-
PDF
- Split View
-
Views
-
Cite
Cite
Trina Racine, Mélanie Denizot, Delphine Pannetier, Ludovic Nguyen, Anaïs Pasquier, Hervé Raoul, Jean-François Saluzzo, Gary Kobinger, Francisco Veas, Cécile H Herbreteau, In Vitro Characterization and In Vivo Effectiveness of Ebola Virus Specific Equine Polyclonal F(ab′)2, The Journal of Infectious Diseases, Volume 220, Issue 1, 1 July 2019, Pages 41–45, https://doi.org/10.1093/infdis/jiz068
- Share Icon Share
Abstract
There is no vaccine or approved therapy against lethal Ebola virus (EBOV). We investigated a proven technology platform to produce polyclonal IgG fragments, F(ab′)2, against EBOV. Horses immunized with nanoparticles harboring surface glycoprotein trimers of EBOV-Zaire/Makona produced anti-Ebola IgG polyclonal antibodies with high neutralization activity. Highly purified equine anti-Ebola F(ab′)2 showed strong cross-neutralization of 2 Zaire EBOV strains (Gabon 2001 and Makona) and in vivo 3 or 5 daily F(ab′)2 intraperitoneal injections provided 100% protection to BALB/c mice against lethal EBOV challenge. Rapid preparation of purified equine anti-Ebola F(ab′)2 offers a potentially efficient therapeutic approach against EBOV disease in humans.
Ebola virus (EBOV) is one of the most virulent representatives of the viral hemorrhagic fever group causing sporadic outbreaks of Ebola virus disease (EVD) in endemic regions of Central Africa [1]. The 2013–2016 EVD outbreak of the Makona variant of EBOV caused 28 616 probable, suspected, and laboratory-confirmed cases, including 11 310 deaths, mainly in Guinea, Liberia, and Sierra Leone [2]. More recently, and despite an intensive Ebola ring vaccination strategy involving more than 70 000 individuals, an ongoing Ebola Zaire outbreak in the Democratic Republic of Congo is continuing to grow with 699 accumulated infected patients, including 433 deaths, by the end of January 2019 [3].
After infection EBOV rapidly spreads within the body with a mean postexposure incubation period of 8 days, ranging from 2 to 21 days. Infection causes multiorgan dysfunction and loss of endothelial integrity with enhanced vascular leakage, shock, and disseminated intravascular coagulation, resulting in coagulopathy and intense generalized bleeding [4]. Death occurs within 5 to 8 days of symptom onset, similar to sepsis.
With no licensed vaccine [5], there is an urgent, major unmet medical need for an affordable, cross-reactive, therapeutic approach to improve prognosis of Ebola-infected patients and reduce person-to-person spread. Animal studies have shown passive transfer of antibodies or application of whole equine immunoglobulin and immunoglobulin fragments, F(ab′)2, protect against lethal EBOV challenge [6, 7]. These may offer more affordable options for routine use in low- and middle-income countries (LMIC) than current research on single or cocktails of multiple monoclonal antibodies (mAbs) [8, 9].
Equine polyclonal immunoglobulins with Fc cleavage and optimal purification of the residual F(ab′)2 are established for treatment for snake envenomation [10] or exposure to rabies virus [11], and are currently in clinical development for the treatment of avian influenza A (H5N1) [12]. Here we describe an application of this technology platform to produce equine anti-EBOV polyclonal IgG and purified F(ab′)2, with demonstration of their protective efficacy in a BALB/c mouse model of EVD.
METHODS
Preparation of Equine Anti-Ebola (Makona) Virus F(ab′)2
After screening for detectable antibodies against Ebola and other viruses, healthy French trotter horses were maintained in strictly controlled conditions according to the European Guidelines (CPMP/BWP/3354/99) in an Association for Assessment and Accreditation of Laboratory Animal Care-certified site. The study protocol was validated by the French ethical committee number 11, protocol authorization number: APAFIS#2254-2015101408317769 v2. For this report, 6 horses were hyperimmunized with micellar Zaire EBOV (Makona strain) nanoparticles harboring recombinant full-length surface glycoprotein GP1 and GP2 trimers produced using a baculovirus protein expression system (Novavax Inc., Gaithersburg, MD). Blood and plasma samples were collected regularly up to 9 months postimmunization and stored at −20°C.
Pooled horse plasma was progressively processed to obtain the highly purified anti-Ebola F(ab′)2 as previously described [13]. Molecular size distribution of immunoglobulin fragments was determined by high-performance liquid chromatography (HPLC) at different production stages and the final F(ab′)2 product (FBH-004) was analyzed by sodium dodecyl sulfate polyacrylamide gel electrophoresis (SDS-PAGE) according to Laemmli (BioRad, ref no.161-0747), with Coomassie Brilliant Blue staining. For this study we used FBH-004 lot LV15-0005 with a protein concentration of 25.5 g/L.
