-
PDF
- Split View
-
Views
-
Cite
Cite
Silvie Huijben, Eusebio Macete, Ghyslain Mombo-Ngoma, Michael Ramharter, Simon Kariuki, Meghna Desai, Ya Ping Shi, Grace Mwangoka, Achille Massougbodji, Michel Cot, Nicaise Tuikue Ndam, Estefania Uberegui, Himanshu Gupta, Pau Cisteró, John J Aponte, Raquel González, Clara Menéndez, Alfredo Mayor, Counter-Selection of Antimalarial Resistance Polymorphisms by Intermittent Preventive Treatment in Pregnancy, The Journal of Infectious Diseases, Volume 221, Issue 2, 15 January 2020, Pages 293–303, https://doi.org/10.1093/infdis/jiz451
- Share Icon Share
Abstract
Innovative approaches are needed to limit antimalarial resistance evolution. Understanding the role of intermittent preventive treatment in pregnancy (IPTp) on the selection for resistance and the impact such selection has on pregnancy outcomes can guide future interventions.
Plasmodium falciparum isolates (n = 914) from 2 randomized clinical trials were screened for pfmdr1 copy number variation and pfcrt, pfmdr1, pfdhfr, and pfdhps resistance markers. The trials were conducted between 2010 and 2013 in Benin, Gabon, Kenya, and Mozambique to establish the efficacy of IPTp-mefloquine (MQ) compared with IPTp-sulphadoxine-pyrimethamine (SP) in human immunodeficiency virus (HIV)-uninfected and to IPTp-placebo in HIV-infected women.
In HIV-uninfected women, the prevalence of pfcrt mutants, pfdhfr/pfdhps quintuple mutants, and pfmdr1 copy number was similar between women receiving IPT-SP and IPTp-MQ. However, prevalence of pfmdr1 polymorphism 86Y was lower in the IPTp-MQ group than in the IPTp-SP group, and within the IPTp-MQ group it was lower at delivery compared with recruitment. No effect of IPTp-MQ on resistance markers was observed among HIV-infected women. The carriage of resistance markers was not associated with pregnancy outcomes.
Selection of wild-type pfmdr1 polymorphism N86 by IPTp-MQ highlights the strong selective pressure IPTp can exert and the opportunity for using negative cross-resistance in drug choice for clinical treatment and IPTp.
Drug-resistance evolution is considered one of the main challenges for malaria control and elimination and a particular challenge for special groups such as pregnant women [1–3] and human immunodeficiency virus (HIV)-infected individuals [4, 5]. Novel approaches to better manage resistance are greatly needed to avoid widespread resistance for newly introduced drugs [6, 7], particularly for prevalent interventions such as intermittent preventive therapy of malaria in pregnant women (IPTp). The antifolate drug sulphadoxine-pyrimethamine (SP), which initially replaced chloroquine (CQ) as a first-line treatment in many places, has been used in IPTp and IPT in infants (IPTi). However, resistance evolution is a threat to the continued success of the interventions [3, 5]. Although IPTi-SP is barely being implemented, IPTp-SP is still being recommended for the majority of malaria-endemic African areas [8], despite widespread resistance.
Polymorphisms in various genes have been shown to confer resistance against antimalarials. For instance, mutations in the Plasmodium falciparum dihydrofolate reductase gene (pfdhfr) result in a dihydrofolate synthase enzyme that prevents the inhibition by pyrimethamine and cycloguanil, and mutations in the P falciparum dihydropteroate synthase gene (pfdhps) lead to resistance to sulphadoxine. Polymorphisms in the P falciparum CQ resistance transporter gene (pfcrt) result in a CQ resistance transporter protein that prevents CQ accumulation within the digestive vacuole. Mutations in the P falciparum multidrug resistance 1 gene (pfmdr1) and its copy number variation have been implicated with CQ, mefloquine (MQ), and lumefantrine resistance and may compromise sensitivity to artemisinin-based combination therapies [9].
The spread of such resistant mutants depends on an intricate balance between their level of resistance, fitness cost, and the likelihood that a parasite is exposed to treatment. In addition, the interaction between resistance alleles and different drugs may impact resistance evolution: increased selection in the case of positive cross-resistance (resistance to one drug increases resistance to another drug) or decreased selection in the case of negative cross-resistance (resistance to one drug leads to hypersensitivity to another drug). In particular, this latter effect may be important to incorporate in a resistance management strategy for malaria control, because negative cross-resistance between artemether-lumefantrine (AL) and dihydroartemisinin-piperaquine (DP) have been observed [10–12]. Counter-selecting drugs are being used for resistance management in agriculture and antibiotics [13, 14], but less is known in the context of malaria. Beyond determining the level of positive and negative resistance between the different available drugs, the effect of the interactions of the drugs in the various operational uses—clinical therapy and preventive therapy such as IPTp and mass drug administration—are unknown.
When IPTp was newly introduced, increased selection of pfdhfr/pfdhps genes mutants compared with placebo groups was frequently observed [15–17]. In addition, higher SP levels in plasma of pregnant women have been associated with higher prevalence of resistance markers [18], and parasite isolates collected in early pregnancy have been shown to have lower prevalence of SP resistance-related polymorphisms than at delivery [19, 20]. The use of SP for malaria control has been drastically reduced since the introduction of artemisinin-based combination therapies, and any continued selection for SP resistance is mostly coming from its use as IPTp and the mechanistically similar antibiotic co-trimoxazole (CTX) to prevent opportunistic infections in HIV-infected individuals [21]. However, the role of such intervention on resistance evolution at the population level is not entirely clear. Resistance selection by IPTp has been shown to be of transient nature [4, 20]. Thus, the use of a drug such as SP or CTX solely in the context of preventive treatment in a limited population such as pregnant women (corresponding to approximately 5% of the population) may not be sufficient pressure for continued evolution of SP resistance [22].
Still, the impact resistance has on the effectiveness of IPTp has not been clearly established. Reduced effectiveness of IPTp-SP to clear peripheral parasites and prevent new infections during pregnancy has been observed in populations with over 90% prevalence of the pfdhps K540E polymorphism, yet, IPTp was still linked with increases in birth weight and maternal hemoglobin levels [23]. However, the pfdhps A581G polymorphism has been associated with reduced efficacy of IPTp in Uganda and Malawi [24, 25]. Most worryingly was a report that drug-resistant malaria parasites could lead to more virulent pregnancy-related infections due to a competitive facilitation effect (overgrowth of resistant parasites that out-compete sensitive parasites under drug pressure) [2], an observation not confirmed in other studies [4, 20, 26, 27]. Since then, the presence of competitive facilitation has been the center of discussion on IPTp with SP and a source of major controversy [24, 28].
