-
PDF
- Split View
-
Views
-
Cite
Cite
Pablo Tebas, Kimberly A Kraynyak, Ami Patel, Joel N Maslow, Matthew P Morrow, Albert J Sylvester, Dawson Knoblock, Elisabeth Gillespie, Dinah Amante, Trina Racine, Trevor McMullan, Moonsup Jeong, Christine C Roberts, Young K Park, Jean Boyer, Kate E Broderick, Gary P Kobinger, Mark Bagarazzi, David B Weiner, Niranjan Y Sardesai, Scott M White, Intradermal SynCon® Ebola GP DNA Vaccine Is Temperature Stable and Safely Demonstrates Cellular and Humoral Immunogenicity Advantages in Healthy Volunteers, The Journal of Infectious Diseases, Volume 220, Issue 3, 1 August 2019, Pages 400–410, https://doi.org/10.1093/infdis/jiz132
- Share Icon Share
Abstract
Nonlive vaccine approaches that are simple to deliver and stable at room temperature or 2–8°C could be advantageous in controlling future Ebola virus (EBOV) outbreaks. Using an immunopotent DNA vaccine that generates protection from lethal EBOV challenge in small animals and nonhuman primates, we performed a clinical study to evaluate both intramuscular (IM) and novel intradermal (ID) DNA delivery.
Two DNA vaccine candidates (INO-4201 and INO-4202) targeting the EBOV glycoprotein (GP) were evaluated for safety, tolerability, and immunogenicity in a phase 1 clinical trial. The candidates were evaluated alone, together, or in combination with plasmid-encoded human cytokine interleukin-12 followed by in vivo electroporation using either the CELLECTRA® IM or ID delivery devices.
The safety profile of all 5 regimens was shown to be benign, with the ID route being better tolerated. Antibodies to EBOV GP were generated by all 5 regimens with the fastest and steepest rise observed in the ID group. Cellular immune responses were generated with every regimen.
ID delivery of INO-4201 was well tolerated and resulted in 100% seroreactivity after 2 doses and elicited interferon-γ T-cell responses in over 70% of subjects, providing a new approach for EBOV prevention in diverse populations.
Clinical Trials Registration. NCT02464670.
Ebola virus disease (EVD) is a severe hemorrhagic fever virus infection caused by the filovirus Ebolavirus [1]. There are currently no licensed vaccines for EVD prevention and the absence of approved therapeutics to treat EVD is a major obstacle for outbreak control. A safe and immunogenic vaccine that is also temperature stable is highly desirable for field delivery and to help prevent future EVD outbreaks.
Significant resources have been deployed to rapidly advance experimental EVD vaccine candidates to the clinic. Vaccine candidates encoding 1 or more Ebolavirus and other filovirus glycoprotein (GP) immunogens have been evaluated in phase 1 trials, including a human adenovirus serotype 5 (Ad5-EBOV) [2, 3], adenovirus type 26 prime with a modified vaccinia virus Ankara (Ad26-MVA) boost [4], and DNA vaccines delivered by Biojector [5, 6].
Three vaccine candidates have advanced further into clinical development: (1) a chimpanzee adenovirus 3 (ChAd3-EBO-Z) expressing wild-type EBOV GP from the Zaire Mayinga strain [7, 8]; (2) a MVA Ankara, (MVA-BN-Filo) expressing Zaire EBOV, Sudan EBOV GPs, and other filovirus proteins, which has been used as a booster after the administration of the cAd3-EBO-Z vaccine [9]; and (3) an attenuated, replication-competent, recombinant vesicular stomatitis virus (rVSV-ZEBOV) [10–13], expressing GPs of the Zaire Kikwit strain.
rVSV-ZEBOV is the most extensively evaluated vaccine candidate in that it was studied in a phase 3 trial in West Africa using a ring vaccination cluster randomized design [11, 12, 14]. There were no cases of EVD in the immediate vaccination group clusters from day 10 onwards, whereas in delayed vaccination groups, there were 16 cases of EVD from 7 clusters, showing a high protective efficacy, which represents a major advance in vaccine development. Some issues remain for a next-generation vaccine as rVSV-ZEBOV has some limitations, including safety concerns in specific immunosuppressed populations [10]. In addition, a relative lack of temperature stability of investigational viral vectored EBOV vaccines leads to significant challenges for their deployment in the EVD outbreak regions in terms of shipping (requires −80°C transport) and storage (eg, stable for <24 hours at 25°C) [15]. Viral-vectored vaccines can be difficult to boost due to antiviral vector immunity, which a recent publication has shown in more than one-third rVSV-ZEBOV vaccinees [16], and is relevant for health care workers who are redeployed with each additional outbreak. A new generation of synthetic optimized DNA (SynCon) vaccines against various viral targets, including human papilloma virus [17], chikungunya virus [18], human immunodeficiency virus [19], and Zika virus [20], appear immunopotent in clinical trials, exhibit excellent safety profiles, and are not hampered by antivector immunity. Unlike the vectored approaches, SynCon DNA vaccines are temperature stable (room temperature and 2–8°C) over extended time periods, enabling easier shipping and storage, and maintain stability through multiple freeze-thaw cycles. The potency of this SynCon DNA vaccine platform has been optimized through a combination of genetic modifications, genetic adjuvants, and facilitated delivery by CELLECTRA electroporation (EP) [21–23] with demonstrated impact on clinical disease [17]. Importantly, this platform can be rapidly deployed, as demonstrated for 2 phase I studies of the GLS_5700 Zika vaccine, including a study in an endemic region [20]. DNA vaccine candidates against EBOV have been shown to be protective against lethal challenge in nonhuman primates after 3 intramuscular (IM) injections followed by EP [24, 25] and 100% protective after just 2 intradermal (ID) injections followed by EP with the CELLECTRA device [25].
In this phase 1 clinical study (ClinicalTrials.gov NCT02464670), we evaluated the safety and immunogenicity of SynCon EBOV DNA vaccines INO-4201, INO-4202, and the combination, INO-4212, in healthy adults, alone or in combination with cytokine genetic adjuvant INO-9012, by IM or ID administration routes, delivered by CELLECTRA devices.
METHODS
Study Population
Healthy adult volunteers, 18–50 years of age, were enrolled between 21 May 2015 and 10 November 2016. Additional details are provided in the Supplementary Materials. The study was reviewed and approved by the institutional review board or local ethics committees at each participating center and all participants provided written informed consent.