In Vitro ELISA Binding and Neutralization Assays
Equine plasma anti-Ebola IgG antibodies were monitored over the 9-month sampling period using a specific in-house enzyme-linked immunosorbent assay (ELISA), expressed in ELISA units (EU) to determine the average concentration obtained for 3 samples (at 3 different dilutions: 1/105, 1.5/105, and 1/106). These samples and subsequently purified anti-Ebola F(ab′)2 were tested in the absence of complement for neutralization activity in a 50% plaque reduction neutralization test (PRNT50) using Zaire EBOV (Gabon 2001 strain) at the INSERM Jean Mérieux Biosecurity Level 4 laboratory (BSL-4, Lyon, France). Neutralizing titers are expressed as: (1) a range of dilutions (between the 2 relevant dilutions including the dilution that neutralized 50% of the 200 focus-forming units EBOV dose) and (2) the precise PRNT50 titer using the Reed-Muench method.
In Vivo Ebola Virus-Challenge BALB/c Mouse Model
Mice experiments were performed in the BSL-4 laboratory at the National Microbiology Laboratory, Public Health Agency of Canada (PHAC, Winnipeg, Manitoba, Canada), as described in the Animal Use Document No. CSCHAH AUD# H-14-001 approved by the Animal Care Committee at the Canadian Science Centre for Human and Animal Health in accordance with the guidelines provided by the Canadian Council on Animal Care.
After 7 days, acclimation groups of 4–8-week-old BALB/c female mice (Charles River, Quebec, Canada), 10 mice per group, were challenged with 1000 median lethal dose (LD50) of mouse-adapted EBOV (Mayinga strain). From day 1 postchallenge, mice received either 5 or 3 daily doses of 2 mg of FBH-004, or 3 or 1 daily doses of 4 mg FBH-004. Control groups received either formulation buffer or were untreated. A positive control group of 5 mice was treated with 100 μg of anti-Ebola 2G4 mAb (kindly provided by Dr Qiu, PHAC) on day 1 postchallenge. Animals were observed and weighed daily until day 21, at which point all surviving animals were ethically euthanized.
Statistics
For analysis of time to death we performed a 1-way ANOVA with Tukey multiple comparisons tests with a single pooled variance on all groups that had mortality, using only animals that died (eg, in group 4, 1 mouse died on day 8 giving n = 1 for this group, while all the other groups had n = 9 or 10). With 4 groups used in the ANOVA (groups 1, 4, 5, and no treatment control), that lead to 6 comparisons (1 vs 4, 1 vs 5, 1 vs no treatment, 4 vs 5, 4 vs no treatment, and no treatment vs 5).
RESULTS
Horse Hyperimmunization and F(ab′)2 Preparation
Six healthy French trotter horses were hyperimmunized with EBOV VLPs. Generation of anti-Ebola polyclonal antibodies in each horse exhibited similar kinetics assessed by ELISA for anti-Ebola IgG and Zaire EBOV (Gabon strain) PRNT50 titers (Figure 1). Anti-Ebola IgG could be detected 1 month after the beginning of the immunization protocol with some natural variability observed between animals. Titers increased up to 4 months postimmunization, when levels plateaued until 9 months postimmunization.
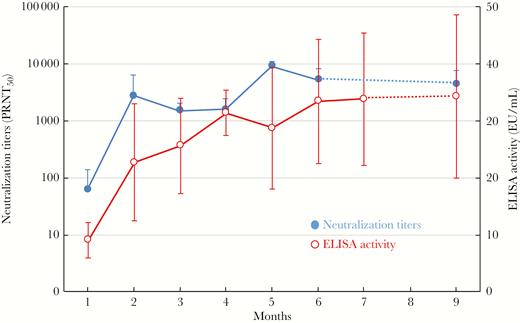
Development of anti-Ebola antibodies in hyperimmune horses. Horse plasma anti-Ebola IgG efficacy assessment was carried out over a 9-month period of horse hyperimmunizations using both an in-house enzyme-linked immunosorbent assay (ELISA) to detect antibodies to the Ebola-Gabon strain and a 50% plaque reduction neutralization test (PRNT50) to assess the neutralization capacity against the same strain of virus. Statistics were established with 6 individuals per group to calculate the mean and SD at each time point for both assays using the Mann and Whitney nonparametric method.
