-
PDF
- Split View
-
Views
-
Cite
Cite
Chun-Ru Hsu, I-Wei Chang, Pei-Fang Hsieh, Tzu-Lung Lin, Pei-Yin Liu, Chen-Hsiu Huang, Kun-Tzu Li, Jin-Town Wang, A Novel Role for the Klebsiella pneumoniae Sap (Sensitivity to Antimicrobial Peptides) Transporter in Intestinal Cell Interactions, Innate Immune Responses, Liver Abscess, and Virulence, The Journal of Infectious Diseases, Volume 219, Issue 8, 15 April 2019, Pages 1294–1306, https://doi.org/10.1093/infdis/jiy615
- Share Icon Share
Abstract
Klebsiella pneumoniae is an important human pathogen causing hospital-acquired and community-acquired infections. Systemic K. pneumoniae infections may be preceded by gastrointestinal colonization, but the basis of this bacterium’s interaction with the intestinal epithelium remains unclear. Here, we report that the K. pneumoniae Sap (sensitivity to antimicrobial peptides) transporter contributes to bacterial–host cell interactions and in vivo virulence. Gene deletion showed that sapA is required for the adherence of a K. pneumoniae blood isolate to intestinal epithelial, lung epithelial, urinary bladder epithelial, and liver cells. The ΔsapA mutant was deficient for translocation across intestinal epithelial monolayers, macrophage interactions, and induction of proinflammatory cytokines. In a mouse gastrointestinal infection model, ΔsapA yielded significantly decreased bacterial loads in liver, spleen and intestine, reduced liver abscess generation, and decreased mortality. These findings offer new insights into the pathogenic interaction of K. pneumoniae with the host gastrointestinal tract to cause systemic infection.
Klebsiella pneumoniae is an important human pathogen that can cause hospital-acquired and community-acquired infections [1–3]. This organism causes nosocomial infections, including septicemia, pneumonia, urinary tract infections, surgical site infections, and catheter-related infections. A type of community-acquired K. pneumoniae that is associated with pyogenic liver abscess (PLA) has emerged. This invasive infection is often complicated by metastatic infections, such as meningitis and endophthalmitis. Diabetes mellitus, a predisposing factor, has been detected in about 50% of patients with PLA [3–5]. Mortality rates are 10% for patients with K. pneumoniae PLA alone [6] and 30%–40% for those with metastatic meningitis [7, 8]. K. pneumoniae strains with the K1 and K2 capsular types have been identified as the most prevalent in PLA [9, 10].
K. pneumoniae is a member in the human gut microbiota. Many K. pneumoniae infections may be preceded by gastrointestinal colonization [11–15]. Notably, strains isolated from patients with liver abscess and bacteremia possess genotypes similar to strains obtained from gastrointestinal reservoirs [12, 13]. An earlier study by Hsu et al [16] demonstrated that K. pneumoniae bacteremia and PLA isolates are able to invade and translocate across the intestinal epithelium via a transcellular pathway, a process that involves manipulation of host signaling machinery. These results imply that K. pneumoniae that have colonized the gut use this transcellular pathway to penetrate the intestinal barrier and access extraintestinal locations, thereby causing systemic infections. However, the mechanistic and molecular details of K. pneumoniae interactions with host intestinal epithelial cells remain unclear. The role of cell interactions in K. pneumoniae virulence needs to be elucidated.
The Sap (sensitivity to antimicrobial peptides) transporter of bacteria is a multifunctional inner membrane protein complex that belongs to the adenosine triphosphate–binding-cassette (ABC) transport family [17, 18]. Sap transporters were first identified in Salmonella based on the ability to transport antimicrobial peptides (AMPs); these peptides, which often demonstrate bactericidal activity, serve as a component of host innate immunity [17]. The Sap transporter consists of 5 proteins. SapA is a periplasmic substrate binding protein, SapB and SapC are permease proteins that form a pore in the bacterial inner membrane, and SapD and SapF provide the adenosine triphosphatase activity of the transporter. The Sap transporter confers resistance to human AMPs in several gram-negative pathogens, such as Salmonella enterica serovar Typhimurium, nontypeable Haemophilus influenzae, Proteus mirabilis, Escherichia coli, Vibrio fischeri, and Erwinia chrysanthemi [19–24]. Moreover, in Haemophilus, the Sap transporter was reported to transport heme iron for heme homeostasis [25]. However, the function of the Sap transporter in K. pneumoniae has not, to our knowledge, been reported. Notably, it remains unclear whether the Sap transporter is involved in K. pneumoniae–host cell interactions or in K. pneumoniae virulence.
In the current study, we demonstrated that the Sap transporter contributes to K. pneumoniae interactions with host intestinal cells. Screening of a mutant library disclosed that sapA is involved in the adherence of a blood isolate to human intestinal epithelial cells. Gene deletion and complementation showed that the Sap transporter promoted K. pneumoniae translocation across the intestinal epithelial monolayer, macrophage interactions, and cytokine induction. A mouse model of gastrointestinal infection demonstrated that Sap mediated bacterial colonization, liver abscess formation, and mouse mortality. Collectively, the K. pneumoniae Sap transporter is important for epithelial interactions, host innate immune responses, and in vivo virulence.
METHODS
Bacterial Strains and Plasmids
K. pneumoniae Ca0437 is a clinical isolate originally obtained from the blood of a patient with septicemia [26]. K. pneumoniae and E. coli strains were cultured in Luria-Bertani (LB) medium at 37°C. The bacterial strains, plasmids, and primers used in this study are listed in Supplementary Table 1.
Adherence, Invasion, and Translocation Assays
Caco-2 human intestinal epithelial cells and A549 human lung epithelial cells were grown in Dulbecco’s modified Eagle medium supplemented with 10% heat-inactivated fetal bovine serum (FBS) and 1% nonessential amino acids (Gibco). HepG2 human hepatocytes were grown in Dulbecco’s modified Eagle medium/F12 medium (1:1) supplemented with 10% FBS. T24 human urinary bladder epithelial cells were grown in McCoy’s 5a medium supplemented with 10% FBS. All cells were determined to be mycoplasma free using a polymerase chain reaction (PCR) Mycoplasma Detection Kit (Biosmart). Cells at passages 15–35 were used for all assays. The adherence assays and invasion assays of K. pneumoniae were performed as described elsewhere [16, 27]. Details of these 2 assays and library screening via adherence assays are described in the Supplementary Methods.