Study Design and Vaccines
INO-4201 is a SynCon DNA construct, designed as a consensus of 14 different Ebolavirus GP sequences from outbreaks that occurred in Zaire from 1976 to 2008 (Supplementary Figure 1). INO-4202 encodes a Zaire Makona Ebolavirus GP 100% amino acid matched to a West African clinical isolate from the 2014 EVD outbreak. Vaccine stability was established for 36 months at 4°C based on nucleic acid concentration, preservation of circular and supercoiled forms, pH, and sterility. INO-4212 is an equal-part formulation of INO-4201 and INO-4202. INO-9012 encodes human interleukin-12 (IL-12). DNA sequences were codon and RNA optimized to enhance expression in humans.
Vaccine doses and dose schedules are shown in Table 1; each cohort consisted of 15 participants. Vaccines were administered at 0, 4, and 12 weeks via the IM route (1 mL volume) or ID route (0.1 mL volume) followed immediately by EP with CELLECTRA-5P (IM) or CELLECTRA-3P (ID). CELLECTRA delivers 52 ms controlled electrical pulses (max voltage 200 V) that increase cell membrane permeability and intracellular transfer of the plasmid DNA to the nucleus, resulting in enhanced transfection and immunogenicity [19, 26]. Additional details are provided in the Supplementary Materials.
EBOV-001 Cohort Summary of Vaccination Regimen, Route, Dose, and Plasmids Included in the Vaccine
Cohort . | Vaccine . | Plasmids Included in Vaccine . | Immunization Schedule . | Route . | Total Dose, mg . |
---|---|---|---|---|---|
1 | INO-4201 | INO-4201 | 0–4–12 weeks | IM | 2 |
2 | INO-4202 | INO-4202 | 0–4–12 weeks | IM | 2 |
3 | INO-4201 | INO-4201 | 0–4–12 weeks | ID | 2 |
4 | INO-4212 | INO-4201 and INO-4202 | 0–4–12 weeks | IM | 4 |
5 | INO-4212 + INO-9012 | INO-4201, INO-4202, and INO-9012 | 0–4–12 weeks | IM | 4 + 1 |
Cohort . | Vaccine . | Plasmids Included in Vaccine . | Immunization Schedule . | Route . | Total Dose, mg . |
---|---|---|---|---|---|
1 | INO-4201 | INO-4201 | 0–4–12 weeks | IM | 2 |
2 | INO-4202 | INO-4202 | 0–4–12 weeks | IM | 2 |
3 | INO-4201 | INO-4201 | 0–4–12 weeks | ID | 2 |
4 | INO-4212 | INO-4201 and INO-4202 | 0–4–12 weeks | IM | 4 |
5 | INO-4212 + INO-9012 | INO-4201, INO-4202, and INO-9012 | 0–4–12 weeks | IM | 4 + 1 |
Abbreviations: ID, intradermal; IM, intramuscular.
EBOV-001 Cohort Summary of Vaccination Regimen, Route, Dose, and Plasmids Included in the Vaccine
Cohort . | Vaccine . | Plasmids Included in Vaccine . | Immunization Schedule . | Route . | Total Dose, mg . |
---|---|---|---|---|---|
1 | INO-4201 | INO-4201 | 0–4–12 weeks | IM | 2 |
2 | INO-4202 | INO-4202 | 0–4–12 weeks | IM | 2 |
3 | INO-4201 | INO-4201 | 0–4–12 weeks | ID | 2 |
4 | INO-4212 | INO-4201 and INO-4202 | 0–4–12 weeks | IM | 4 |
5 | INO-4212 + INO-9012 | INO-4201, INO-4202, and INO-9012 | 0–4–12 weeks | IM | 4 + 1 |
Cohort . | Vaccine . | Plasmids Included in Vaccine . | Immunization Schedule . | Route . | Total Dose, mg . |
---|---|---|---|---|---|
1 | INO-4201 | INO-4201 | 0–4–12 weeks | IM | 2 |
2 | INO-4202 | INO-4202 | 0–4–12 weeks | IM | 2 |
3 | INO-4201 | INO-4201 | 0–4–12 weeks | ID | 2 |
4 | INO-4212 | INO-4201 and INO-4202 | 0–4–12 weeks | IM | 4 |
5 | INO-4212 + INO-9012 | INO-4201, INO-4202, and INO-9012 | 0–4–12 weeks | IM | 4 + 1 |
Abbreviations: ID, intradermal; IM, intramuscular.
Safety Evaluations
Subjects were sequentially enrolled and a safety review committee provided oversight, reviewed laboratory and adverse event data collected through 2 weeks postvaccination from the first 9 subjects in each cohort prior to opening enrollment of the next cohort, as outlined in the Supplementary Methods. Participants were monitored for adverse events utilizing the Toxicity Grading Scale for Healthy Adult and Adolescent Volunteers Enrolled in Preventive Vaccine Clinical Trials for the study duration [27]. Pain was assessed by visual analog scale (VAS) immediately after EP and at 5 and 10 minutes post-EP [28]. Laboratory safety assessments including hematology, electrolytes, renal and liver function, and creatine phosphokinase (CPK) were obtained at screening weeks 2 and 14.
Binding Enzyme-Linked Immunosorbent Assays
A standard binding enzyme-linked immunosorbent assay (ELISA) was developed at Inovio to assay total IgG antibodies specific for Zaire EBOV GP (Sino Biologicals, 40442-V08B1), 99% identical to INO-4201 and 97% identical to INO-4202. It was optimized using samples from vaccinated nonhuman primates as positive controls. Postvaccination end point titers were reported as the dilution of sera at which the optical density (OD) was 2.5 SD above baseline and above the OD cutoff of 0.2. Seroreactivity was defined as an end point titer greater than 1.
The commercially available EBOV-GP ELISA assay (Alpha Diagnostic, TX; ADI ELISA), was performed according to manufacturer’s instructions (quantification method C).
Interferon-γ ELISpot Assay
ELISpot assay was performed as previously described [29] except using full-length consensus GP (100% identical to INO-4201 and 97.3% identical to INO-4202) 15-mer peptides. The responder threshold was generated using baseline values from all subjects, excluding outliers (Supplementary Figure 2). Eight subjects were outliers for at least 1 peptide pool or total EBOV (sum of the 4 peptide pools) and excluded from subsequent analyses. Please see Supplementary Methods for additional information.