Similarly, neutralizing titers increased from zero preimmunization to a mean of 61 (range: 15 to 220) at month 1, then to 2742 (range: 5080 to 10 240, the maximal tested dilution) at month 2, after which there was a gradual increase in mean titer to 9022 (range: 4946 to 10 240) at month 5, where values plateaued until month 9 (Figure 1).
Purified F(ab′)2 (FBH-004) was prepared from hyperimmune plasma collected from months 2 to 6. In the experimental batch used in this study (LV15-0005) HPLC-exclusion size chromatography revealed that 79% of the antibodies present were F(ab′)2, 18% were Fab′, and 0.5% were aggregates and polymers. In SDS-PAGE most of the detectable product migrated at the expected 100 kDa size, with some degradation observed as a band at 50 kDa, but no detectable IgG contaminant at the expected 150–160 kDa size range.
Four batches of FBH-004 tested in PRNT assays against Gabon 2001 and Makona Zaire Ebola viruses demonstrated consistent anti-Ebola virus activity, with titers between 2560 and 10 240 for Gabon 2001 strain and around 10 240 for Makona strain.
Mouse Challenge
Control mice displayed rapid onset of clinical symptoms 3–4 days postchallenge, including significant weight loss (Figure 2A). All control animals died by day 8 postchallenge, except for 1 animal in the formulation buffer control group (Figure 2B). The challenged mice treated with a single 4-mg dose of FBH-004 on day 1 postchallenge also displayed significant weight loss and illness (Figure 2A, group 5). Death was delayed in group 5 compared with the no treatment group, with a hazard ratio (HR) of 3.13 (95% confidence interval [CI], 1.13–8.72), and compared with the group 1 formulation group, HR 2.34 (95% CI, 0.86–6.38), but all animals in group 5 eventually died by day 10 postchallenge. The only groups with high levels of lethality that could be tested statistically were the no treatment controls and groups 1 and 5. One-way ANOVA showed significant differences between groups 1 and 5, and the no treatment controls and group 5, with respective adjusted P values of .0003 and .0028.
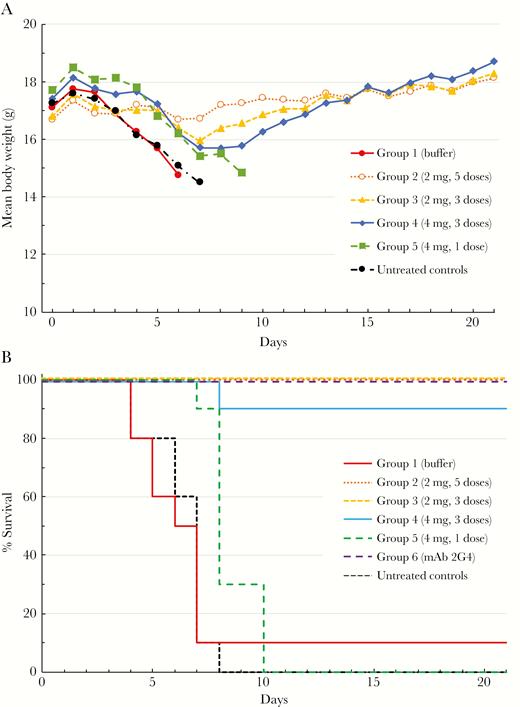
Protective efficacy of different treatment regimens of FBH-004. BALB/c mice were challenged with 1000 median lethal dose (LD50) of mouse-adapted Ebola virus and treated with either 2 mg (3 or 5 doses) or 4 mg (1 or 3 doses) of FBH-004 starting 1 day postinfection; (A) group mean body weights and (B) survival curves of experimental groups. Body weights represent means of 3 or more surviving animals from the 10 originally challenged in each group.
In contrast, animals treated with 5 daily 2 mg FBH-004 doses (group 2) did not exhibit any significant weight loss or clinical signs of illness and appeared fully protected against the lethal EBOV challenge. Some mice treated with 3 daily 2-mg (group 3) or 4-mg (group 4) doses of FBH-004 displayed low but apparent weight loss from which they fully recovered, except for 1 animal from group 4 that died on day 8. All animals treated with 2G4 anti-Ebola virus monoclonal antibody (group 6) survived lethal challenge with no signs of morbidity or mortality up to day 21 postchallenge.