Translocation assays were used to assess the potential ability of K. pneumoniae to penetrate the intestinal epithelium, based on the method described elsewhere [16, 28]. Caco-2 cells can form tight polarized monolayers and differentiate to generate tight junctions (TJs) and a brush border, mimicking the human intestinal epithelium [28]. Caco-2 cells were grown on Transwell inserts (3-µm pore size and 0.33-cm2 filtration area) for 15 days to form tight polarized monolayers, assessed by transepithelial electrical resistance >300 Ω/cm2 [28]. Differentiation of Caco-2 monolayers was also confirmed by immunofluorescence staining of TJs (Supplementary Methods). In translocation assays, K. pneumoniae were added to the apical side of Caco-2 monolayers in the upper chambers of the Transwell inserts and incubated at 37°C for 1 hour. The numbers of viable bacteria in the upper and lower chambers were determined by plating appropriate dilutions on LB medium.
Macrophage Adherence, Phagocytosis, Cytokine Induction, and Serum Resistance Assays
RAW 264.7 murine macrophages were grown in RPMI 1640 medium supplemented with 10% FBS. K. pneumoniae adherence and phagocytosis by macrophages were determined according to methods described elsewhere [29]. For cytokine induction [30], RAW 264.7 cells were infected with K. pneumoniae for 1 hour at 37°C, washed with phosphate-buffered saline, and then incubated in gentamicin-containing medium. Culture supernatants were collected at various time points as indicated in Figure 3C. The levels of proinflammatory cytokines tumor necrosis factor (TNF) α and interleukin 6 (IL-6) were measured using enzyme-linked immunosorbent assay kits (R&D Systems), according to the manufacturer’s instructions. Serum resistance assays to determine the survival ratio of K. pneumoniae in human serum were performed as described elsewhere [31]. Values ≥1 were defined as serum resistance.
Mouse Inoculation
All animal experiments were approved by the Institutional Animal Care and Use Committee (IACUC) of the E-Da Hospital (IACUC-105013). All animal procedures were conducted in accordance with the recommendations of the Guide for the Care and Use of Laboratory Animals [32] from the National Institutes of Health and Taiwan’s Animal Protection Act.
K. pneumoniae in vivo virulence was evaluated by means of intragastric inoculation in a murine model. To analyze the survival of infected mice [33], we inoculated 5-week-old female BALB/cByl mice intragastrically with 4 × 108 colony-forming units (CFUs) of mid–log phase K. pneumoniae and monitored them for 30 days. Their in vivo bacterial load was determined as described elsewhere [33], by testing at either 24 or 72 hours after inoculation. Briefly, tissues were homogenized, and appropriate dilutions were plated for counting of recovered CFUs. For in vivo competition assays [34], the test strain (wild type (WT) or ΔsapA) was mixed with the fully virulent isogenic lacZ promoter deletion mutant (ΔplacZ) at a 1:1 ratio. Each BALB/cByl mouse was given an intragastric inoculation of 1 × 108 CFUs in 100 μL of saline solution. At 24 hours after inoculation, the liver and spleen were removed and homogenized in 1× phosphate-buffered saline; bacteria were recovered by plating appropriate dilutions on LB plates containing 1 mmol/mL isopropyl β-D-1-thiogalactopyranoside (IPTG) and 50 µg/mL X-Gal. The number of LacZ-positive (blue) and LacZ-negative (white) colonies were counted. The competitive index was defined as (test strain output/ΔplacZ output)/(test strain input/ΔplacZ input); the resulting ratio was interpreted as the in vivo colonization ability [34].
Histological Analysis
Five-week-old female BALB/cByl mice (4 per group) were infected intragastrically with K. pneumoniae at a dose of 1 × 108 CFUs per mouse for 72 hours or 6 × 108 CFUs for 120 hours. The liver and colon were retrieved, fixed in 10% formalin, and embedded in paraffin blocks. Sections were prepared at 5–10-μm thicknesses and stained with hematoxylin-eosin. Stained tissue sections were imaged under a light microscope for blinded evaluation and quantification by the pathologist. The number of abscesses was quantified in five low-power fields (×40 magnification). The total fields for observation equaled 118.79 mm2 (diameter, 5.5 mm).
Statistical Analyses
Data are presented as means with standard errors of the mean (SEM). Statistical significance was assessed using analysis of variance followed by a post hoc multiple comparisons test performed with Prism 5 software (GraphPad). Mouse survival was analyzed by means of Kaplan-Meier analysis using a log-rank test with Bonferroni correction. Differences were considered significant at P < .05.
RESULTS
Identification of Adherence-Deficient Mutants From a K. pneumoniae Mutant Library
To identify genes involved in K. pneumoniae adherence to enterocytes, we constructed a transposon mutant library of bacteremia-inducing strain Ca0437 (Supplementary Methods). A total of 2450 K. pneumoniae mutants were collected and screened by means of adherence assays using Caco-2 cells, with a Ca0437 WT parent and an inadherent strain 2084069 as controls (Supplementary Methods and Supplementary Table 1). Eight adherence-deficient mutants showing reduced adherence rates (compared with WT) were identified (Supplementary Figure 1); transposon insertion sites were determined (Table 1). Mutants harboring insertions in hns, sapA, and kpn_02265 were initially selected for further study, including verification by construction of in-frame unmarked deletion mutations in an isogenic background (Supplementary Methods).