In this study, we aimed to determine whether (1) IPTp-SP and IPTp-MQ during pregnancy were associated with selection for resistance alleles, (2) resistance allele-drug interactions were detected between SP and/or MQ use, and (3) carriage of resistance alleles during pregnancy was associated with adverse pregnancy outcomes.
METHODS
Study Area and Population
Plasmodium falciparum (Pf) isolates were obtained from (1) 383 HIV-uninfected pregnant women enrolled in a clinical trial aimed at assessing the efficacy of IPTp-MQ compared with IPTp-SP in Benin, Gabon, and Mozambique and (2) 83 HIV-infected women enrolled in a trial to compare the efficacy IPTp-MQ with placebo-IPTp in Kenya and Mozambique. Methods and main results of the trials conducted between 2010 and 2013 have been described elsewhere [29, 30] (trial registration number: ClinicalTrials.gov NCT00811421). The study was approved by the national and the Hospital Clínic of Barcelona ethics review committees. In brief, pregnant women attending the antenatal clinics at gestational age ≤28 weeks were recruited into the study and received long-lasting insecticide-treated bed nets. Hemoglobin levels were measured on finger prick-collected capillary blood, gestational age was determined from symphysis fundal height palpation, and HIV status was voluntarily assessed with an HIV rapid test. Among HIV-infected women, CD4+ T-cell counts were measured by flow cytometry, and viral load was determined using the COBAS AMPLICOR or AmpliPrep (Roche Diagnostics) device. Human immunodeficiency virus-uninfected pregnant women (gestational age ≥13 weeks) were randomized to receive either 2 doses of 500/15 mg SP or 2 doses of 15 mg/kg MQ. Human immunodeficiency virus-infected pregnant women (gestational age ≥13 weeks) received daily CTX (800/160 mg trimethroprim/sulfamethoxazole) and were randomized to receive either 3 doses of 15 mg/kg MQ or 3 doses of placebo.
At delivery, peripheral and placental blood smears and 50 μL blood spots on filter paper were collected, and a placental biopsy was made and examined. Newborns were weighed, and their gestational age at birth was evaluated using the Ballard's score. One month after delivery, a capillary blood spot was taken. In addition, blood spots were collected at recruitment from a subset of women in Gabon, and at recruitment, second, and third antenatal visit from a subset of women in Mozambique. Blood spots were also taken at unscheduled sick visits at the antenatal care clinic in both trials, with the exception of the sites in Benin. Uncomplicated malaria episodes were treated with oral quinine in the first trimester and AL in the second and third trimesters, and severe malaria was treated with parenteral quinine. National treatment guidelines in the respective countries was AL as first-line treatment of Pf infection in Benin, Kenya, and Mozambique and artesunate-amodiaquine in Gabon [31].
Sample Selection
Deoxyribonucleic acid (DNA) was extracted from half the blood spot using the QIAamp DNA Mini Kit (QIAGEN), and Pf parasite density was assessed using real-time polymerase chain reaction PCR as described elsewhere [32]. All delivery blood spots (peripheral and placental blood) of the HIV-infected women were analyzed by quantitative (qPCR). For HIV-uninfected women, qPCR was performed on (1) a random subset of 30% of paired peripheral and placental blood samples at delivery, (2) all smear-positive samples, and (3) samples taken within 2 months of a qPCR-positive sample, with the exception of samples from Benin where all blood samples were analyzed.
Molecular Genotyping
All Pf isolates were tested on multiplicity of infection (MOI) by PCR genotyping of Pf merozoite surface proteins 1 (msp1) and 2 (msp2) genes as described in [32]. Resistance marker testing was performed using Ligase Detection Reaction-fluorescent microsphere (LDR-fm) following the methods decribed in [33]. The polymorphisms evaluated were as follows: pfdhfr codons 51, 59, 108, and 164; pfdhps codons 436, 437, 540, 581, and 613; pfmdr1 codons 86, 184, 1034, 1042, and 1246; and pfcrt codons 72–76. Sanger sequencing was performed on pfcrt and pfdhps haplotypes, to detect missing nontargeted haplotypes by LDR-fm [34]. Finally, pfmdr1 copy number was determined relative to the single-copy β-tubulin gene by real-time PCR pfmdr1 [35]. Full details on the methods are provided in the Supplementary Information.
Definitions and Statistical Analysis
Isolates with resistance-associated polymorphisms either alone or mixed were defined as resistant. We focused on 3 polymorphic haplotypes for analysis: quintuple pfdhfr/pfdhps (polymorphisms at each of codons pfdhfr 51, 59 and 108, and pfdhps 437 and 540), pfmdr1 polymorphism 86Y, and pfcrt codons 72–76. Multiple pfmdr1 copies were considered when raw estimates of pfmdr1 copy number were ≥1.5. Recent IPTp treatment was defined as less than 75 days between last IPTp dose and sample date, clinical malaria episode was defined as presence of Pf parasites in blood smear plus at least 1 of the symptoms suggestive of malaria [29]. Differences between sites were tested by analysis of variance for continuous data and Kruskal-Wallis for malaria episodes.
Prevalence of Pf isolates with resistance markers and pfmdr1 copy numbers were compared between IPTp groups using logistic and linear generalized mixed-effect models (GLMM; “glmer” function of the “lme4” package in statistical program R [36]) including repeated measurements analysis, respectively. Study participant was included as repeated measure unit and gravidity (primigravidae/multigravidae) as covariate. Due to low sample size, repeated measures were not included in the model for HIV-infected women and General Linear Modeling was performed instead. Dependent variable was presence/absence of resistant genotype, and fixed factors were IPTp drug (MQ/SP or MQ/placebo), treatment time (early/recent), and country (Benin/Gabon/Mozambique or Kenya/Mozambique). The maximal model with all fixed effects and their interactions was fitted first, with insignificant interaction terms removed by backward elimination using F-statistics for model comparison. In a subanalysis stratified by IPTp-drug, resistance prevalence was assessed by time of sample collection (recruitment/peripheral blood delivery/postpartum) and country (Gabon/Mozambique only).
The association between resistance markers during pregnancy and pregnancy outcomes was tested using (1) resistance markers as fixed factors and (2) country, treatment, and gravidity as covariates. Models were fitted with Gaussian distribution for continuous data (birth weight, hemoglobin level, gestational age, and log-transformed parasite densities), Poisson distribution for counts (malaria episodes), and binomial distribution for events (presence/absence placental inflammation, defined by presentation of >5 inflammatory cells by histological examination). All analyses were adjusted for multiple comparisons by Bonferroni correction and adjusted P values are presented. Models were performed in R version 3.3.3 [37].