Neutralization Assay
After inactivating complement, serum samples were incubated with 200 plaque-forming units/well of Zaire EBOV (Makona C05, 97% identical to INO-4201 and 99% identical to INO-4202) for 45 minutes at room temperature before adding to Vero E6 cells for 60 minutes at 37°C. The virus/serum mixture was replaced with cell culture media and plates were incubated for 13–14 days. The neutralizing antibody titer is reported as the reciprocal of the highest dilution with no cytopathic effect (ie, the dilution that provided 100% neutralization). Please see Supplementary Methods for additional information.
Intracellular Cytokine Staining
Intracellular cytokine staining was performed as previously described [29]. Prepared cells were acquired using an LSR Fortessa flow cytometer equipped with BD FACSDiva software (BD Biosciences). Data was analyzed using FlowJo software version 10 (Tree Star, Ashland, OR).
Statistics
All subjects who were vaccinated at least once were included in safety analyses, where they were grouped according to treatment regimen. Safety analyses included tabulating the frequencies of adverse events, injection site reactions, changes in VAS scores, and changes in laboratory parameters. Demographic analyses were conducted on the modified intent-to-treat (mITT) set, where vaccinated subjects were assigned according to their enrolled groups. Subjects were included in immunogenicity analyses if they were vaccinated at least once, had a blood sample available, and were members of the mITT set. As no formal hypothesis testing was planned, descriptive statistics are presented from the data collected with no correction for multiple comparisons. Please see Supplementary Methods for additional statistical information.
RESULTS
Study Population Demographics
A total of 75 participants were enrolled, aged between 18 and 50 years. Participant demographic characteristics are summarized in Supplementary Table 1. The median age was 35 years (range 18–50). Participants were 67% female, 12% Latino, and 52% white.
Disposition, Safety, and Tolerability
Of the 75 participants, 68 (91%) completed all 3 vaccinations and 62 (83%) completed all follow-up study visits (Supplementary Table 2). Of the 13 participants who did not complete all study visits, 3 (4%) withdrew consent and 10 (13%) were lost to follow-up. No subjects discontinued the study due to an adverse event. No treatment-related serious adverse events were reported. The most frequent solicited local adverse events by subject were injection site pain (67%), redness (39%), itching (31%), and swelling (19%). Grade 1 or 2 injection site reactions were experienced by 75% (n = 56) and 25% (n = 19) of subjects, respectively (zero grade 3 or 4) (Supplementary Table 3) with the majority of events lasting 1 day or less. There was no observed difference in the occurrence of injection site reactions with the addition of INO-9012. Among injection site reactions, subjects in IM groups were significantly more likely to report pain (73%) compared to those in the ID group (40%, P = .0159). Similarly, VAS scores obtained immediately following dosing and then 5 and 10 minutes later were higher in the IM groups (Supplementary Figure 3A–3C). Specifically, mean VAS scores were significantly lower in the ID group immediately following dosing (weeks 0, 4, and 12) and 5 minutes later (weeks 0 and 4). Also, mean VAS scores were relatively consistent from the first to the second and third doses.
The most frequent systemic adverse events included myalgia (33%), fatigue (27%), headache (25%), and malaise (12%) (Supplementary Table 4). These systemic adverse events were more frequently observed in IM cohorts than in the ID cohort, and more frequently in the IM cohort with INO-9012 than the other IM cohorts. There were no febrile reactions. There were no abnormal laboratory values or abnormal laboratory value trends over time of clinical concern during the study (Supplementary Table 5 and Table 6). One subject in cohort 3 (ID dosing) had a single CPK value of 5498 U/L at week 14, which by patient history was associated with recent vigorous physical activity. No other adverse event was associated with this elevated CPK value.
Humoral Immune Responses
Seroreactivity to EBOV GP
Antibodies to Zaire EBOV GP were measured by an ELISA developed at Inovio and rates of seroreactivity through week 14 are shown in Figure 1A. Little seroreactivity was observed following a single immunization across any of the 4 IM cohorts. Seroreactivity was observed in 38% (5/13) of subjects following a single ID immunization of INO-4201 (cohort 3), compared to 0% (0/15) following a single IM administration of the same vaccine (cohort 1) (P = .0102). Seroreactivity rates were 53% (8/15) in cohort 1 (IM INO-4201) after 2 doses and 87% (13/15) after 3 doses (Figure 1A). By comparison, the same vaccine given ID (cohort 3) resulted in 100% seroreactivity (13/13) after 2 doses (P = .0044) and remained 100% seropositive following 3 doses (Figure 1A). The addition of plasmid IL-12 enhanced seroreactivity of INO-4212 after 2 doses to 92% (12/13) in cohort 5 compared to 57% (8/14) in cohort 4, although this was not significant (P = .0529; Figure 1A).
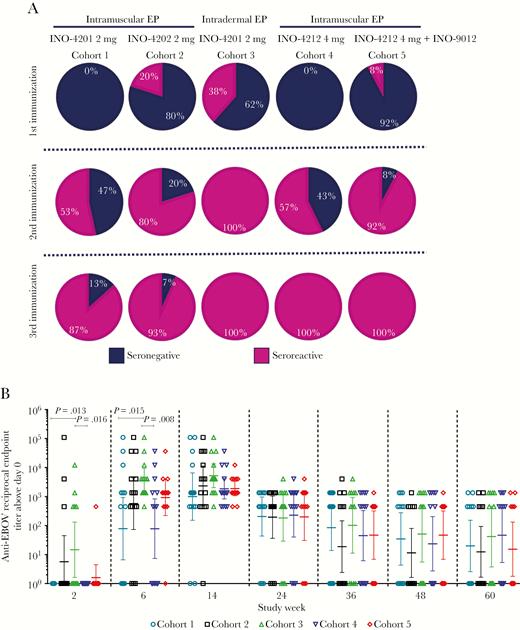
Seroreactivity and total IgG antibody titers against EBOV GP. A, Seroreactive percentages following 1, 2, and 3 immunizations (up to n = 15 subjects per cohort). B, Anti-EBOV GP antibody end point titers. Open symbols represent the reciprocal end point titer of individual subjects 2 weeks following each immunization. Horizontal lines are at the GMT with error bars representing the 95% confidence intervals, from Wilcoxon rank-sum test. Abbreviations: EBOV, Ebola virus; EP, electroporation; GMT, geometric mean titer; GP, glycoprotein.