Identification of Cell Adherence-Deficient Mutants from the Klebsiella pneumoniae Ca0437 Mutant Library
Mutant No. . | Tn-Inserted Genea . | Predicted Gene Functionb . | Accession No. . | Amino Acid Ssequence Similarity,% . | Relative Adherence, Mean (SEM), %c . |
---|---|---|---|---|---|
8-1G | hflX | GTPase HflX | CDL63628.1 | 100 | 57.3 (4.4) |
9-6E | hns | DNA-binding protein H-NS | CP002910.1 | 97 | 0.8 (0.8) |
9-10E | sapA | Antimicrobial peptide ABC transporter periplasmic binding protein SapA | CP002910.1 | 96 | 18.4 (5.9) |
11-2A | kpn_02265 | Putative transmembrane protein containing GGDEF domain | WP_002887653 | 100 | 18.1 (3.1) |
12-1B | trkA | Potassium transporter peripheral membrane protein | WP_002919153.1 | 97 | 15.7 (11.5) |
21-11A | kpn_25946 | Hypothetical protein (plasmid pKCTC2242) | AEK01010.1 | 93 | 53.7 (11.7) |
25-12G | kpn_07830 | Murein L,D-transpeptidase | WP_048333314.1 | 100 | 67.3 (19.4) |
26-6B | degP/htrA | Serine endoprotease | WP_004177363.1 | 100 | 29.5 (10.2) |
Mutant No. . | Tn-Inserted Genea . | Predicted Gene Functionb . | Accession No. . | Amino Acid Ssequence Similarity,% . | Relative Adherence, Mean (SEM), %c . |
---|---|---|---|---|---|
8-1G | hflX | GTPase HflX | CDL63628.1 | 100 | 57.3 (4.4) |
9-6E | hns | DNA-binding protein H-NS | CP002910.1 | 97 | 0.8 (0.8) |
9-10E | sapA | Antimicrobial peptide ABC transporter periplasmic binding protein SapA | CP002910.1 | 96 | 18.4 (5.9) |
11-2A | kpn_02265 | Putative transmembrane protein containing GGDEF domain | WP_002887653 | 100 | 18.1 (3.1) |
12-1B | trkA | Potassium transporter peripheral membrane protein | WP_002919153.1 | 97 | 15.7 (11.5) |
21-11A | kpn_25946 | Hypothetical protein (plasmid pKCTC2242) | AEK01010.1 | 93 | 53.7 (11.7) |
25-12G | kpn_07830 | Murein L,D-transpeptidase | WP_048333314.1 | 100 | 67.3 (19.4) |
26-6B | degP/htrA | Serine endoprotease | WP_004177363.1 | 100 | 29.5 (10.2) |
Abbreviations: ABC, adenosine triphosphate–binding-cassette; GTPase, guanosinetriphosphatase; SEM, standard error of the mean; Tn, transposon.
aCompared with the Klebsiella pneumoniae KCTC2242 complete genome from the National Center for Biotechnology Information (NCBI) as the template genome.
bAnalysis performed with the NCBI Basic Local Alignment Search Tool.
cCompared with the ca0437 wild-type strain (100%).
Identification of Cell Adherence-Deficient Mutants from the Klebsiella pneumoniae Ca0437 Mutant Library
Mutant No. . | Tn-Inserted Genea . | Predicted Gene Functionb . | Accession No. . | Amino Acid Ssequence Similarity,% . | Relative Adherence, Mean (SEM), %c . |
---|---|---|---|---|---|
8-1G | hflX | GTPase HflX | CDL63628.1 | 100 | 57.3 (4.4) |
9-6E | hns | DNA-binding protein H-NS | CP002910.1 | 97 | 0.8 (0.8) |
9-10E | sapA | Antimicrobial peptide ABC transporter periplasmic binding protein SapA | CP002910.1 | 96 | 18.4 (5.9) |
11-2A | kpn_02265 | Putative transmembrane protein containing GGDEF domain | WP_002887653 | 100 | 18.1 (3.1) |
12-1B | trkA | Potassium transporter peripheral membrane protein | WP_002919153.1 | 97 | 15.7 (11.5) |
21-11A | kpn_25946 | Hypothetical protein (plasmid pKCTC2242) | AEK01010.1 | 93 | 53.7 (11.7) |
25-12G | kpn_07830 | Murein L,D-transpeptidase | WP_048333314.1 | 100 | 67.3 (19.4) |
26-6B | degP/htrA | Serine endoprotease | WP_004177363.1 | 100 | 29.5 (10.2) |
Mutant No. . | Tn-Inserted Genea . | Predicted Gene Functionb . | Accession No. . | Amino Acid Ssequence Similarity,% . | Relative Adherence, Mean (SEM), %c . |
---|---|---|---|---|---|
8-1G | hflX | GTPase HflX | CDL63628.1 | 100 | 57.3 (4.4) |
9-6E | hns | DNA-binding protein H-NS | CP002910.1 | 97 | 0.8 (0.8) |
9-10E | sapA | Antimicrobial peptide ABC transporter periplasmic binding protein SapA | CP002910.1 | 96 | 18.4 (5.9) |
11-2A | kpn_02265 | Putative transmembrane protein containing GGDEF domain | WP_002887653 | 100 | 18.1 (3.1) |
12-1B | trkA | Potassium transporter peripheral membrane protein | WP_002919153.1 | 97 | 15.7 (11.5) |
21-11A | kpn_25946 | Hypothetical protein (plasmid pKCTC2242) | AEK01010.1 | 93 | 53.7 (11.7) |
25-12G | kpn_07830 | Murein L,D-transpeptidase | WP_048333314.1 | 100 | 67.3 (19.4) |
26-6B | degP/htrA | Serine endoprotease | WP_004177363.1 | 100 | 29.5 (10.2) |
Abbreviations: ABC, adenosine triphosphate–binding-cassette; GTPase, guanosinetriphosphatase; SEM, standard error of the mean; Tn, transposon.
aCompared with the Klebsiella pneumoniae KCTC2242 complete genome from the National Center for Biotechnology Information (NCBI) as the template genome.
bAnalysis performed with the NCBI Basic Local Alignment Search Tool.
cCompared with the ca0437 wild-type strain (100%).
Contribution of hns and sapA to K. pneumoniae Adherence to Different Epithelial Cells
First, we assessed host cell adherence by the Δhns, ΔsapA, and Δkpn_02265 gene deletion mutants. Specifically, the bacterial mutants were tested for adherence to 4 types of cells (Figure 1). Compared with the WT strain, the Δhns and ΔsapA strains showed significantly decreased adherence to all tested cell types. The Δkpn_02265 strain exhibited decreased adherence to Caco-2 cells but no significant reduction in adherence to HepG2, A549, or T24 cells. A recent parallel study of a K. pneumoniae pneumonia–causing isolate found that hns, which encodes the putative global gene regulator H-NS, contributed to adherence [29]. The remainder of the present study focused on sapA.
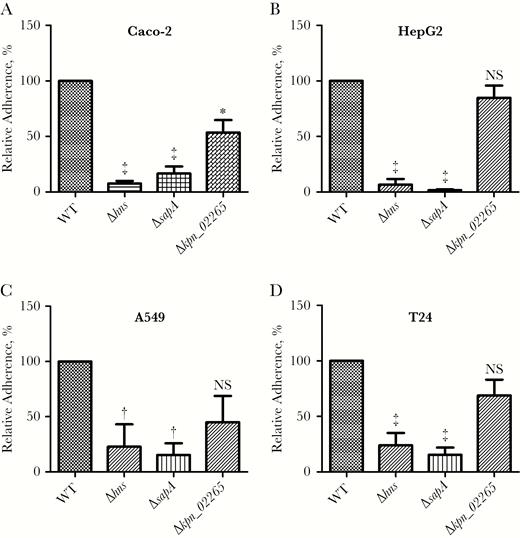
Adherence of Klebsiella pneumoniae Ca0437 wild type (WT) and Δhns, ΔsapA, and Δkpn_02265 deletion mutants to various cell lines, including colonic epithelial cells Caco-2 (A), hepatocytes HepG2 (B), lung epithelial cells A549 (C), and urinary bladder epithelial cells T24 (D). Mid–log phase K. pneumoniae were added to cells of the indicated cell line, and the mixture was incubated for 15 minutes, washed with phosphate-buffered saline wash, lysed with Triton X-100, and plated for colony-forming unit counts. The adherence rates of the isogenic mutants were normalized to that of the WT parent strain (defined as 100%). Bars represent the mean and standard error of the mean from ≥3 independent experiments. *P < .05; †P < .01; ‡P < .001 (comparison with WT; analysis of variance followed by Dunnett multiple comparisons test). Abbreviation: NS, not significant.