RESULTS
A total of 914 samples, from 466 women, were identified as qPCR positive for Pf: 326, 301, 77, and 210 from Benin, Gabon, Kenya, and Mozambique, respectively. Among these samples, 133 were collected at recruitment, 238 were collected at delivery from peripheral blood, 262 were collected at delivery from placental blood, 155 were collected at 1 month postpartum, 118 were collected at sick visits, and 8 were collected from antenatal visits between recruitment and delivery. Of these 914 samples, 843 isolates (92%) were genotyped on at least 1 locus (Supplementary Table S3). Sixty-five percent of the isolates were from HIV-uninfected women treated with IPTp-MQ (compared with 67% of IPTp-MQ in the overall clinical trial; χ 2 = 0.2; P = .63) and 34% in the HIV-infected women (compared with 50% IPTp-MQ in the clinical trial; χ 2 = 10; P = .001). Baseline characteristics of the study participants are shown in Supplementary Table S4.
No differences in birth weight, placental parasite density, and maternal hemoglobin levels at delivery were observed between the different study sites in the trial in HIV-uninfected women, but differences in peripheral parasite densities, gestational age of newborn, and number of malaria episodes were seen between the sites. Pregnancy outcomes amongst the HIV-infected women were similar between women from Kenya and Mozambique, with the exception of higher maternal hemoglobin concentration levels at delivery in Kenya compared with Mozambique. Multiclonal infections were common at all sites, although average MOI was low, ranging from 2.2 to 2.7 (Table 1).
Clinical and Parasitological Data of Women Included in Study (at Least One Polymorphism Successfully Analyzed)a
. | HIV Uninfected . | HIV Infected . | |||||
---|---|---|---|---|---|---|---|
Health Parameter . | Benin n = 186 . | Gabon n = 120 . | Mozambique n = 77 . | P Value . | Kenya n = 51 . | Mozambique n = 32 . | P Value . |
Gestational age (weeks) | 39.8 (1.5) | 37.4 (4.7) | 37.8 (2.4) | P < .001 (aov) | - | 38.7 (2.2) | - |
Newborn birth weight (gram) | 3013 (416) | 2899 (520) | 3001 (425) | P = .34 (aov) | 3023 (509) | 3079 (410) | P = .60 (aov) |
Hemoglobin level (g/dL) | 10.9 (1.6) | 10.5 (1.4) | 10.6 (1.7) | P = .056 (aov) | 11.3 (2.4) | 10.3 (1.7) | P = .026 (aov) |
Peripheral parasite density (pars/µL blood) | 2056 (5737) | 498 (1968) | 594 (1967) | P = .02 (aov) | 1112 (2883) | 8018 (20 305) | P = .11 (aov) |
Placental parasites density (pars/µL blood) | 2290 (9209) | 1768 (6630) | 6048 (25 750) | P = .40 (aov) | 2395 (6502) | 7110 (18 472) | P = .41 (aov) |
Clinical malaria episode during pregnancy (%women) | 19.3 | 44.5 | 30.8 | P < .001 (chi2) | 24.7 | 29.8 | P = .68 (kw) |
Number of malaria episodes | 0.23 (0.5) | 0.48 (0.6) | 0.31 (0.5) | P < .001 (kw) | 0.25 (0.43) | 0.36 (0.61) | P = .42 (kw) |
Multiplicity of infection | 2.2 (1.4) | 2.4 (1.5) | 2.6 (1.6) | P = .028 (aov) | 2.3 (1.4) | 2.7 (1.7) | P = .16 (aov) |
. | HIV Uninfected . | HIV Infected . | |||||
---|---|---|---|---|---|---|---|
Health Parameter . | Benin n = 186 . | Gabon n = 120 . | Mozambique n = 77 . | P Value . | Kenya n = 51 . | Mozambique n = 32 . | P Value . |
Gestational age (weeks) | 39.8 (1.5) | 37.4 (4.7) | 37.8 (2.4) | P < .001 (aov) | - | 38.7 (2.2) | - |
Newborn birth weight (gram) | 3013 (416) | 2899 (520) | 3001 (425) | P = .34 (aov) | 3023 (509) | 3079 (410) | P = .60 (aov) |
Hemoglobin level (g/dL) | 10.9 (1.6) | 10.5 (1.4) | 10.6 (1.7) | P = .056 (aov) | 11.3 (2.4) | 10.3 (1.7) | P = .026 (aov) |
Peripheral parasite density (pars/µL blood) | 2056 (5737) | 498 (1968) | 594 (1967) | P = .02 (aov) | 1112 (2883) | 8018 (20 305) | P = .11 (aov) |
Placental parasites density (pars/µL blood) | 2290 (9209) | 1768 (6630) | 6048 (25 750) | P = .40 (aov) | 2395 (6502) | 7110 (18 472) | P = .41 (aov) |
Clinical malaria episode during pregnancy (%women) | 19.3 | 44.5 | 30.8 | P < .001 (chi2) | 24.7 | 29.8 | P = .68 (kw) |
Number of malaria episodes | 0.23 (0.5) | 0.48 (0.6) | 0.31 (0.5) | P < .001 (kw) | 0.25 (0.43) | 0.36 (0.61) | P = .42 (kw) |
Multiplicity of infection | 2.2 (1.4) | 2.4 (1.5) | 2.6 (1.6) | P = .028 (aov) | 2.3 (1.4) | 2.7 (1.7) | P = .16 (aov) |
Abbreviations: aov, analysis of variance; chi2, χ 2 test; HIV, human immunodeficiency virus; kw, Kruskal-Wallis test; qPCR, quantitative polymerase chain reaction.
aPeripheral and placental parasite density = geometric mean parasite density as measured by qPCR; clinical malaria episodes = percentage of women with at least 1 smear-positive clinical malaria episode; number of malaria episodes = overall mean number of episodes of all participants. Means plus standard deviation are given, standard deviation of parasite density includes Taylor's expansion.