Glycoprotein-Specific End Point Titers
ID delivery of INO-4201 (cohort 3) resulted in the highest geometric mean titer (GMT) of GP-specific antibodies: after dose 1 GMT was 15 (range 1–12 150), after dose 2 GMT was 3722 (range 450–109 350), and after dose 3 GMT was 5219 (range 450–109 350) (Figure 1B and Table 2). These titers were significantly higher than after 1 and 2 IM doses (P = .013 and P = .015, respectively), demonstrating that ID delivery of the same dose of DNA afforded more rapid seroreactivity and total seroreactivity, and generated significantly higher antibody titers (Figure 1A and 1B) than IM delivery. The addition of INO-9012, IL-12 DNA (cohort 5), also had a positive impact on antibody titers with a GMT of 918 after 2 doses compared to a GMT of 78 in the similar cohort without IL-12 (cohort 4), although this difference was not significant (Figure 1B and Table 2). Responses in all cohorts remained high 12 months following the final dose (week 60) and were not significantly different from one another.
Cohort . | GMT (range) . | ||||||
---|---|---|---|---|---|---|---|
Week 2 . | Week 6 . | Week 14 . | Week 24 . | Week 36 . | Week 48 . | Week 60 . | |
1 | 1 (1–1) | 78 (1–109 350) | 998 (1–109 350) | 206 (1–1350) | 85 (1–1350) | 35 (1–1350) | 20 (1–1350) |
2 | 6 (1–109 350) | 574 (1–109 350) | 2328 (1–109 350) | 195 (1–1350) | 19 (1–4050) | 11 (1–1350) | 12 (1–1350) |
3 | 15 (1–12 150) | 3722 (450–109 350) | 5219 (450–109 350) | 182 (1–4050) | 102 (1–4050) | 51 (1–1350) | 42 (1–1350) |
4 | 1 (1–1) | 78 (1–36 450) | 1848 (450–36 450) | 228 (1–4050) | 45 (1–1350) | 24 (1–1350) | 46 (1–1350) |
5 | 2 (1–450) | 918 (1–36 450) | 1893 (450–12 150) | 199 (1–4050) | 47 (1–1350) | 47 (1–1350) | 15 (1–1350) |
Cohort . | GMT (range) . | ||||||
---|---|---|---|---|---|---|---|
Week 2 . | Week 6 . | Week 14 . | Week 24 . | Week 36 . | Week 48 . | Week 60 . | |
1 | 1 (1–1) | 78 (1–109 350) | 998 (1–109 350) | 206 (1–1350) | 85 (1–1350) | 35 (1–1350) | 20 (1–1350) |
2 | 6 (1–109 350) | 574 (1–109 350) | 2328 (1–109 350) | 195 (1–1350) | 19 (1–4050) | 11 (1–1350) | 12 (1–1350) |
3 | 15 (1–12 150) | 3722 (450–109 350) | 5219 (450–109 350) | 182 (1–4050) | 102 (1–4050) | 51 (1–1350) | 42 (1–1350) |
4 | 1 (1–1) | 78 (1–36 450) | 1848 (450–36 450) | 228 (1–4050) | 45 (1–1350) | 24 (1–1350) | 46 (1–1350) |
5 | 2 (1–450) | 918 (1–36 450) | 1893 (450–12 150) | 199 (1–4050) | 47 (1–1350) | 47 (1–1350) | 15 (1–1350) |
Abbreviations: EBOV, Ebola virus; EU, ELISA unit; GMT, geometric mean titer; GP, glycoprotein.
Cohort . | GMT (range) . | ||||||
---|---|---|---|---|---|---|---|
Week 2 . | Week 6 . | Week 14 . | Week 24 . | Week 36 . | Week 48 . | Week 60 . | |
1 | 1 (1–1) | 78 (1–109 350) | 998 (1–109 350) | 206 (1–1350) | 85 (1–1350) | 35 (1–1350) | 20 (1–1350) |
2 | 6 (1–109 350) | 574 (1–109 350) | 2328 (1–109 350) | 195 (1–1350) | 19 (1–4050) | 11 (1–1350) | 12 (1–1350) |
3 | 15 (1–12 150) | 3722 (450–109 350) | 5219 (450–109 350) | 182 (1–4050) | 102 (1–4050) | 51 (1–1350) | 42 (1–1350) |
4 | 1 (1–1) | 78 (1–36 450) | 1848 (450–36 450) | 228 (1–4050) | 45 (1–1350) | 24 (1–1350) | 46 (1–1350) |
5 | 2 (1–450) | 918 (1–36 450) | 1893 (450–12 150) | 199 (1–4050) | 47 (1–1350) | 47 (1–1350) | 15 (1–1350) |
Cohort . | GMT (range) . | ||||||
---|---|---|---|---|---|---|---|
Week 2 . | Week 6 . | Week 14 . | Week 24 . | Week 36 . | Week 48 . | Week 60 . | |
1 | 1 (1–1) | 78 (1–109 350) | 998 (1–109 350) | 206 (1–1350) | 85 (1–1350) | 35 (1–1350) | 20 (1–1350) |
2 | 6 (1–109 350) | 574 (1–109 350) | 2328 (1–109 350) | 195 (1–1350) | 19 (1–4050) | 11 (1–1350) | 12 (1–1350) |
3 | 15 (1–12 150) | 3722 (450–109 350) | 5219 (450–109 350) | 182 (1–4050) | 102 (1–4050) | 51 (1–1350) | 42 (1–1350) |
4 | 1 (1–1) | 78 (1–36 450) | 1848 (450–36 450) | 228 (1–4050) | 45 (1–1350) | 24 (1–1350) | 46 (1–1350) |
5 | 2 (1–450) | 918 (1–36 450) | 1893 (450–12 150) | 199 (1–4050) | 47 (1–1350) | 47 (1–1350) | 15 (1–1350) |
Abbreviations: EBOV, Ebola virus; EU, ELISA unit; GMT, geometric mean titer; GP, glycoprotein.