We tested whether the defects in adherence to the colonic epithelial cells could be overcome by genetic complementation (Supplementary Methods). Complementation of ΔsapA with sapABC (3 genes) provided significant restoration of adherence to Caco-2 cells (Figure 2A; ΔsapA+sapABC), although complementation of ΔsapA with sapA alone (ΔsapA+sapA) was not sufficient to restore the defective function. Reverse-transcription quantitative PCR (Supplementary Methods) showed that, in the ΔsapA mutant, the expression levels of sapA, sapB, and sapC were all decreased compared with WT (Supplementary Figure 2A). Expression of these 3 genes was rescued by complementation with sapABC (ΔsapA+sapABC); complementation with sapA alone (ΔsapA+sapA) did not restore the decreased expression of sapB and sapC observed with ΔsapA. No significant differences in bacterial growth were observed among WT, ΔsapA, and ΔsapA+sapABC strains (Supplementary Figure 2B). Adherence assays using the differentiated Caco-2 monolayers also showed that sapA deletion caused the decrease of adherence, with complementation by ΔsapA+sapABC (Supplementary Figure 3A and 3B).
![The Klebsiella pneumoniae Sap (sensitivity to antimicrobial peptides) transporter promotes intestinal cell adherence and epithelial translocation. A, Adherence assays showing complementation of ΔsapA in K. pneumoniae. Human Caco-2 cells were infected with K. pneumoniae Ca0437 wild type (WT), ΔsapA deletion mutant, ΔsapA+sapA complementation strain, or ΔsapA+sapABC complementation strain. Genetic complementation of sapABC in K. pneumoniae ΔsapA strain (ΔsapA+sapABC) overcame ΔsapA-associated defects in cell adherence. Bars represent the mean and standard error of the mean (SEM) from ≥3 independent experiments. B, Three-dimensional confocal micrograph showing invasion into Caco-2 intestinal epithelial cells by K. pneumoniae Ca0437. Cells were infected with K. pneumoniae Ca0437 carrying pCRII-TOPO::green fluorescent protein (green) for 2 hours. The actin cytoskeleton and DNA were stained with rhodamine-phalloidin (red) and 4',6-diamidino-2-phenylindole (blue). Images from confocal microscopy with Z-stacking were analyzed by XY, XZ, and YZ sections. Intracellular K. pneumoniae is indicated by white arrows. C, Invasion assays into intestinal cells. Invasion of Caco-2 cells by K. pneumoniae Ca0437 WT, ΔsapA, or ΔsapA+sapABC complementation strain was quantified using a gentamicin-based assay (Supplementary Methods). The invasive index (number of invaded bacterial colony-forming units [CFUs] divided by the number of adherent bacterial CFUs) of strains was expressed [35]. Bars represent the mean and SEM from 4 independent experiments D, Translocation assays across intestinal epithelial monolayers. Caco-2 cells were grown on Transwell inserts for 15 days to form a tight polarized monolayer; monolayers then were infected with K. pneumoniae Ca0437 WT, ΔsapA, or ΔsapA+sapABC strains for 1 hour, followed by recovery and quantification of CFUs of in the lower chambers (translocated bacteria). Bars represent the mean and SEM from 4 independent experiments. *P < .05; †P < .01; ‡P < .001 (analysis of variance followed by Bonferroni multiple comparisons test). Abbreviation: NS, not significant.](https://oup.silverchair-cdn.com/oup/backfile/Content_public/Journal/jid/219/8/10.1093_infdis_jiy615/3/m_jiy61502.jpeg?Expires=1749814104&Signature=kh0AyS6aKFFQttg7~PyXzRQ~~Xx0x4kBia49q6ASdFc~cl~JsoUuF6-4-vFzq-3D4UWvpAg9FClTE14QYkgBLB40-RjUNPe98GJ0qgjgPIcRCR3bPvNHab0wlHG9Fq5~sJP4dG3aC4rgdn7UhQd~VsQSlf~Fu0BddvhL8r6sJ0tCmos2M~uu2XtJlSOvWLAy7ceNGglaMqGgoojitS-MKXG1fKNIqTt-gELyoPDlTHvV3T4TExaeVSm1JeVL9oj5JMhzb6jQtIrOLEUZueL016pjUezeTsulF0p16hRY3EqwC82kI2FzfffN1VDg81x43qUjSSyNYJoA3CTq9Lmnaw__&Key-Pair-Id=APKAIE5G5CRDK6RD3PGA)
The Klebsiella pneumoniae Sap (sensitivity to antimicrobial peptides) transporter promotes intestinal cell adherence and epithelial translocation. A, Adherence assays showing complementation of ΔsapA in K. pneumoniae. Human Caco-2 cells were infected with K. pneumoniae Ca0437 wild type (WT), ΔsapA deletion mutant, ΔsapA+sapA complementation strain, or ΔsapA+sapABC complementation strain. Genetic complementation of sapABC in K. pneumoniae ΔsapA strain (ΔsapA+sapABC) overcame ΔsapA-associated defects in cell adherence. Bars represent the mean and standard error of the mean (SEM) from ≥3 independent experiments. B, Three-dimensional confocal micrograph showing invasion into Caco-2 intestinal epithelial cells by K. pneumoniae Ca0437. Cells were infected with K. pneumoniae Ca0437 carrying pCRII-TOPO::green fluorescent protein (green) for 2 hours. The actin cytoskeleton and DNA were stained with rhodamine-phalloidin (red) and 4',6-diamidino-2-phenylindole (blue). Images from confocal microscopy with Z-stacking were analyzed by XY, XZ, and YZ sections. Intracellular K. pneumoniae is indicated by white arrows. C, Invasion assays into intestinal cells. Invasion of Caco-2 cells by K. pneumoniae Ca0437 WT, ΔsapA, or ΔsapA+sapABC complementation strain was quantified using a gentamicin-based assay (Supplementary Methods). The invasive index (number of invaded bacterial colony-forming units [CFUs] divided by the number of adherent bacterial CFUs) of strains was expressed [35]. Bars represent the mean and SEM from 4 independent experiments D, Translocation assays across intestinal epithelial monolayers. Caco-2 cells were grown on Transwell inserts for 15 days to form a tight polarized monolayer; monolayers then were infected with K. pneumoniae Ca0437 WT, ΔsapA, or ΔsapA+sapABC strains for 1 hour, followed by recovery and quantification of CFUs of in the lower chambers (translocated bacteria). Bars represent the mean and SEM from 4 independent experiments. *P < .05; †P < .01; ‡P < .001 (analysis of variance followed by Bonferroni multiple comparisons test). Abbreviation: NS, not significant.