Clinical and Parasitological Data of Women Included in Study (at Least One Polymorphism Successfully Analyzed)a
. | HIV Uninfected . | HIV Infected . | |||||
---|---|---|---|---|---|---|---|
Health Parameter . | Benin n = 186 . | Gabon n = 120 . | Mozambique n = 77 . | P Value . | Kenya n = 51 . | Mozambique n = 32 . | P Value . |
Gestational age (weeks) | 39.8 (1.5) | 37.4 (4.7) | 37.8 (2.4) | P < .001 (aov) | - | 38.7 (2.2) | - |
Newborn birth weight (gram) | 3013 (416) | 2899 (520) | 3001 (425) | P = .34 (aov) | 3023 (509) | 3079 (410) | P = .60 (aov) |
Hemoglobin level (g/dL) | 10.9 (1.6) | 10.5 (1.4) | 10.6 (1.7) | P = .056 (aov) | 11.3 (2.4) | 10.3 (1.7) | P = .026 (aov) |
Peripheral parasite density (pars/µL blood) | 2056 (5737) | 498 (1968) | 594 (1967) | P = .02 (aov) | 1112 (2883) | 8018 (20 305) | P = .11 (aov) |
Placental parasites density (pars/µL blood) | 2290 (9209) | 1768 (6630) | 6048 (25 750) | P = .40 (aov) | 2395 (6502) | 7110 (18 472) | P = .41 (aov) |
Clinical malaria episode during pregnancy (%women) | 19.3 | 44.5 | 30.8 | P < .001 (chi2) | 24.7 | 29.8 | P = .68 (kw) |
Number of malaria episodes | 0.23 (0.5) | 0.48 (0.6) | 0.31 (0.5) | P < .001 (kw) | 0.25 (0.43) | 0.36 (0.61) | P = .42 (kw) |
Multiplicity of infection | 2.2 (1.4) | 2.4 (1.5) | 2.6 (1.6) | P = .028 (aov) | 2.3 (1.4) | 2.7 (1.7) | P = .16 (aov) |
. | HIV Uninfected . | HIV Infected . | |||||
---|---|---|---|---|---|---|---|
Health Parameter . | Benin n = 186 . | Gabon n = 120 . | Mozambique n = 77 . | P Value . | Kenya n = 51 . | Mozambique n = 32 . | P Value . |
Gestational age (weeks) | 39.8 (1.5) | 37.4 (4.7) | 37.8 (2.4) | P < .001 (aov) | - | 38.7 (2.2) | - |
Newborn birth weight (gram) | 3013 (416) | 2899 (520) | 3001 (425) | P = .34 (aov) | 3023 (509) | 3079 (410) | P = .60 (aov) |
Hemoglobin level (g/dL) | 10.9 (1.6) | 10.5 (1.4) | 10.6 (1.7) | P = .056 (aov) | 11.3 (2.4) | 10.3 (1.7) | P = .026 (aov) |
Peripheral parasite density (pars/µL blood) | 2056 (5737) | 498 (1968) | 594 (1967) | P = .02 (aov) | 1112 (2883) | 8018 (20 305) | P = .11 (aov) |
Placental parasites density (pars/µL blood) | 2290 (9209) | 1768 (6630) | 6048 (25 750) | P = .40 (aov) | 2395 (6502) | 7110 (18 472) | P = .41 (aov) |
Clinical malaria episode during pregnancy (%women) | 19.3 | 44.5 | 30.8 | P < .001 (chi2) | 24.7 | 29.8 | P = .68 (kw) |
Number of malaria episodes | 0.23 (0.5) | 0.48 (0.6) | 0.31 (0.5) | P < .001 (kw) | 0.25 (0.43) | 0.36 (0.61) | P = .42 (kw) |
Multiplicity of infection | 2.2 (1.4) | 2.4 (1.5) | 2.6 (1.6) | P = .028 (aov) | 2.3 (1.4) | 2.7 (1.7) | P = .16 (aov) |
Abbreviations: aov, analysis of variance; chi2, χ 2 test; HIV, human immunodeficiency virus; kw, Kruskal-Wallis test; qPCR, quantitative polymerase chain reaction.
aPeripheral and placental parasite density = geometric mean parasite density as measured by qPCR; clinical malaria episodes = percentage of women with at least 1 smear-positive clinical malaria episode; number of malaria episodes = overall mean number of episodes of all participants. Means plus standard deviation are given, standard deviation of parasite density includes Taylor's expansion.
Prevalence of Molecular Markers of Antimalarial Resistance
Genotyping was successful for all 19 polymorphisms of interest in 69% of samples (Supplementary Table S3). pfmdr1 copy number estimation was successful in 50% of samples (Supplementary Tables S3 and S5). Prevalence of wild-type pfdhfr/pfdhps haplotype NCSAKA (dhfr 51N-59C-108S and dhps 437A-540K) parasites was low. Quadruple mutant IRNGK was most common in Benin and Gabon (95% and 80%, respectively), and quintuple mutant genotype IRNGE was most common in Kenya and Mozambique (99% and 88%) (Figure 1). The pfdhps polymorphism A581G was only observed in Benin at very low prevalence (3%) on a background of quadruple IRNGK genotypes (3%). The prevalence of all genotyped polymorphisms are presented in Figure 1, Tables 2, and Supplementary Table S6. The majority of the Pf infections in this study were of single pfmdr1 copy (94%, 93%, 93%, and 84% in Benin, Gabon, Kenya, and Mozamique, respectively).
. | . | HIV Uninfected . | HIV Infected . | |||
---|---|---|---|---|---|---|
Genetic Locus . | Genotype . | Benin n/N (%) . | Gabon n/N (%) . | Mozambique n/N (%) . | Kenya n/N (%) . | Mozambique n/N (%) . |
pfdhfr 51/59/108 pfdhps 437/540 | NCSAK* (wild type) | 0/251 (0) | 1/203 (0.5) | 1/153 (0.7) | 0/76 (0) | 0/43 (0) |
NCSGK (single437) | 2/251 (0.8) | 4/203 (2.0) | 0/153 (0) | 0/76 (0) | 0/43 (0) | |
IRNAK (Triple) | 3/251 (1.2) | 22/203 (10.8) | 9/153 (5.9) | 0/76 (0) | 4/43 (9.3) | |
NRNGK (Triple437) | 4/251 (1.6) | 2/203 (1.0) | 0/153 (0) | 0/76 (0) | 0/43 (0) | |