ADI ELISA Quantification
A commercially available ELISA kit that measures IgG specific for EBOV GP IgG was also run in addition to the Inovio ELISA in order to quantify IgG levels and serve as a basis for comparison with other vaccine platforms. There was a strong Spearman correlation (r = 0.70, P < .0001) between the 2 ELISA assays on a per patient basis (Supplementary Figure 4A). Cohorts 3 and 5 were the only 2 treatment arms in which significantly higher ELISA unit (EU)/mL was measured after 1 dose (study week 2) and at each study week, compared with the respective baseline samples (Figure 2). The GP-specific binding titers observed at baseline were unexpected, but similar to those reported by other groups [13], and ranged from 1 to 10 000. Due to the variability in background levels observed between cohorts, values at study week 0 were subtracted from each subsequent study week on an individual subject basis to allow for comparisons between cohorts (Table 3). The geometric mean fold changes from baseline for each cohort over time were also calculated (Supplementary Figure 4B and 4C). Similar GMTs were measured in the serum from cohorts 3 and 5 after the second dose, which were significantly greater than the GMTs in all the other cohorts at weeks 6 and 14 (data not shown).
Baseline Subtracted GMT of EBOV GP Specific Antibodies (EU/mL) Across Cohorts and Timepoints
Cohort . | GMT, EU/mL (range) . | ||||
---|---|---|---|---|---|
Week 2 . | Week 6 . | Week 14 . | Week 24 . | Week 36 . | |
1 | 6 (1–135) | 54 (1–780) | 75 (1–1511) | 60 (1–622) | 14 (1–237) |
2 | 4 (1–289) | 26 (1–515) | 93 (1–793) | 46 (1–436) | 13 (1–4064) |
3 | 10 (1–72) | 476 (57–2831) | 1227 (170–8531) | 200 (21–871) | 45 (1–455) |
4 | 6 (1–37) | 116 (7–2290) | 396 (22–11 700) | 82 (1–1343) | 23 (1–603) |
5 | 15 (1–160) | 558 (61–11 142) | 1130 (190–10 446) | 233 (1–1147) | 38 (1–498) |
Cohort . | GMT, EU/mL (range) . | ||||
---|---|---|---|---|---|
Week 2 . | Week 6 . | Week 14 . | Week 24 . | Week 36 . | |
1 | 6 (1–135) | 54 (1–780) | 75 (1–1511) | 60 (1–622) | 14 (1–237) |
2 | 4 (1–289) | 26 (1–515) | 93 (1–793) | 46 (1–436) | 13 (1–4064) |
3 | 10 (1–72) | 476 (57–2831) | 1227 (170–8531) | 200 (21–871) | 45 (1–455) |
4 | 6 (1–37) | 116 (7–2290) | 396 (22–11 700) | 82 (1–1343) | 23 (1–603) |
5 | 15 (1–160) | 558 (61–11 142) | 1130 (190–10 446) | 233 (1–1147) | 38 (1–498) |
Abbreviations: EBOV, Ebola virus; EU, ELISA unit; GMT, geometric mean titer; GP, glycoprotein.
Baseline Subtracted GMT of EBOV GP Specific Antibodies (EU/mL) Across Cohorts and Timepoints
Cohort . | GMT, EU/mL (range) . | ||||
---|---|---|---|---|---|
Week 2 . | Week 6 . | Week 14 . | Week 24 . | Week 36 . | |
1 | 6 (1–135) | 54 (1–780) | 75 (1–1511) | 60 (1–622) | 14 (1–237) |
2 | 4 (1–289) | 26 (1–515) | 93 (1–793) | 46 (1–436) | 13 (1–4064) |
3 | 10 (1–72) | 476 (57–2831) | 1227 (170–8531) | 200 (21–871) | 45 (1–455) |
4 | 6 (1–37) | 116 (7–2290) | 396 (22–11 700) | 82 (1–1343) | 23 (1–603) |
5 | 15 (1–160) | 558 (61–11 142) | 1130 (190–10 446) | 233 (1–1147) | 38 (1–498) |
Cohort . | GMT, EU/mL (range) . | ||||
---|---|---|---|---|---|
Week 2 . | Week 6 . | Week 14 . | Week 24 . | Week 36 . | |
1 | 6 (1–135) | 54 (1–780) | 75 (1–1511) | 60 (1–622) | 14 (1–237) |
2 | 4 (1–289) | 26 (1–515) | 93 (1–793) | 46 (1–436) | 13 (1–4064) |
3 | 10 (1–72) | 476 (57–2831) | 1227 (170–8531) | 200 (21–871) | 45 (1–455) |
4 | 6 (1–37) | 116 (7–2290) | 396 (22–11 700) | 82 (1–1343) | 23 (1–603) |
5 | 15 (1–160) | 558 (61–11 142) | 1130 (190–10 446) | 233 (1–1147) | 38 (1–498) |
Abbreviations: EBOV, Ebola virus; EU, ELISA unit; GMT, geometric mean titer; GP, glycoprotein.
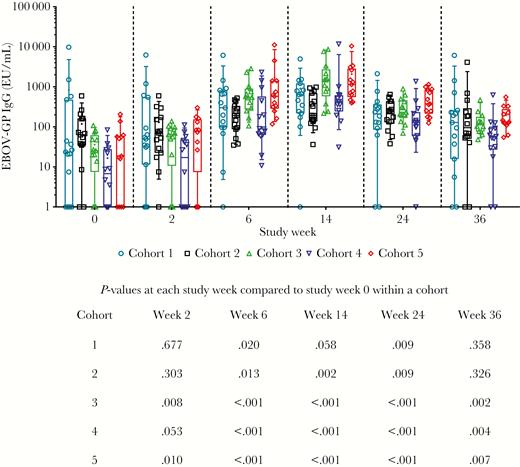
EBOV GP Specific Antibodies measured by ADI ELISA. Open symbols represent individual subjects, the box extends from the 25th to 75th percentile, line inside the box is at the median, and whiskers represent the 10th–90th percentile. The mean is denoted by +. Below the graph is shown P values for each cohort at each time point compared to their own baseline, from Wilcoxon signed rank test. Abbreviations: ADI ELISA, Alpha Diagnostic enzyme-linked immunosorbent assay; EBOV, Ebola virus; EU, ELISA unit; GMT, geometric mean titer; GP, glycoprotein.
Neutralizing Antibody Titers
We assessed the functionality of the antibodies using a live virus neutralization assay without a reporter gene, therefore a result was only scored positive if 100% prevention of cytopathic effects (neutralization) was observed. The detected neutralization titers were low and similar between cohorts (Supplementary Figure 5A). Responses ranged from 27% (4/15) to 54% (7/13) of participants having 100% neutralization in any specimen (Supplementary Figure 5B). At study week 14, levels of serum neutralizing antibody positively correlated with levels of binding antibody as measured by ADI ELISA, (r = 0.3200, P = .007; Supplementary Figure 5C).