Effect of the Sap Transporter in Promoting K. pneumoniae Translocation Across Intestinal Epithelium
Confocal microscopy showed that K. pneumoniae Ca0437 WT invaded and was internalized into Caco-2 cells (Figure 2B). Invasion assays revealed that the invasive index (after normalization for adherence) [35] of ΔsapA did not differ significantly from that of the WT strain (Figure 2C), although the relative invasion rate (without normalization) was lower (Supplementary Figure 4).
Translocation assays were used to determine whether sapA deletion influenced K. pneumoniae translocation across Caco-2–polarized epithelial monolayers on the Transwell inserts. Ca0437 WT translocated across Caco-2 monolayers, such that bacteria were recovered from the medium in the lower chamber (Figure 2D). In contrast, ΔsapA exhibited a defect in transepithelial translocation. The ΔsapA+sapABC complementation strain counteracted the translocation defect.
Sap Transporter Enhancement of K. pneumoniae–Macrophage Interactions and Cytokine Induction
Macrophage adherence assays showed that ΔsapA was significantly less adherent to Raw 264.7 macrophages, with relative adherence 2.8% that of the WT strain (Figure 3A). K. pneumoniae phagocytosis by macrophages was diminished in ΔsapA, with relative phagocytosis 0.5% that of the WT strain (Figure 3B). For both macrophage adherence and phagocytosis assays, the ΔsapA+sapABC complementation strain exhibited a recovery from the defects (Figure 3A and 3B).
![The Klebsiella pneumoniae Sap (sensitivity to antimicrobial peptides) transporter contributes to macrophage adherence, phagocytosis, and secretion of proinflammatory cytokines. A, Macrophage adherence assays. Mid–log phase K. pneumoniae Ca0437 wild type (WT), ΔsapA, or ΔsapA+sapABC was added to RAW 264.7 macrophages in 24-well plates for 1-hour incubation at 4°C, followed by phosphate-buffered saline wash, Triton X-100 lysis of eukaryotic cells, and colony-forming unit (CFU) recovery for plate counts. B, Phagocytosis assays. RAW 264.7 macrophages were infected by mid–log phase K. pneumoniae Ca0437 WT, ΔsapA, and ΔsapA+sapABC for 2 hours at 37°C. Phagocytosed bacteria cells then were quantified using a gentamicin-based assay (see Materials and Methods). C, Induction of cytokines tumor necrosis factor (TNF) α and interleukin 6 (IL-6). RAW 264.7 macrophages were incubated with K. pneumoniae Ca0437 WT (white bars) or ΔsapA (black bars), and the levels of secreted cytokines in the medium were measured at the indicated time points. D, Serum resistance assays. The indicated strains of K. pneumoniae were incubated with human serum (75%) at 37°C for 1 hour. The numbers of recovered CFUs were determined, and the survival ratio was expressed as recovered CFUs/inoculum CFUs. K. pneumoniae Ca0437 WT strain and the Ca0437 ΔsapA mutant were both serum resistant. K. pneumoniae 4425/51 is a serum-sensitive strain for comparison [30]. Throughout figure, data represent the mean and standard error of the mean from 3 independent experiments. †P < .01; ‡P < .001 (analysis of variance followed by Bonferroni multiple comparisons test). Abbreviation: NS, not significant.](https://oup.silverchair-cdn.com/oup/backfile/Content_public/Journal/jid/219/8/10.1093_infdis_jiy615/3/m_jiy61503.jpeg?Expires=1749814104&Signature=mDfclxVBv2TGAF3uKz7N1aOElfc6yrMvXH4VA9QNHytWmdsCI-KejQ5jvRNZObmgEnj8SGQsc2O~SDCuqf6CJVcrH00OQJ-c3O1eG0soKdtDvcgJXUlD7KtC~Lx9toWpXzhbTdViQr~5cKErc8ZoAdW~Ltn3Do-DOv2nXRDRo1Vb1Dw3zsWHJKRuxXMDKgqjr8CrpWLypgFqOoJgsRhfYgBd1RubOprfjkYbSqWU3Vwe-n2UCVOW3eksIAhV8VTogvKZ-OIDJCALHC-TUTE7r8BUaUDOQl-KXsgEFTftTDZr0~sN8LZ1oQ3k8U~6LmRlglOUcgYShkjpDRyauxWNwA__&Key-Pair-Id=APKAIE5G5CRDK6RD3PGA)
The Klebsiella pneumoniae Sap (sensitivity to antimicrobial peptides) transporter contributes to macrophage adherence, phagocytosis, and secretion of proinflammatory cytokines. A, Macrophage adherence assays. Mid–log phase K. pneumoniae Ca0437 wild type (WT), ΔsapA, or ΔsapA+sapABC was added to RAW 264.7 macrophages in 24-well plates for 1-hour incubation at 4°C, followed by phosphate-buffered saline wash, Triton X-100 lysis of eukaryotic cells, and colony-forming unit (CFU) recovery for plate counts. B, Phagocytosis assays. RAW 264.7 macrophages were infected by mid–log phase K. pneumoniae Ca0437 WT, ΔsapA, and ΔsapA+sapABC for 2 hours at 37°C. Phagocytosed bacteria cells then were quantified using a gentamicin-based assay (see Materials and Methods). C, Induction of cytokines tumor necrosis factor (TNF) α and interleukin 6 (IL-6). RAW 264.7 macrophages were incubated with K. pneumoniae Ca0437 WT (white bars) or ΔsapA (black bars), and the levels of secreted cytokines in the medium were measured at the indicated time points. D, Serum resistance assays. The indicated strains of K. pneumoniae were incubated with human serum (75%) at 37°C for 1 hour. The numbers of recovered CFUs were determined, and the survival ratio was expressed as recovered CFUs/inoculum CFUs. K. pneumoniae Ca0437 WT strain and the Ca0437 ΔsapA mutant were both serum resistant. K. pneumoniae 4425/51 is a serum-sensitive strain for comparison [30]. Throughout figure, data represent the mean and standard error of the mean from 3 independent experiments. †P < .01; ‡P < .001 (analysis of variance followed by Bonferroni multiple comparisons test). Abbreviation: NS, not significant.