IRNGK (Quadruple) | 239/251 (95.2) | 163/203 (80.3) | 1/153 (0.7) | 0/76 (0) | 0/43 (0) | |
IRNGE (Quintuple) | 0/251 (0) | 7/203 (3.4) | 137/153 (89.5) | 75/76 (98.7) | 36/43 (83.7) | |
Othera | 3/251 (1.2) | 4/203 (2.0) | 5/153 (3.3) | 1/76 (1.3) | 3/43 (7.0) | |
pfmdr1 86/184/1246 | NYD* | 47/247 (19.0) | 39/190 (20.5) | 48/136 (35.3) | 34/76 (44.7) | 9/41 (22.0) |
YYD | 3/247 (1.2) | 12/190 (6.3) | 22/136 (16.2) | 3/76 (3.9) | 8/41 (19.5) | |
NFD | 102/247 (41.3) | 45/190 (23.7) | 60/136 (44.1) | 32/76 (42.1) | 21/41 (51.2) | |
NYY | 0/247 (0) | 0/190 (0) | 0/136 (0) | 1/76 (1.3) | 0/41 (0) | |
YFD | 83/247 (33.6) | 94/190 (49.5) | 6/136 (4.4) | 1/76 (1.3) | 3/41 (7.3) | |
NFY | 1/247 (0.4) | 0/190 (0) | 0/136 (0) | 1/76 (1.3) | 0/41 (0) | |
YYY | 5/247 (2.0) | 0/190 (0) | 0/136 (0) | 3/76 (3.9) | 0/41 (0) | |
YFY | 6/247 (2.4) | 0/190 (0) | 0/136 (0) | 1/76 (1.3) | 0/41 (0) | |
pfcrt 72-76 | CVMNK* | 15/271 (5.5) | 31/237 (13.0) | 81/151 (53.6) | 59/76 (77.6) | 26/42 (61.9) |
CVIET | 256/271 (94.5) | 206/237 (86.9) | 70/151 (46.4) | 17/76 (22.4) | 16/42 (38.1) |
. | . | HIV Uninfected . | HIV Infected . | |||
---|---|---|---|---|---|---|
Genetic Locus . | Genotype . | Benin n/N (%) . | Gabon n/N (%) . | Mozambique n/N (%) . | Kenya n/N (%) . | Mozambique n/N (%) . |
pfdhfr 51/59/108 pfdhps 437/540 | NCSAK* (wild type) | 0/251 (0) | 1/203 (0.5) | 1/153 (0.7) | 0/76 (0) | 0/43 (0) |
NCSGK (single437) | 2/251 (0.8) | 4/203 (2.0) | 0/153 (0) | 0/76 (0) | 0/43 (0) | |
IRNAK (Triple) | 3/251 (1.2) | 22/203 (10.8) | 9/153 (5.9) | 0/76 (0) | 4/43 (9.3) | |
NRNGK (Triple437) | 4/251 (1.6) | 2/203 (1.0) | 0/153 (0) | 0/76 (0) | 0/43 (0) | |
IRNGK (Quadruple) | 239/251 (95.2) | 163/203 (80.3) | 1/153 (0.7) | 0/76 (0) | 0/43 (0) | |
IRNGE (Quintuple) | 0/251 (0) | 7/203 (3.4) | 137/153 (89.5) | 75/76 (98.7) | 36/43 (83.7) | |
Othera | 3/251 (1.2) | 4/203 (2.0) | 5/153 (3.3) | 1/76 (1.3) | 3/43 (7.0) | |
pfmdr1 86/184/1246 | NYD* | 47/247 (19.0) | 39/190 (20.5) | 48/136 (35.3) | 34/76 (44.7) | 9/41 (22.0) |
YYD | 3/247 (1.2) | 12/190 (6.3) | 22/136 (16.2) | 3/76 (3.9) | 8/41 (19.5) | |
NFD | 102/247 (41.3) | 45/190 (23.7) | 60/136 (44.1) | 32/76 (42.1) | 21/41 (51.2) | |
NYY | 0/247 (0) | 0/190 (0) | 0/136 (0) | 1/76 (1.3) | 0/41 (0) | |
YFD | 83/247 (33.6) | 94/190 (49.5) | 6/136 (4.4) | 1/76 (1.3) | 3/41 (7.3) | |
NFY | 1/247 (0.4) | 0/190 (0) | 0/136 (0) | 1/76 (1.3) | 0/41 (0) | |
YYY | 5/247 (2.0) | 0/190 (0) | 0/136 (0) | 3/76 (3.9) | 0/41 (0) | |
YFY | 6/247 (2.4) | 0/190 (0) | 0/136 (0) | 1/76 (1.3) | 0/41 (0) | |
pfcrt 72-76 | CVMNK* | 15/271 (5.5) | 31/237 (13.0) | 81/151 (53.6) | 59/76 (77.6) | 26/42 (61.9) |
CVIET | 256/271 (94.5) | 206/237 (86.9) | 70/151 (46.4) | 17/76 (22.4) | 16/42 (38.1) |
Abbreviations: HIV, human immunodeficiency virus.
aSee Supplementary Information (Supplementary Table S6) for full list. Wild-type genotypes are indicated with an asterisk, and polymorphisms are shown in bold lettering.
. | . | HIV Uninfected . | HIV Infected . | |||
---|---|---|---|---|---|---|
Genetic Locus . | Genotype . | Benin n/N (%) . | Gabon n/N (%) . | Mozambique n/N (%) . | Kenya n/N (%) . | Mozambique n/N (%) . |
pfdhfr 51/59/108 pfdhps 437/540 | NCSAK* (wild type) | 0/251 (0) | 1/203 (0.5) | 1/153 (0.7) | 0/76 (0) | 0/43 (0) |
NCSGK (single437) | 2/251 (0.8) | 4/203 (2.0) | 0/153 (0) | 0/76 (0) | 0/43 (0) | |
IRNAK (Triple) | 3/251 (1.2) | 22/203 (10.8) | 9/153 (5.9) | 0/76 (0) | 4/43 (9.3) | |
NRNGK (Triple437) | 4/251 (1.6) | 2/203 (1.0) | 0/153 (0) | 0/76 (0) | 0/43 (0) | |
IRNGK (Quadruple) | 239/251 (95.2) | 163/203 (80.3) | 1/153 (0.7) | 0/76 (0) | 0/43 (0) | |
IRNGE (Quintuple) | 0/251 (0) | 7/203 (3.4) | 137/153 (89.5) | 75/76 (98.7) | 36/43 (83.7) | |
Othera | 3/251 (1.2) | 4/203 (2.0) | 5/153 (3.3) | 1/76 (1.3) | 3/43 (7.0) | |
pfmdr1 86/184/1246 | NYD* | 47/247 (19.0) | 39/190 (20.5) | 48/136 (35.3) | 34/76 (44.7) | 9/41 (22.0) |
YYD | 3/247 (1.2) | 12/190 (6.3) | 22/136 (16.2) | 3/76 (3.9) | 8/41 (19.5) | |
NFD | 102/247 (41.3) | 45/190 (23.7) | 60/136 (44.1) | 32/76 (42.1) | 21/41 (51.2) | |
NYY | 0/247 (0) | 0/190 (0) | 0/136 (0) | 1/76 (1.3) | 0/41 (0) | |
YFD | 83/247 (33.6) | 94/190 (49.5) | 6/136 (4.4) | 1/76 (1.3) | 3/41 (7.3) | |
NFY | 1/247 (0.4) | 0/190 (0) | 0/136 (0) | 1/76 (1.3) | 0/41 (0) | |
YYY | 5/247 (2.0) | 0/190 (0) | 0/136 (0) | 3/76 (3.9) | 0/41 (0) | |
YFY | 6/247 (2.4) | 0/190 (0) | 0/136 (0) | 1/76 (1.3) | 0/41 (0) | |
pfcrt 72-76 | CVMNK* | 15/271 (5.5) | 31/237 (13.0) | 81/151 (53.6) | 59/76 (77.6) | 26/42 (61.9) |
CVIET | 256/271 (94.5) | 206/237 (86.9) | 70/151 (46.4) | 17/76 (22.4) | 16/42 (38.1) |
. | . | HIV Uninfected . | HIV Infected . | |||
---|---|---|---|---|---|---|