Cellular Immune Responses
Interferon-γ ELISpot
An average T-cell responder rate of 74.6% was observed across all cohorts, ranging from 53.3% in cohort 1 to 93.3% in cohort 2 (Table 4). Responses were observed to all regions of the GP antigen, with the largest number of subjects (72.2%) reacting to pool 1 (Table 4), corresponding to the GP1 region. ID delivery of INO-4201 showed a tendency toward a higher frequency of responders compared to the same vaccine delivered via the IM route (cohort 3, 73.3% vs cohort 1, 53.3%; Table 4). Overall, the magnitude of the interferon-γ (IFN-γ) responses was not significantly different across cohorts (Figure 3). Following 2 immunizations, significant increases from study week 0 were observed in cohort 3 (Figure 3). Importantly, memory responses were observed with levels significantly above baseline present at study week 24 and 36, that is 12 and 24 weeks following the final immunization, respectively (Figure 3).
. | Cohort 1 IM . | Cohort 2 IM . | Cohort 3 ID . | Cohort 4 IM . | Cohort 5 IM + IL-12 . | |||||
---|---|---|---|---|---|---|---|---|---|---|
Pool . | Response, n (%) . | Mean SFU . | Response, n (%) . | Mean SFU . | Response, n (%) . | Mean SFU . | Response, n (%) . | Mean SFU . | Response, n (%) . | Mean SFU . |
1 | 7/13 (53.8) | 69.0 | 13/14 (92.9) | 79.1 | 10/15 (66.7) | 30.9 | 13/15 (86.7) | 84.8 | 9/15 (60.0) | 56.9 |
2 | 7/15 (46.7) | 62.1 | 10/14 (71.4) | 65.3 | 8/15 (53.3) | 40.5 | 6/14 (42.9) | 94.4 | 6/15 (40.0) | 117.6 |
3 | 0/14 (0.0) | NA | 5/15 (33.3) | 99.7 | 2/14 (14.3) | 291.3 | 0/14 (0.0) | NA | 1/15 (6.7) | 40.0 |
4 | 0/14 (0.0) | NA | 1/14 (7.1) | 103.3 | 2/14 (14.3) | 476.3 | 1/14 (7.1) | 83.3 | 2/15 (13.3) | 169.6 |
EBOV | 1/14 (7.1) | 296.7 | 3/15 (20.0) | 522.2 | 1/14 (7.1) | 1431.7 | 1/14 (7.1) | 158.3 | 2/15 (13.3) | 335.0 |
Any pool | 8/15 (53.3) | 14/15 (93.3) | 11/15 (73.3) | 13/15 (86.7) | 10/15 (66.7) |
. | Cohort 1 IM . | Cohort 2 IM . | Cohort 3 ID . | Cohort 4 IM . | Cohort 5 IM + IL-12 . | |||||
---|---|---|---|---|---|---|---|---|---|---|
Pool . | Response, n (%) . | Mean SFU . | Response, n (%) . | Mean SFU . | Response, n (%) . | Mean SFU . | Response, n (%) . | Mean SFU . | Response, n (%) . | Mean SFU . |
1 | 7/13 (53.8) | 69.0 | 13/14 (92.9) | 79.1 | 10/15 (66.7) | 30.9 | 13/15 (86.7) | 84.8 | 9/15 (60.0) | 56.9 |
2 | 7/15 (46.7) | 62.1 | 10/14 (71.4) | 65.3 | 8/15 (53.3) | 40.5 | 6/14 (42.9) | 94.4 | 6/15 (40.0) | 117.6 |
3 | 0/14 (0.0) | NA | 5/15 (33.3) | 99.7 | 2/14 (14.3) | 291.3 | 0/14 (0.0) | NA | 1/15 (6.7) | 40.0 |
4 | 0/14 (0.0) | NA | 1/14 (7.1) | 103.3 | 2/14 (14.3) | 476.3 | 1/14 (7.1) | 83.3 | 2/15 (13.3) | 169.6 |
EBOV | 1/14 (7.1) | 296.7 | 3/15 (20.0) | 522.2 | 1/14 (7.1) | 1431.7 | 1/14 (7.1) | 158.3 | 2/15 (13.3) | 335.0 |
Any pool | 8/15 (53.3) | 14/15 (93.3) | 11/15 (73.3) | 13/15 (86.7) | 10/15 (66.7) |
Abbreviations: EBOV, Ebola virus; ID, intradermal; IFN-γ, interferon-γ; IL-12, interleukin-12; IM, intramuscular; NA, not applicable; SFU, spot forming unit.