The influence of the sapA deletion on the proinflammatory responses was assessed. Enzyme-linked immunosorbent assay showed that Ca0437 WT induced secretion of the cytokines TNF-α and IL-6 (Figure 3C). However, ΔsapA yielded decreased induction (compared with WT) of both TNF-α and IL-6. Serum resistance of the ΔsapA mutant did not differ significantly from that of the WT strain (Figure 3D).
Influence of the Sap Transporter on Type I Fimbriae Expression and AMP Resistance
Fimbriae (pili) and capsular polysaccharides have been implicated in K. pneumoniae adherence [27, 36]. We compared the expression of type I fimbrial genes (fimA and fimC), type III fimbrial genes (mrkA and mrkD), and galF in the capsular polysaccharide synthesis (cps) gene cluster (Figure 4A). Reverse-transcription quantitative PCR showed that fimA and fimC expression was significantly decreased in ΔsapA (compared with the WT strain). The expression of mrkA and mrkD, and that of the cps gene galF, was not significantly changed in ΔsapA.
![The Klebsiella pneumoniae Sap (sensitivity to antimicrobial peptides) transporter influences transcriptional expression of type I fimbriae and antimicrobial peptide (AMP) resistance of K. pneumoniae. A, Quantitative real-time expression of capsular polysaccharide synthesis (cps) and fimbrial genes. The cps gene galF, type I fimbrial genes fimA and fimC, and type III fimbrial genes mrkA and mrkD in Ca0437 wild type (WT), ΔsapA, and the complementation strain ΔsapA+sapABC were determined with reverse-transcription quantitative polymerase chain reaction (RT-qPCR). B, Sensitivity tests of K. pneumoniae to human AMP LL-37 (left) and human β-defensin 2 (HBD-2) (right). K. pneumoniae Ca0437 WT, ΔsapA, ΔsapA+sapABC, and Escherichia coli American Type Culture Collection (ATCC) 25922 were incubated with LL-37 (2 μg/mL) or HBD-2 (0.5 μg/mL), respectively. The survival of bacteria (percentage of colony-forming units of control wells not exposed to AMP) is shown. For HBD-2, there were no significant differences between all tested strains. C, Heme use of K. pneumoniae Ca0437 WT and ΔsapA. Bacteria were starved for heme iron for 24 hours and then shifted to DIS cultures supplemented with (+ heme, 10 μg/mL) or without heme (− heme). Growth was assessed by monitoring the increase in culture absorbance (optical density at 600 nm [OD600]) at the time points indicated. D, Quantitative expression of heme system gene in K. pneumoniae Ca0437 WT and ΔsapA. RNA transcripts of hmuR were determined with real-time RT-qPCR. There were no significant differences between WT and ΔsapA (Student t test). E, Outer-membrane permeability of K. pneumoniae. The leakage of the periplasmic enzyme β-lactamase into the culture supernatants of Ca0437 WT, ΔsapA, and ΔsapA+sapABC was determined. Throughout figure, data represent the mean and standard error of the mean from 3 independent experiments. *P < .05; †P < .01 (analysis of variance followed by Bonferroni multiple comparisons test. Abbreviation: NS, not significant.](https://oup.silverchair-cdn.com/oup/backfile/Content_public/Journal/jid/219/8/10.1093_infdis_jiy615/3/m_jiy61504.jpeg?Expires=1749814104&Signature=xuIeJGTdYN-2FmVh6cA9~Ai2igr5-CUWEBDwJ6ebwUwtcsP3Uc~uKkdlr3ZmOqMbyFn6LQMebB~BDdgBS1kBlWD5X5s9Y8Hi-ho46H6NtJfDmQdTkiUOvMoPPodYcPWV5jSySJ-LcNjaflJvJPbsq9hXPF5~tMZLp5V8EDQboOJ5Ms60~GlLivsEQpdNlJgYP-tT8xhAs2GevTD0G~WRT7Zsaxj5JXYT0I6Gwscel4jTn7HU6qh4rG6EofVXHGzXRzdOIKGIfNzdep0r1LjBEoDYEnSWy3AY2S1j1IjTYQLjObj0JGJrjIBnl65~veN0UrgUsV6x-wZDMqX4hMkQSA__&Key-Pair-Id=APKAIE5G5CRDK6RD3PGA)
The Klebsiella pneumoniae Sap (sensitivity to antimicrobial peptides) transporter influences transcriptional expression of type I fimbriae and antimicrobial peptide (AMP) resistance of K. pneumoniae. A, Quantitative real-time expression of capsular polysaccharide synthesis (cps) and fimbrial genes. The cps gene galF, type I fimbrial genes fimA and fimC, and type III fimbrial genes mrkA and mrkD in Ca0437 wild type (WT), ΔsapA, and the complementation strain ΔsapA+sapABC were determined with reverse-transcription quantitative polymerase chain reaction (RT-qPCR). B, Sensitivity tests of K. pneumoniae to human AMP LL-37 (left) and human β-defensin 2 (HBD-2) (right). K. pneumoniae Ca0437 WT, ΔsapA, ΔsapA+sapABC, and Escherichia coli American Type Culture Collection (ATCC) 25922 were incubated with LL-37 (2 μg/mL) or HBD-2 (0.5 μg/mL), respectively. The survival of bacteria (percentage of colony-forming units of control wells not exposed to AMP) is shown. For HBD-2, there were no significant differences between all tested strains. C, Heme use of K. pneumoniae Ca0437 WT and ΔsapA. Bacteria were starved for heme iron for 24 hours and then shifted to DIS cultures supplemented with (+ heme, 10 μg/mL) or without heme (− heme). Growth was assessed by monitoring the increase in culture absorbance (optical density at 600 nm [OD600]) at the time points indicated. D, Quantitative expression of heme system gene in K. pneumoniae Ca0437 WT and ΔsapA. RNA transcripts of hmuR were determined with real-time RT-qPCR. There were no significant differences between WT and ΔsapA (Student t test). E, Outer-membrane permeability of K. pneumoniae. The leakage of the periplasmic enzyme β-lactamase into the culture supernatants of Ca0437 WT, ΔsapA, and ΔsapA+sapABC was determined. Throughout figure, data represent the mean and standard error of the mean from 3 independent experiments. *P < .05; †P < .01 (analysis of variance followed by Bonferroni multiple comparisons test. Abbreviation: NS, not significant.
