is shown along with the median (range) oocyst count per midgut. The suffix -M depicts a subject with mixed Plasmodium falciparum/Plasmodium vivax infection.
In addition, minor variant gametocyte haplotypes not readily detected in the blood were found to be transmitted to mosquitoes. Deep sequencing of DNA extracted from gametocytes isolated in 3 subjects (SN002, SN060, SN063-M) revealed the same dominant PfAMA1 haplotype as that detected in the blood (Figure 5A). However, 1 gametocyte sample (SN063-M) revealed 3 minor variant haplotypes not found in the corresponding blood sample, 2 of which were also detected at low frequency in the mosquitoes (Figure 5B). Thus, haplotypes that might have appeared to be novel in the mosquito due to their absence in the blood were detected upon sequencing of gametocytes. Overall, the composition of variants in the mosquito reflected those found in the human host.
Human-to-Mosquito Transmission of Plasmodium vivax Clones
Compared with the Pf infections, the 7 Pv-transmitting subjects showcased more diversity of haplotypes in both blood and mosquito stages (Figure 6). Again, haplotypes in the blood were also detected in mosquitoes, with those that were missed in mosquito pools being detected in individual mosquitoes (SN055-M and SN063-M in Figure 6B). However, haplotype frequencies dynamically changed through transmission, especially upon examination of individual mosquitoes. Nondominant haplotypes in the blood sometimes became the dominant haplotype in individual mosquitoes (for example, Ms13 in SN019-M, Mo24 in SN055-M, and Mo24 in SN073-M). In 2 of 4 subjects with multiclonal blood-stage Pv infection, the composition of haplotype frequencies observed at the oocyst and sporozoite stages differed from that in the blood, with the dominant haplotype rising in frequency in one (SN055-M) and decreasing in another (SN063-M) (Supplementary Table S4).
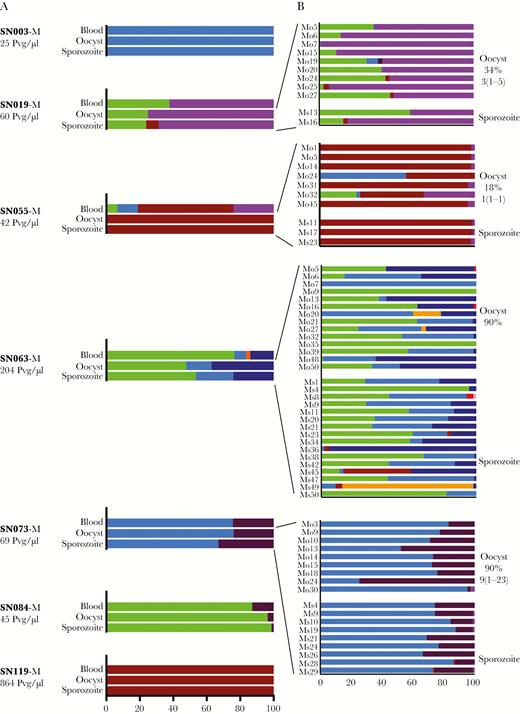
PvMSP1 haplotypes in patient blood through transmission to mosquitoes. Plasmodium vivax haplotypes within mixed infections are depicted through human-to-mosquito transmission (A). For 4 subjects, data are shown for both pooled and individual mosquitoes, with individual mosquitoes sometimes displaying haplotypes not apparent in pooled samples (B).
These shifts in haplotype frequency from blood to mosquitoes seemed to be associated with the efficiency of transmission. In 1 subject with abundant gametocytes (SN063, 204 Pvg/μL), 2 minority haplotypes in the blood (light and dark blue in Figure 6B) increased in frequency through transmission, together making up 21% of sequencing reads in the blood, but rising to 52% and 46% in pooled oocyst and sporozoite-stage mosquitoes, respectively; however, the majority haplotype in the blood (light green) remained the majority haplotype in fewer than half of individual mosquitoes. In contrast, in a subject who harbored fewer gametocytes (SN055, 42 Pvg/μL) that led to single-oocyst mosquito infections, the majority haplotype, (CAM_AV3, dark red) prevailed: although all 4 haplotypes in blood were detected in individual oocyst-stage mosquitoes, by the time parasites progressed to the sporozoite stage, only CAM_AV3 was detected in both pooled and individual mosquitoes (Figure 6). Thus, in association with a lower gametocyte burden, MOI fell from 4 in the human host to 1–2 in all but 1 of the individually sequenced mosquitoes.