Genetic Locus . | Genotype . | Benin n/N (%) . | Gabon n/N (%) . | Mozambique n/N (%) . | Kenya n/N (%) . | Mozambique n/N (%) . |
pfdhfr 51/59/108 pfdhps 437/540 | NCSAK* (wild type) | 0/251 (0) | 1/203 (0.5) | 1/153 (0.7) | 0/76 (0) | 0/43 (0) |
NCSGK (single437) | 2/251 (0.8) | 4/203 (2.0) | 0/153 (0) | 0/76 (0) | 0/43 (0) | |
IRNAK (Triple) | 3/251 (1.2) | 22/203 (10.8) | 9/153 (5.9) | 0/76 (0) | 4/43 (9.3) | |
NRNGK (Triple437) | 4/251 (1.6) | 2/203 (1.0) | 0/153 (0) | 0/76 (0) | 0/43 (0) | |
IRNGK (Quadruple) | 239/251 (95.2) | 163/203 (80.3) | 1/153 (0.7) | 0/76 (0) | 0/43 (0) | |
IRNGE (Quintuple) | 0/251 (0) | 7/203 (3.4) | 137/153 (89.5) | 75/76 (98.7) | 36/43 (83.7) | |
Othera | 3/251 (1.2) | 4/203 (2.0) | 5/153 (3.3) | 1/76 (1.3) | 3/43 (7.0) | |
pfmdr1 86/184/1246 | NYD* | 47/247 (19.0) | 39/190 (20.5) | 48/136 (35.3) | 34/76 (44.7) | 9/41 (22.0) |
YYD | 3/247 (1.2) | 12/190 (6.3) | 22/136 (16.2) | 3/76 (3.9) | 8/41 (19.5) | |
NFD | 102/247 (41.3) | 45/190 (23.7) | 60/136 (44.1) | 32/76 (42.1) | 21/41 (51.2) | |
NYY | 0/247 (0) | 0/190 (0) | 0/136 (0) | 1/76 (1.3) | 0/41 (0) | |
YFD | 83/247 (33.6) | 94/190 (49.5) | 6/136 (4.4) | 1/76 (1.3) | 3/41 (7.3) | |
NFY | 1/247 (0.4) | 0/190 (0) | 0/136 (0) | 1/76 (1.3) | 0/41 (0) | |
YYY | 5/247 (2.0) | 0/190 (0) | 0/136 (0) | 3/76 (3.9) | 0/41 (0) | |
YFY | 6/247 (2.4) | 0/190 (0) | 0/136 (0) | 1/76 (1.3) | 0/41 (0) | |
pfcrt 72-76 | CVMNK* | 15/271 (5.5) | 31/237 (13.0) | 81/151 (53.6) | 59/76 (77.6) | 26/42 (61.9) |
CVIET | 256/271 (94.5) | 206/237 (86.9) | 70/151 (46.4) | 17/76 (22.4) | 16/42 (38.1) |
Abbreviations: HIV, human immunodeficiency virus.
aSee Supplementary Information (Supplementary Table S6) for full list. Wild-type genotypes are indicated with an asterisk, and polymorphisms are shown in bold lettering.
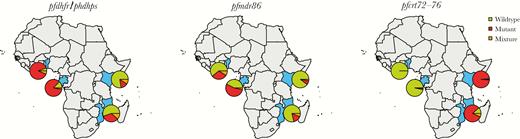
Geographical distribution of resistance markers in study. Prevalence of polymorphisms of resistance markers pooled throughout the study at the different African sites (Benin, Gabon, Kenya, and Mozambique): quintuple IRNGE pfdhfr/pfdhps (left plot), pfmdr1 polymorphism 86Y (middle plot), and pfcrt codons 72–76 haplotype CVIET (right plot). Green denotes prevalence of wild type, red denotes resistant, and orange denotes prevalence of mixtures at any single-nucleotide polymorphism in the genotype.
IPTp Selective Pressure on Resistance Markers During Pregnancy
In HIV-uninfected women, no statistically significant association was found between the carriage of pfdhfr/pfdhps quintuple mutants or pfcrt72-76 mutants and the type of IPTp drug (Padj > .99 in both cases), nor time since last IPTp administration (Padj > .99 in both cases) (Figure 2). However, women receiving IPTp-SP were more likely to carry the resistant pfmdr1 polymorphism 86Y during pregnancy than women receiving IPTp-MQ (59% vs 29%, Padj = .002) without a significant association with time since administration (Padj = .15) (Figure 2). This trend was most strongly observed in Benin and Gabon (Supplementary Figure S1). The association between the IPTp drug and each polymorphism is shown in Supplementary Table S7. There was no distinct association between prevalence of multiple pfmdr1 copies and the type of IPTp drug (P = .80), although Gabonese women taking IPTp-MQ had a slightly higher copy number than those taking IPTp-SP (P = .003), a trend that was not observed in the other countries (Supplementary Figure S2). In HIV-infected women, no differences were observed in the carriage of molecular markers of resistance between IPTp-MQ and IPTp-placebo (Padj > .05 in all 3 comparisons) (Figure 2B) nor in pfmdr1 copy numbers (IPTp-drug; P > .05) (Supplementary Figure S2).
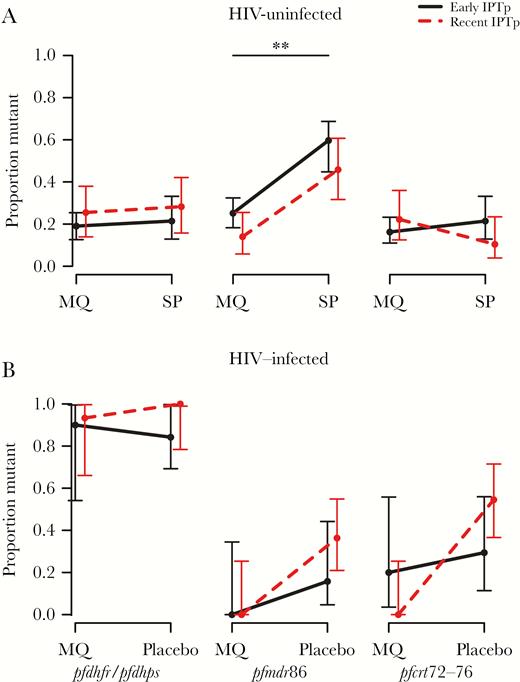
Resistance marker prevalence between treatment groups. Proportion of Plasmodium falciparum isolates at any time during pregnancy with quintuple IRNGE pfdhfr/pfdhps (left plot), pfmdr1 polymorphism 86Y (middle plot), and pfcrt codons 72–76 haplotype CVIET (right plot) collected from women receiving intermittent preventive treatment in pregnancy (IPTp)-mefloquine (MQ) and IPTp-sulphadoxine-pyrimethamine (SP) in human immunodeficiency virus (HIV)-uninfected individuals (A) and IPTp-MQ and IPTp-placebo in HIV-infected individuals (B). Solid lines show isolates with more than 2.5 months from last IPTp dose (early IPTp), and dashed lines show isolates with less than 2.5 months from last IPTp dose (recent IPTp). Data are pooled between countries (HIV-uninfected: Benin, Gabon, and Mozambique; HIV-infected: Kenya and Mozambique) and show overall mean proportion with 95% confidence intervals. Data by country are shown in Supplementary Figure S1. ** denotes significance at the Padj < .01 level.