We characterized the functions of the K. pneumoniae Sap transporter in AMPs interactions and heme use. We tested cathelicidin LL-37 and human β-defensin 2, 2 human AMPs present in the gut epithelium [37]. Although K. pneumoniae Ca0437 WT was resistant against LL-37, ΔsapA became sensitive (Figure 4B, left). No differences in the susceptibility to human β-defensin 2 were observed between Ca0437 WT, ΔsapA, ΔsapA+sapABC, and E. coli American Type Culture Collection 25922 (Figure 4B, right). Involvement of the K. pneumoniae Sap transporter in heme iron use was not observed (Figure 4C). Although heme iron–starved WT bacteria failed to grow in heme iron–depleted medium, growth was restored when cultured in the presence of heme iron. Similar to WT, heme iron–starved ΔsapA was able to use heme to grow again in the presence of heme. Expression of the heme system hmuR gene in ΔsapA did not differ significantly from that in the WT strain (Figure 4D). Outer membrane permeability was similar in WT, ΔsapA, and ΔsapA+sapABC (Figure 4E), indicating that sapA deletion might not cause a broad disruption of the outer membrane. In conclusion, the Sap transporter in K. pneumoniae–mediated bacterial resistance against AMP LL-37, but its effects on heme acquisition were not observed.
Contribution of the Sap Transporter to K. pneumoniae Virulence and Liver Abscess Formation In Vivo
We used a murine gastrointestinal infection model to investigate the role of the Sap transporter in K. pneumoniae virulence. Mouse survival was monitored after intragastric inoculation. By 30 days after infection, 7 of 8 WT-infected mice had died (survival rate, 12.5%), compared with 1 of 8 ΔsapA-infected mice (survival rate, 87.5%); the virulence of ΔsapA was significantly attenuated (Figure 5A, WT vs ΔsapA). The complementation strain caused death in 5 of 8 mice (survival rate, 37.5%), not significantly different from the WT strain (Figure 5A, WT vs ΔsapA+sapABC).
![The Klebsiella pneumoniae Sap (sensitivity to antimicrobial peptides) transporter contributes to the in vivo virulence of K. pneumoniae during gastrointestinal infection. A, Mouse survival analysis. Female BALB/cByl mice (8 per group) were infected intragastrically with K. pneumoniae Ca0437 wild type (WT), sapA, or ΔsapA+sapABC at a dose of 4 × 108 colony-forming units (CFUs) per mouse. Survival of mice was monitored for 4 weeks. B, In vivo bacterial loads in liver and spleen. WT, ΔsapA or ΔsapA+sapABC was administered intragastrically to female BALB/cByl mice (4 per group) at an inoculation dose of either 4 × 108 CFUs for 24 hours or 1 × 108 CFUs for 72 hours. Bacterial levels in liver and spleen were determined at 24 (right) or 72 (left) hours after infection. Bacterial numbers (expressed as log10 CFUs) were standardized per 0.1 g of wet organ weight. C, In vivo competition assays. Either test strain (WT, ΔsapA, or ΔsapA+sapABC) was compared with the ΔplacZ mutant strain in female BALB/cByl mice (8 per group) by intragastric inoculation with 1 × 108 CFUs per strain per mouse. After bacterial recovery on isopropyl β-D-1-thiogalactopyranoside (IPTG)/X-Gal plates, the ratio of LacZ-positive (blue) to LacZ-negative (white) colonies in the liver or spleen of each mouse was determined. The competitive index was defined as (ΔplacZ output/test strain output)/(ΔplacZ input/test strain input). Each symbol represents the index for each inoculum, and bars represent medians. D, Intestinal colonization in vivo. K. pneumoniae were administered intragastrically to each of female BALB/cByl mice (4 per group) at an inoculation dose of 4 × 108 CFUs. Bacterial numbers (expressed as log10 CFUs) in the colorectum were determined 72 hours after infection. *P < .05; †P < .01; ‡ P < .001 (log-rank test [A] or analysis of variance [B–D] with Bonferroni multiple comparisons test). Abbreviation: NS, not significant.](https://oup.silverchair-cdn.com/oup/backfile/Content_public/Journal/jid/219/8/10.1093_infdis_jiy615/3/m_jiy61505.jpeg?Expires=1749814104&Signature=r1RmB6BdLvqOAOqoDmqR4v5M-U1aSbkwPX-xKeLN60ckQ2lb127~fPcDdLAk0ZE4-z8O4MTKQT8OXEW4r~62bqbCf8HCfbFUeskNDBNuSp2DlLFngGVIO4zfbCvdlYOR3Z5cNYI7i8ngQkKC6yhiFL~4xwGy-uJri0PyM45HQjgEaYX30AF~HFlLUwwUujlj5GdHd-D3Y-6n4S1vjAXyrhapa50MRq-jjxWlHbpCku2eP8892~EZ9XvPBnInAAXDIJ9LWElra9zyr0Jj2dNAO3dYDiP5gg5ULlHel8DFDfLajhcWcg5TDQLW4kKrnoz2xgVKBs-E8MTkdfgBshotnQ__&Key-Pair-Id=APKAIE5G5CRDK6RD3PGA)
The Klebsiella pneumoniae Sap (sensitivity to antimicrobial peptides) transporter contributes to the in vivo virulence of K. pneumoniae during gastrointestinal infection. A, Mouse survival analysis. Female BALB/cByl mice (8 per group) were infected intragastrically with K. pneumoniae Ca0437 wild type (WT), sapA, or ΔsapA+sapABC at a dose of 4 × 108 colony-forming units (CFUs) per mouse. Survival of mice was monitored for 4 weeks. B, In vivo bacterial loads in liver and spleen. WT, ΔsapA or ΔsapA+sapABC was administered intragastrically to female BALB/cByl mice (4 per group) at an inoculation dose of either 4 × 108 CFUs for 24 hours or 1 × 108 CFUs for 72 hours. Bacterial levels in liver and spleen were determined at 24 (right) or 72 (left) hours after infection. Bacterial numbers (expressed as log10 CFUs) were standardized per 0.1 g of wet organ weight. C, In vivo competition assays. Either test strain (WT, ΔsapA, or ΔsapA+sapABC) was compared with the ΔplacZ mutant strain in female BALB/cByl mice (8 per group) by intragastric inoculation with 1 × 108 CFUs per strain per mouse. After bacterial recovery on isopropyl β-D-1-thiogalactopyranoside (IPTG)/X-Gal plates, the ratio of LacZ-positive (blue) to LacZ-negative (white) colonies in the liver or spleen of each mouse was determined. The competitive index was defined as (ΔplacZ output/test strain output)/(ΔplacZ input/test strain input). Each symbol represents the index for each inoculum, and bars represent medians. D, Intestinal colonization in vivo. K. pneumoniae were administered intragastrically to each of female BALB/cByl mice (4 per group) at an inoculation dose of 4 × 108 CFUs. Bacterial numbers (expressed as log10 CFUs) in the colorectum were determined 72 hours after infection. *P < .05; †P < .01; ‡ P < .001 (log-rank test [A] or analysis of variance [B–D] with Bonferroni multiple comparisons test). Abbreviation: NS, not significant.