Parasite Uptake in Individual Mosquitoes
Mosquitoes sampling multiclonal infections readily acquired multiple parasite haplotypes and sometimes displayed new haplotypes not detected in the blood. Even in the setting of mixed-species infection, only 3 of 69 individual A dirus sequenced at either oocyst or sporozoite stages after blood feeding displayed a single PvMSP1 haplotype by deep sequencing, with the rest acquiring multiple parasite haplotypes in a single blood meal (Figure 6B). In addition, in 16 of 70 (23%) Pv-infected mosquitoes, sequencing revealed new haplotypes not detected in the blood, usually at very low frequencies (<5%). In all but 2 mosquitoes, these new haplotypes also appeared in multiple other mosquitoes. For one Pf-infected subject (SN063), a seemingly new minority haplotype appearing in oocyst-stage mosquitoes was in fact detectable in gametocyte-enriched blood spots from the subject, as previously mentioned. For the other Pf subject in which new haplotypes appeared in the mosquito (SN086), mosquito infection arose from submicroscopic gametocytes, and gametocyte enrichment was not attempted.
DISCUSSION
In this study, we directly examine the transmission of mixed species and mixed genotype infections from humans to mosquitoes using sensitive deep-sequencing methods. Our first observation, that Pv appears to be more readily transmitted than Pf from persons coinfected with Pf/Pv is largely attributable to more prevalent Pv gametocytemia in the cohort. Our sample size is small, but this finding is consistent with previous observations from Southeast Asia that patent Pf gametocytemia is infrequent but usually necessary for Pf transmission [8, 18], whereas the Pv parasite densities that cause symptomatic infection are frequently sufficient to infect mosquitoes [19–21]. Thus, Pv enjoys a transmission advantage via early gametocytogenesis, whereby Pv gametocytes appear in tandem with the first generation of blood-stage asexual parasites, as opposed to the longer maturation period and delayed appearance of Pf gametocytes in the peripheral blood [22–24]. We show that this advantage persists in the setting of mixed infections, without evidence of interspecies competition hindering Pv transmission to mosquitoes once gametocytes are formed [25].
Second, we show that from a single blood meal from a Pf/Pv-infected person, A dirus mosquitoes frequently carry both species to life cycle completion. Impressively, wild-caught A dirus have been found to harbor as many as 3–4 different Plasmodium spp at once, including human and primate malaria species, although whether these arose from single or multiple blood meals is unknown [26]. In a recent study, a wild-caught Anopheles stephensi mosquito in Djibouti, Horn of Africa was also reported to be coinfected with Pf/Pv [27].
Finally, we observed that the malaria genetic diversity present in human blood infections is efficiently maintained through transmission to mosquitoes, with Plasmodium clones existing at very low frequencies in the blood also detected in mosquitoes. Previous studies have suggested that the capacity of mosquitoes to sample minority alleles often exceeds our capacity to detect them in the blood [28–30]. We observed new haplotypes in mosquitoes as well, but to a much lesser degree that that seen in other studies, we speculate for two reasons. First, deep sequencing was applied to leukodepleted blood samples from symptomatic subjects. A higher parasite density in the blood found with symptomatic infections combined with enrichment of parasite versus human DNA maximized the chance of capturing all circulating clones at sequencing. Second, the polyclonal infections we tracked into the mosquito were mostly due to Pv. It may be that for Pv, a greater temporal and quantitative association between asexual and sexual-stage parasitemia [20, 23] results in more proportionate representation of all gametocyte genotypes in the blood.