. | Cohort 1 IM . | Cohort 2 IM . | Cohort 3 ID . | Cohort 4 IM . | Cohort 5 IM + IL-12 . | |||||
---|---|---|---|---|---|---|---|---|---|---|
Pool . | Response, n (%) . | Mean SFU . | Response, n (%) . | Mean SFU . | Response, n (%) . | Mean SFU . | Response, n (%) . | Mean SFU . | Response, n (%) . | Mean SFU . |
1 | 7/13 (53.8) | 69.0 | 13/14 (92.9) | 79.1 | 10/15 (66.7) | 30.9 | 13/15 (86.7) | 84.8 | 9/15 (60.0) | 56.9 |
2 | 7/15 (46.7) | 62.1 | 10/14 (71.4) | 65.3 | 8/15 (53.3) | 40.5 | 6/14 (42.9) | 94.4 | 6/15 (40.0) | 117.6 |
3 | 0/14 (0.0) | NA | 5/15 (33.3) | 99.7 | 2/14 (14.3) | 291.3 | 0/14 (0.0) | NA | 1/15 (6.7) | 40.0 |
4 | 0/14 (0.0) | NA | 1/14 (7.1) | 103.3 | 2/14 (14.3) | 476.3 | 1/14 (7.1) | 83.3 | 2/15 (13.3) | 169.6 |
EBOV | 1/14 (7.1) | 296.7 | 3/15 (20.0) | 522.2 | 1/14 (7.1) | 1431.7 | 1/14 (7.1) | 158.3 | 2/15 (13.3) | 335.0 |
Any pool | 8/15 (53.3) | 14/15 (93.3) | 11/15 (73.3) | 13/15 (86.7) | 10/15 (66.7) |
. | Cohort 1 IM . | Cohort 2 IM . | Cohort 3 ID . | Cohort 4 IM . | Cohort 5 IM + IL-12 . | |||||
---|---|---|---|---|---|---|---|---|---|---|
Pool . | Response, n (%) . | Mean SFU . | Response, n (%) . | Mean SFU . | Response, n (%) . | Mean SFU . | Response, n (%) . | Mean SFU . | Response, n (%) . | Mean SFU . |
1 | 7/13 (53.8) | 69.0 | 13/14 (92.9) | 79.1 | 10/15 (66.7) | 30.9 | 13/15 (86.7) | 84.8 | 9/15 (60.0) | 56.9 |
2 | 7/15 (46.7) | 62.1 | 10/14 (71.4) | 65.3 | 8/15 (53.3) | 40.5 | 6/14 (42.9) | 94.4 | 6/15 (40.0) | 117.6 |
3 | 0/14 (0.0) | NA | 5/15 (33.3) | 99.7 | 2/14 (14.3) | 291.3 | 0/14 (0.0) | NA | 1/15 (6.7) | 40.0 |
4 | 0/14 (0.0) | NA | 1/14 (7.1) | 103.3 | 2/14 (14.3) | 476.3 | 1/14 (7.1) | 83.3 | 2/15 (13.3) | 169.6 |
EBOV | 1/14 (7.1) | 296.7 | 3/15 (20.0) | 522.2 | 1/14 (7.1) | 1431.7 | 1/14 (7.1) | 158.3 | 2/15 (13.3) | 335.0 |
Any pool | 8/15 (53.3) | 14/15 (93.3) | 11/15 (73.3) | 13/15 (86.7) | 10/15 (66.7) |
Abbreviations: EBOV, Ebola virus; ID, intradermal; IFN-γ, interferon-γ; IL-12, interleukin-12; IM, intramuscular; NA, not applicable; SFU, spot forming unit.
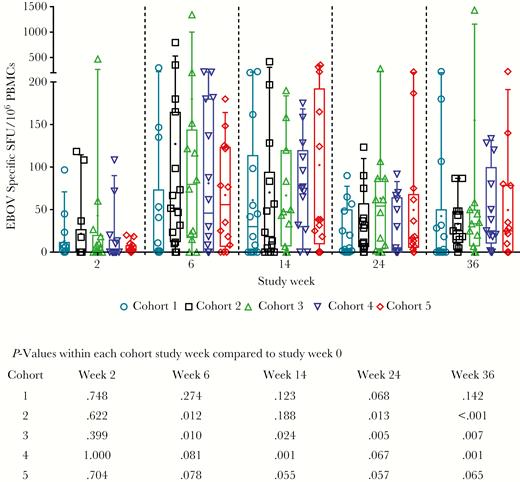
Cellular immune responses to EBOV as measured by IFN-γ ELISpot by cohort. Background subtracted EBOV SFU per million PBMCs are shown for individual subjects to total EBOV (the sum of glycoprotein peptide pools 1–4). For each cohort, open symbols represent individual subjects, box extends from the 25th to 75th percentile, line inside the box is at the median, and whiskers represent the 10th–90th percentile. The mean is denoted by +. Below the graph is shown P values for each cohort at each time point compared to their own baseline, from Wilcoxon signed rank test. Abbreviations: EBOV, Ebola virus; IFN-γ, interferon-gamma; PBMCs, peripheral blood mononuclear cells; SFU, specific spot forming units.
Intracellular Cytokine Staining
We further evaluated EBOV-specific cellular immune responses and induction of IFN-γ and tumor necrosis factor-alpha (TNF-α) via intracellular cytokine staining. Representative images are shown in Figure 4A pre- and postimmunization (week 14) for T cells producing TNF-α in response to stimulation with EBOV GP peptides. The upstream gating scheme is shown in Supplementary Figure 6. Antigen-specific T cells were induced in both the CD4+ and CD8+ compartments. Following ID delivery of INO-4201, significant increases in the frequency of EBOV GP-specific CD8+ and CD4+ T cells producing TNF-α (P = .001 and P = .008, respectively) were observed (Figure 4B). The frequency of CD8+ T cells producing IFN-γ and TNF-α significantly increased following ID immunization (P = .027); however, the frequency of CD8+ T cells producing IFN-γ alone did not reach significance (Figure 4B). No significant increases in TNF-α and/or IFN-γ–producing CD4+ or CD8+ T cells was observed with IM delivery of INO-4201 (Figure 4B). The higher percentage of TNF-α over IFN-γ is similar to observations in memory T cells from EVD survivors [30].
Responses to intracellular cytokine staining in subjects that received INO-4201 via the intradermal (cohort 3) or intramuscular (cohort 1) route. A, Representative images of CD4+ T cells (top) and CD8+ T cells (bottom) producing TNF-α in response to stimulation with EBOV peptides pre- and postimmunization. B, Frequencies of IFN-γ and/or TNF-α–producing CD8+ and CD4+ T-cell populations. For each timepoint, the box extends from the 25th to 75th percentile, the line inside the box is at the median, and whiskers represent the 10th–90th percentile. The mean is denoted by +. The memory phenotype of TNF-α secreting cells is shown in the bottom 2 graphs; the box extends from the 25th to 75th percentile, the line inside the box is drawn at the median, and whiskers extend from the minimum to maximum values. Abbreviations: CM, central memory; E, effector; EBOV, Ebola virus; EM, effector memory; GP, glycoprotein; IFN-γ, interferon-gamma; TNF-α, tumor necrosis factor-alpha.
The composition of CD8+ or CD4+ T cells producing TNF-α was also assessed for surface markers CCR7 and CD45RO to characterize effector (CCR7−CD45RO−), effector memory (CCR7−CD45RO+), and central memory (CCR7+CD45RO+) cells. In both ID and IM-vaccinated participants, CD8+ T cells producing TNF-α were predominantly of the effector memory type, whereas the majority of CD4+ T cells producing TNF-α were of the central memory phenotype (Figure 4B).