DISCUSSION
Currently, with no approved specific therapy against EBOV infection, supportive care (including hydration, ventilation, analgesics) has a case fatality rate varying between 40% and 90%. Prophylactic vaccines against human Ebola virus infection are in the late stages of clinical development [5]. However, adequate vaccination coverage may not be achieved in LMIC, particularly in Ebola endemic areas, and so reliable, affordable, and rapidly available therapeutic interventions will still be required. Despite an extensive ring vaccination strategy during the current DRC Ebola outbreak, this epidemic is growing continuously and dangerously, highlighting the urgent need for additional therapeutic countermeasures [3]. Nonhuman primate (NHP) studies have demonstrated that passive transfer of polyclonal equine IgG raised against Ebola viruses could confer postexposure protection to animals exposed to a homologous viral challenge [7]. Anti-EBOV mAbs have been shown to be an effective postexposure treatment in rhesus macaques [14]; intravenous ZMapp, a cocktail of 3 anti-EBOV surface glycoprotein mAbs, protected macaques against a lethal Ebola infection [8] and has shown encouraging indications in Ebola-infected patients [9].
Innovative therapies such as mAb cocktails will probably be too expensive for routine use in these Ebola-susceptible regions. This situation mirrors that of rabies therapeutics, where treatment of potential rabies exposure with human rabies immunoglobulins (HRIG) is the gold standard, but limited supply and relatively high cost makes HRIG too difficult to obtain in countries with the highest demand. For these countries, the commercial production of equine rabies F(ab′)2, together with rabies vaccination form the standard protocol in rabies postexposure prophylaxis. Applying a similar methodology to rapidly produce a relatively low-cost, cross-protective treatment for EBOV infection could be a major breakthrough in the therapeutic armamentarium for this devastating disease.
Hyperimmunizing horses elicited strong IgG responses that plateaued 4 months postimmunization and then persisted for several months. Producing efficient specific IgG only 2 months after starting horse immunization could be key to the adoption of rapid countermeasures for this unmet medical need. We applied our ion-exchange chromatography protocol to prepare FBH-004, a highly purified anti-Ebola F(ab′)2 against a single strain of Zaire EBOV (Makona). In vitro FBH-004 neutralized various Zaire EBOV strains from Central Africa (obtained from outbreaks in 2001 and 2014) with similar titers (up to 10 240) to those detected in horse plasma before hydrolysis. This suggests that the preparation and purification processes do not adversely affect the cross-reactive neutralization capacities of these antibodies. Three or 5 daily doses of FBH-004 fully protected mice against a lethal mouse-adapted strain of EBOV, the 5-day regimen also being effective in preventing signs of illness or weight loss. These in vitro and in vivo results suggest that FBH-004 may have sufficient cross-reactivity to be of clinical benefit against newly emerging EBOV strains.
Further testing of the effectiveness of FBH-004 in guinea pig and NHP models of EBOV infection is ongoing or planned. Subsequent human tolerability testing, as recently performed for the polyclonal anti-H5N1 influenza F(ab′)2, is in preparation using the same World Health Organization and European Medicines Agency approved methodological approaches [15].
Results obtained with rabies, avian influenza H5N1, and now with Ebola clearly demonstrate that a polyclonal F(ab′)2 production platform represents a useful preparedness tool that can be efficiently used against many different viruses. Compared with other technologies, such as mAbs or antivirals, such a platform offers the additional advantages of being rapid and low cost, which may be key factors to address major public health concerns in LMIC. Furthermore, polyclonal F(ab′)2 could be combined with other therapeutic approaches to enhance the chances of treatment success.
Notes
Author contributions. M. D., L. N., A. P., J.-F. S., and C. H. H. managed the production of anti-Ebola F(ab′)2, performed the purity analyses, designed the experiments, and analyzed data. D. P. and H. R. assessed equine serum anti-Ebola virus seroneutralization activity, and T. R. and G. K. performed the BALB/c mice experiments. F. V. contributed to the study design and the coordination the Global IF-EBOLA European-USA project in which Fab’entech, T. R. and G. K. were partners. C. H. H. drafted the initial manuscript with the assistance of Keith Veitch (keithveitch communications, Amsterdam, the Netherlands) and all authors then contributed to editing the drafts and agreed to the submission of the final manuscript.
Acknowledgments. The authors thanks Larry Ellingsworth, Gregory Glenn, and Sven Andreasson from Novavax, Inc. for kindly providing Ebola antigen for horse immunization, and for reviewing the manuscript.
Financial support. This work was supported by the European Commission within the H2020 framework in the context of the Global IF-EBOLA European-US project (grant number 666102); the french Public Bank for Investment (BPI) within the industrial strategic innovation program (EMER-IT project), and by the study sponsor, Fab’entech.
Potential conflicts of interest. M. D., L. N., A. P., and C. H. H. are full-time employees of Fab’entech and J. F. S. works as external consultant for Fab’entech. All other authors report no potential conflicts. All authors have submitted the ICMJE Form for Disclosure of Potential Conflicts of Interest. Conflicts that the editors consider relevant to the content of the manuscript have been disclosed.
References
Author notes
T. R. and M. D. contributed equally to the work.