The maintenance of plasmodial diversity from the human to mosquito reservoir has important implications for malaria control and elimination efforts. The capacity to maintain genetic diversity through transmission enhances the parasite’s capacity to evolve in response to control interventions, including drugs, bednets, vaccines, and diagnostic tests. Previous studies have variously found substantial or negligible impact of drug resistance mutations and vaccine-induced escape on parasite survival in the mosquito [31–35]. Our small sample size and especially the limited Pf genetic diversity found at our study site in Cambodia, where intensive drug pressure has selected for specific artemisinin-resistant subpopulations of parasites [36], may not reflect the range of selective factors that may impact upon parasite fitness in the mosquito.
Despite the efficiency of mosquito sampling, changing clone frequencies through transmission was commonplace. There was some indication that a lower gametocyte density translated into preferential transmission of the dominant haplotype and decreased MOI in individual mosquitoes. Although our sample size is too small to draw conclusions, it may be that transmission of genetic diversity is maximized in the majority of gametocyte carriers, until intensive malaria interventions result in a mostly asymptomatic reservoir of very low-density infections that allows intermittent transmission of a few dominant highly transmissible parasite strains [37–39].
There are methodological limitations that will be addressed in future iterations of this work. Amplicon sequencing of a single polymorphic marker may have underestimated the level of genetic diversity present in our samples. However, MOIs calculated by these methods previously aligned well with diversity estimates from genome-wide sequencing [1, 16, 24]. The use of different genetic markers between Pf and Pv requires caution when making any comparisons between species. We did not isolate and genotype single oocysts [5, 30, 40]. Future application of multilocus deep sequencing to single clonal oocysts could help determine which clones sampled from the blood are actually meiotic siblings related by descent and thus are more likely to have arisen through single inoculation rather than superinfection from multiple mosquito bites [41–43]. Findings may well differ with different vectors in Asia versus Africa. Finally, membrane feeding may not faithfully replicate mosquito sampling via skin feeding, which is likely to be even more efficient [44], although still ultimately limited by gametocyte numbers in the blood.
CONCLUSIONS
Overall, our detailed portrait of the transmission of multiple-clone Pf/Pv malaria infections from naturally infected Cambodian adults to A dirus mosquitoes highlights the efficiency with which Plasmodium gametocytes maintain population-level genetic diversity through a vulnerable part of the malaria life cycle. Although we did observe changes in haplotype frequencies through transmission, overall, our data failed to show a selective bottleneck, although one may exist at lower gametocyte densities, such as those often found in asymptomatic carriers [45–47]. The methods shown here may yet reveal at what densities such a bottleneck appears and whether it leads to stochastic transmission of clones or a selective process that shapes population genetic diversity and response to malaria control interventions [34, 40]. Such tools and insights will be central to understanding how changes in transmission intensity [48], genetic complexity [30], and parasite densities over time will affect the evolution of drug and vaccine resistance [31, 34, 40] and the composition of the human infectious reservoir [21, 47]. The limited data presented here support the notion that more readily transmitted Pv infections with preserved genetic diversity will ultimately prove the greater challenge for malaria elimination.
Supplementary Data
Supplementary materials are available at The Journal of Infectious Diseases online. Consisting of data provided by the authors to benefit the reader, the posted materials are not copyedited and are the sole responsibility of the authors, so questions or comments should be addressed to the corresponding author.
Notes
Acknowledgments. We thank the study participants as well as the Cambodian and Thai field staff who made the study possible.
Disclaimer. The views expressed in this manuscript are those of the authors and do not reflect official policy of the Department of the Army, Department of Defense, or the United States Government.
Financial support. This work was funded by National Institutes of Health Grant K08 AI110651 (to J. T. L.) and the American Society of Tropical Medicine and Hygiene and Burroughs Wellcome Fund (postdoctoral fellowship to J. T. L.).
Potential conflicts of interest. All authors: No reported conflicts of interest. All authors have submitted the ICMJE Form for Disclosure of Potential Conflicts of Interest.