For the subset of women of whom we had samples also at recruitment, prevalence of pfmdr1 polymorphism 86Y was lower at delivery (31%) than at recruitment (63%) in the IPTp-MQ group in Gabon and Mozambique combined (HIV-uninfected; Padj = .015), whereas no significant difference was seen in the IPTp-SP arm (35% vs 72%; Padj = .16) (Figure 3). This trend was consistent in both sites but most distinctly observed in Gabon (Supplementary Figure S3). No statistically significant difference was observed with quintuple pfdhfr/pfdhps and pfcrt72-76 mutants (Padj > .05 in all comparisons) (Figure 3). No longitudinal analyses were performed among the HIV-infected women due to small sample size, but raw data are shown in Supplementary Figure S4.
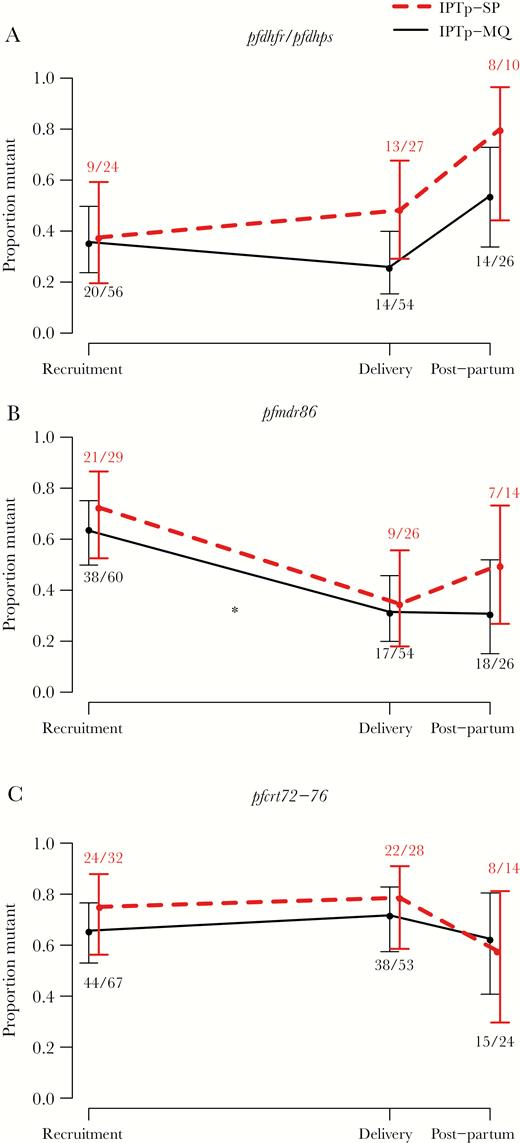
Resistance marker prevalence at different stages of pregnancy and postpartum. Change in proportion of women carrying quintuple pfdhfr/pfdhps (A), pfmdr1 polymorphism 86Y (B), and pfcrt codons 72–76 (C) resistance markers at delivery compared with recruitment (mean gestational age of 18.4 weeks) and postpartum (1 month after delivery) after intermittent preventive treatment in pregnancy (IPTp)-sulphadoxine-pyrimethamine (SP) (dashed lines) and IPTp-mefloquine (MQ) (solid lines). Data are pooled from Gabon and Mozambique. Error bars show 95% confidence intervals. Asterisks show level of statistical significance between different time points, * indicates a significant (Padj < .05) difference compared with delivery.
Associations Between Pregnancy-Related Outcomes and Resistance Markers
No associations were observed between the carriage of pfcrt, pfmdr1 86Y, and/or quintuple pfdhfr/pfdhps resistance markers at any point during pregnancy with either parasitological (peripheral and placental parasitemia) or clinical (birth weight, hemoglobin, gestational age, and number of malaria episodes) outcomes in HIV-uninfected (Padj > .05 in all comparisons [Figure 4], trends by location in Supplementary Figure S5) nor HIV-infected women (all Padj > .99) (Supplementary Figure S6). The carriage of pfdhps polymorphism A581G in pregnant women from Benin was not associated with any adverse pregnancy outcome (all Padj > .99).
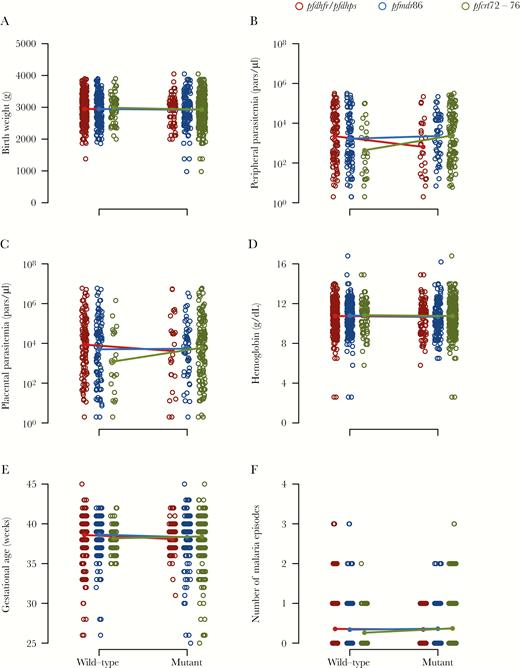
Association between resistance markers and pregnancy health markers. Wild-type and resistant genotypes of quintuple pfdhfr/pfdhps (red), pfmdr1 polymorphism 86Y (blue), and pfcrt codons 72–76 (green) of human immunodeficiency virus-uninfected women at any time during pregnancy with infant birth weight (A), mother peripheral blood (B), and placental blood (C) parasite density at delivery as determined by quantitative polymerase chain reaction, mother hemoglobin level at delivery (D), infant gestational age at birth (E), and number of clinical malaria episodes during pregnancy (F). Data are pooled from Benin, Gabon, and Mozambique, and data by country are shown in Supplementary Figure S3.
DISCUSSION
Wild-type pfmdr1 polymorphism N86 appeared to be markedly selected for by MQ treatment in HIV-uninfected pregnant women. No significant associations were observed in the HIV-infected cohort; however, the study lacks the statistical power due to a low sample size. The IPTp treatment with SP did not lead to increases in existing pfdhfr/pfdhps polymorphisms despite reported associations [4, 15, 16, 18–20]. It is possible that the short time scale (duration of pregnancy) was insufficient to observe these changes because allelic diversity at each site was very low. No association was found with increased pfmdr1 copy number and MQ use; although a significant 3-way interaction is suggestive of selection in women having received IPTp-MQ recently in Gabon. Increased pfmdr1 copy number has been shown to be associated with MQ treatment [35], but minimal variation in pfmdr1 copy numbers in the study areas may have limited the likelihood of identifying signs of selection.