DISCUSSION
The vaccines studied in EBOV-001 were previously shown to generate robust immunity, elicit memory responses persisting more than 1 year, and provided 100% protection against a lethal EBOV challenge in nonhuman primates after 2 ID injections [25]. In the clinic, rates of local and systemic side effects observed in EBOV-001 were unremarkable and similar to those reported for other DNA vaccines [17, 19, 20, 29]. Delivery of the vaccines using the CELLECTRA EP devices was well tolerated, with improved tolerability observed in the group immunized via the ID route. The vaccines generated potent humoral and cellular immune responses by both delivery routes that were higher than what has previously been reported for DNA delivery of GP vaccines [5, 6]. High levels of binding antibodies were induced after only 2 doses following either ID EP of INO-4201 (GMT 3722) and/or after IM EP adjuvanted by INO-9012 (GMT 918), with 100% seroreactivity in the ID EP cohort. Importantly, responses after 2 doses were similar to those reported for rVSV-ZEBOV as measured by ADI ELISA [10, 13] and responses to these DNA vaccines persisted for more than 1 year in most subjects, similar to long-term memory observed in the nonhuman primates [25]. While the binding antibody titers were similar to those reported for the rVSV-ZEBOV vaccine, the number of subjects with 100% neutralization as measured in a live virus assay as well as the titers were lower compared to what has been observed with other EBOV vaccine candidates using pseudovirus assays [13, 30]. A limitation of our assay is the binary readout of yes/no 100% neutralization as opposed to 50% neutralization capability in a pseudovirus assay.
Additionally, T-cell responses were observed in 53.3%–93.3% of subjects in IM cohorts. The same vaccine delivered ID and IM resulted in 73.3% and 53.3% of subjects responding, respectively. The magnitudes of these responses were comparable to what has been previously reported for rVSV-ZEBOV [30].
An issue for field deployment and boosting for some viral vectored vaccines is the presence of naturally acquired or vaccine-induced antivector immunity. The impact of preexisting immunity was notable in human trials evaluating an adenovirus type 5 (Ad5) vaccine encoding a Makona GP [2, 3]; however, a ChAd3-EBO-Z vaccine is being evaluated as most human populations exhibit a lower frequency of preexisting antibodies to ChAd3 than to Ad5 [9]. Similarly, the use of prime-boost approaches to boost and prolong memory responses is important but adds complexity to vaccine deployment. Higher doses of rVSV-ZEBOV vaccine are used to overcome acquired vector immunity [31]; however, higher vaccine doses have been associated with a greater frequency of adverse events [3].
Additional challenges for viral vectored vaccines can include requirements for significant cold-chain transport and storage (−80°C), and that vaccination can be associated with fevers or symptoms in some participants, possibly complicating field monitoring [32].
SynCon DNA vaccines do not elicit backbone-directed immune responses. In this clinical study, vaccines were administered 3 times with no negative impact on immunogenicity and continued immune boosting. This supports the potential to retain long-term immunity as well as reuse of DNA as a “vector” for additional vaccine candidates targeting other infectious disease pathogens in the same individuals. The formulations utilized for INO-4201 and INO-4202 impart temperature stability enabling storage at 4°C for more than 3 years, at least 1 year at 25°C, and 1 month at 37°C (unpublished data). The evaluation of INO-4201 for the revaccination of prior vaccinated persons whose immunity has waned or persons who cannot receive rVSV-ZEBOV [33] is of interest. A 2-dose immunization schedule could be used as preexposure prophylaxis to first responders, military personnel, those living in at-risk regions, and travelers. In the context of the WHO Ebola Vaccine Target Product Profile [34], ID delivery of INO-4201 addresses or surpasses the critical characteristics for reactive use that can be assessed at this stage of development. The EP device is portable and intermittent battery charging can be achieved through multiple methods (solar, kinetic) beyond standard electrical supplies (electrical grid, generators) that are less burdensome relative to cold-chain maintenance in low resource settings.
This study is the first direct clinical comparison of IM vs ID delivery using in vivo EP. The interim results of CELLECTRA EP-enhanced ID delivery showed improved tolerability and potency, suggesting that this could be an important new tool to impact EVD spread during future outbreaks. Importantly, this study highlights the strengths of ID delivery of the SynCon DNA platform that can be easily adapted to rapidly encode target antigens for any emerging infectious disease.
Supplementary Data
Supplementary materials are available at The Journal of Infectious Diseases online. Consisting of data provided by the authors to benefit the reader, the posted materials are not copyedited and are the sole responsibility of the authors, so questions or comments should be addressed to the corresponding author.
Acknowledgments. We thank the volunteers who participated in this study, the personnel at the clinical sites (QPS MRA in Miami, FL, the Center for Pharmaceutical Research in Kansas City, MO and the Perelman School of Medicine of the University of Pennsylvania in Philadelphia, PA), and the entire EBOV-001 clinical team.
Financial support. This work was supported by the Defense Advanced Research Projects Agency (grant number W31P4Q-15-1-0003BAA-14-38); and the W. W. Smith Charitable Trust (Chair in Cancer Research to D. B. W.).
Potential conflicts of interest. K. A. K., M. P. M., A. J. S., D. K., E. G., D. A., T. M., J. B., K. E. B., N. Y. S., M. B., and S. M. W. are employees of Inovio Pharmaceuticals and own shares or have been awarded stock options in the company. J. N. M., M. J., C. C. R., and Y. K. P. are employees of GeneOne Life Science and owns stock or stock options in the company. D. B. W. has received grant funding, participates in industry collaborations, has received speaking honoraria and fees for consulting, including serving on scientific review committees and board services; remuneration includes direct payments or stock or stock options, and in the interest of disclosure he notes potential conflicts associated with this work with Inovio, Pfizer, Merck, VGXi, MedImmune, and possibly others. In addition, D. B. W. has a patent DNA vaccine delivery pending to Inovio.
All other authors report no potential conflicts. All authors have submitted the ICMJE Form for Disclosure of Potential Conflicts of Interest. Conflicts that the editors consider relevant to the content of the manuscript have been disclosed.
Presented in part: International Society of Vaccines, October 2016, Boston, MA; American Association of Immunology, May 2017, Washington, DC, Abstract 225.9; and International Society of Vaccines, October 2018, Atlanta, GA.
References
Author notes
P. T., K. A. K., N. Y. S., and S. M. W. contributed equally to the research.
- cytokine
- enzyme-linked immunosorbent assay
- plasmids
- immune response
- ebola virus
- glycoproteins
- disease outbreaks
- dna
- drug administration routes
- electroporation
- ebola virus disease
- intramuscular injections
- interferons
- interleukin-12
- primates
- safety
- t-lymphocytes
- vaccines
- dna vaccines
- antibodies
- body temperature
- temperature
- human leukocyte interferon
- prevention
- immunogenicity