Strong negative selection against pfmdr 86Y locus by IPTp-MQ was observed. Increased susceptibility to MQ of 86Y genotypes has been shown in vitro [38, 39] but to our knowledge not after clinical treatment or IPTp with MQ. Decreased susceptibility to AL, of which lumefantrine is structurally similar to MQ, has been seen for pfmdr1 N86, pfmdr1 184F, pfmdr1 D1246, and pfcrt K76 after clinical treatment [10, 11]. Thus, parasites carrying N86Y were likely more effectively eliminated by the IPTp-MQ treatment than N86 parasites. In contrast, 86Y has been associated with CQ resistance [40] and likely DP susceptibility [41]. Thus, our data further contribute to the evidence of 86Y being involved in resistance to some drugs but leading to increased susceptibility to other drugs. Such so-called resistance-induced hypersensitivity has been shown for a variety of antimalarial drugs [10–12]. In this study, we demonstrate that this effect even has pronounced shifts in allele frequencies after IPTp. Although these observations are from 2010 to 2012 and on the antimalarial drug MQ that is not recommended for IPTp, they present proof of concept that negative cross-resistance by IPTp treatment could provide a future strategy for malaria resistance management. For instance, CQ, recently shown to have renewed efficacy after its withdrawal for treatment [42], or frequently used AL, could be combined with an antagonistic drug such as MQ or DP, either in combination therapy or as part of a rotation or multiple first-line treatment regime. The resulting effect would be opposing selective pressures on resistance alleles and thus a slower rate of resistance fixation in the population. The exploitation of such negative cross-resistance, previously used in agriculture [43], has also been proposed to be used in insecticide treatment of bed nets to tackle resistance in mosquitoes [44] or as antibiotic resistance management [13]. Although this approach is not evolution-proof—pathogens may eventually evolve resistance against both drugs contemporaneously if general resistance mechanisms evolve [14]—it has the potential to slow the spread of resistance significantly. Of note, however, the selection observed in this study was transient in nature: the effect of IPTp treatment on selection for wild-type parasites had already disappeared 1 month after delivery. Thus, the effect of negative cross-resistance may only be evident when the mother has sufficient drug plasma levels after IPTp. Therefore, the role of selection from intermittent interventions such as IPTp on the overall parasite population may be limited. In all of the above, it is important to consider the limitation of relying on polymorphisms as resistance markers. Parasite isolates with pfcrt and pfmdr1 polymorphism can still display diverse phenotypes in terms of resistance [45]. Ultimately, in vivo susceptibilities should be linked to these polymorphisms to support their role in resistance as well as the increase in MQ susceptibility of 86Y.
We did not observe an association between quintuple pfdhfr/pfdhps resistance carriage and adverse pregnancy outcomes as one might expect in the face of competitive facilitation shown in a previous study [2], although not confirmed in a recent meta-analysis [27]. However, although our study has the advantage of 2 perfectly randomized treatment groups, which was a major limitation of the study by Harrington et al [2], the conditions in our study were not suitable to test the hypothesis of competitive facilitation appropriately. For the facilitative effect to occur, sufficient intrahost competition and, thus, high levels of MOI must be observed, possibly in the presence of the pfdhps polymorphism 581G. In addition, an untreated control group would allow for direct comparison between treated and untreated women. These conditions are rare, and therefore the true significance of the facilitative effect is difficult to verify in different settings [24–26, 28]. In our study, the prevalence of the quintuple folate genotype was very high in Mozambique and Kenya, but here the pfdhps polymorphism 581G was absent. Multiplicity of infection was relatively low in comparison to the site where competitive facilitation was observed, with mean MOI between our different sites being 2.4 (Table 1) compared with 7.4 in the study by Harrington et al [2]. In the clinical trial, pregnancy outcomes were similar between the IPTp-MQ and IPTp-SP, although MQ recipients had less clinical malaria than SP recipients [29].
CONCLUSIONS
The future of IPTp-SP has been a topic of heated debate in recent years. The potential development of resistance and loss of efficacy, although not well documented so far, have led to discussions of whether IPTp-SP should be endorsed and under which conditions. In our study, we have shown a strong negative selective pressure of IPTp-MQ on pfmdr1 86Y, a polymorphism associated with CQ resistance and MQ susceptibility, but no detectable selective pressure from IPTp-SP. Although MQ is an unlikely IPTp candidate [29, 30], other drugs such as DP and AL might have the same effect [10–12]. The finding that IPTp-drugs could lead to strong observable negative cross-resistance with other drugs, even during the short time frame of a pregnancy, opens an area for drug discovery that could potentially limit the spread of antimalarial resistance if designed with such evolutionary dynamics in mind [6]. With IPTp-DP as a suitable alternative for IPTp-SP [46, 47] and AL a common antimalarial drug for clinical treatment, the combination could indeed have great potential to slow the spread of resistance alleles.
Supplementary Data
Supplementary materials are available at The Journal of Infectious Diseases online. Consisting of data provided by the authors to benefit the reader, the posted materials are not copyedited and are the sole responsibility of the authors, so questions or comments should be addressed to the corresponding author.
Note
Acknowledgments. We are grateful to all of the women who participated in the study. We thank the staff at the different study sites for their contribution to the collection and analysis of samples.
Financial support. This work was funded by the European Developing Countries Clinical Trials Partnership (IP.2007.31080.002); the Malaria in Pregnancy Consortium; Marie Curie Incoming Fellowship (623703; to S. H.); Branco Weiss Fellowship – Society in Science (to S. H.); Departament d'Universitats i Recerca de la Generalitat de Catalunya (2017SGR664 to A. M.); and the Overseas Postdoctoral Fellowship by the Department of Science & Technology, Government of India (SB/OS/PDF043/201516; to H. G.). The MiP Consortium is funded through a grant from the Bill & Melinda Gates Foundation to the Liverpool School of Tropical Medicine. ISGlobal is a member of the CERCA Programme, Generalitat de Catalunya. The Centro de Investigacao em Saude da Manhica receives core support from the Spanish Agency for International Cooperation and Development.
Potential conflicts of interest. All authors: No reported conflicts of interest. All authors have submitted the ICMJE Form for Disclosure of Potential Conflicts of Interest.
Presented in part: Annual Meeting of the International Society of Evolution, Medicine and Public Health, August 2018, Park City, Utah; Yearly Society in Science Symposium, November 2017, Zurich, Switzerland; CNRS - Jacques Monod Conference, November 2017, Roscoff, France.
References
Author notes
C. M. and A. M. are co-senior authors.