We examined bacterial loads in the infected mice (Figure 5B). Compared with those in WT-infected mice, recovered colony counts in the ΔsapA-infected mice were significantly reduced in both liver and spleen, suggesting that the bacterial ability to survive and spread in the host was attenuated. Similar results were observed in male mice (Supplementary Figure 5). In addition, in vivo competition assays showed that the ΔsapA mutant had a lower competitive index (ΔsapA/ΔplacZ) than the WT strain (WT/ΔplacZ) (Figure 5C). To clarify the role of the Sap transporter in intestinal colonization in vivo, we quantified K. pneumoniae in the colorectum of infected mice (Figure 5D). Compared with the WT strain, intestinal colonization was significantly decreased in the ΔsapA mutant. These data indicate that the K. pneumoniae Sap transporter mediates in vivo colonization and survival. Gene complementation restored the deficiency of ΔsapA in vivo (Figure 5B–5D, ΔsapA+sapABC).
Histopathological evaluation of the livers from Ca0437 WT–infected mice at 72 hours after infection revealed the frequent presence of either macroabscesses (Figure 6Aupper left) or microabscesses (Figure 6Aupper middle and right). In contrast, only scattered microabscesses were observed in ΔsapA-infected mice (Figure 6Alower left). The numbers of liver abscesses were 13, 23, 49, 27 (mean [SEM], 28 [15.2]) in 4 WT-infected mice, and 0, 7, 0, 14 (5.3 [6.7]) in 4 ΔsapA-infected mice (WT vs ΔsapA, P = .04; Student t test). Therefore, the liver abscess numbers induced by ΔsapA were significantly decreased. The histopathological appearance of the colon was unremarkable in these 2 groups of mice 72 hours after infection (Figure 6Alower middle and right). Similar results were seen with liver histopathology 120 hours after infection (Figure 6B). In WT-infected mice, gross liver abscesses were observed (Figure 6Bleft); microscopically, macroabscesses and microabscesses with rupture causing peritonitis were noted (Figure 6Bmiddle). In contrast, fewer microabscesses were identified in ΔsapA-infected mice, and the liver capsule was intact without peritonitis (Figure 6Bright). In conclusion, the Sap transporter promotes K. pneumoniae induction of liver abscess.
![Histopathological examination of tissues from mice infected with Klebsiella pneumoniae–infected mice. BALB/cByl mice were infected intragastrically with K. pneumoniae Ca0437 wild type (WT) or the isogenic ΔsapA mutant (ΔsapA). Representative micrographs of hematoxylin-eosin–stained sections from the liver or colon retrieved at 72 or 120 hours after infection are shown. A, Findings 72 hours after infection (1 × 108 colony-forming units [CFUs] per mouse). In WT-infected mice, macroabscesses (upper left panel, arrow) and/or microabscesses (upper middle, arrow) were noted in the liver (original magnification, ×40); the high-power field (magnification, ×400) shows the microabscesses composed of neutrophils and lymphocytes (upper right). In the liver of ΔsapA-infected mice (lower left), only a few microabscesses were identified. The colon tissues of WT-infected mice (lower middle) and ΔsapA-infected mice (lower right) were both unremarkable under light microscopy. B, Findings 120 hours after infection (6 × 108 CFUs). In the WT-infected mice, the resected liver revealed grossly visible abscesses (left panel, arrow); microscopically, macroabscesses and microabscesses with rupture causing peritonitis were noted (middle). In the liver of ΔsapA-infected mice (left), only a few microabscesses were identified, and the liver capsule was intact without peritonitis. (All magnifications are original magnifications.)](https://oup.silverchair-cdn.com/oup/backfile/Content_public/Journal/jid/219/8/10.1093_infdis_jiy615/3/m_jiy61506.jpeg?Expires=1749814104&Signature=f~oazMlduBExGtc9LhEdnmH1S4RdzJrfZhtPIBQweFcQXCxkLkyCFCWeY5ZZqLVE5-AHog80M~AEpsyoBlxqwtdp-nZtaK5NL47vQT3471lebeILAtBFNfnds8ASHPCiop~ZM34wpe7mwFH0ermEWVPR64jG7-mDaJucPO~aGjXP9THpsKwb~zpSwrFLeV2o2dO5OKAkpW5sB6u4RqU0P2f6sT8426okgzLnzx7tUMMNha8gGf4z4D1xeSRg5gPvxzQFddgzuraN7DlmsmvGl7Q3VsYfZLcsGV6d0AAk5QlH5oOiywnSlAE3qCoC3gzEu4HEg0UL8bsZomwLouRR8w__&Key-Pair-Id=APKAIE5G5CRDK6RD3PGA)
Histopathological examination of tissues from mice infected with Klebsiella pneumoniae–infected mice. BALB/cByl mice were infected intragastrically with K. pneumoniae Ca0437 wild type (WT) or the isogenic ΔsapA mutant (ΔsapA). Representative micrographs of hematoxylin-eosin–stained sections from the liver or colon retrieved at 72 or 120 hours after infection are shown. A, Findings 72 hours after infection (1 × 108 colony-forming units [CFUs] per mouse). In WT-infected mice, macroabscesses (upper left panel, arrow) and/or microabscesses (upper middle, arrow) were noted in the liver (original magnification, ×40); the high-power field (magnification, ×400) shows the microabscesses composed of neutrophils and lymphocytes (upper right). In the liver of ΔsapA-infected mice (lower left), only a few microabscesses were identified. The colon tissues of WT-infected mice (lower middle) and ΔsapA-infected mice (lower right) were both unremarkable under light microscopy. B, Findings 120 hours after infection (6 × 108 CFUs). In the WT-infected mice, the resected liver revealed grossly visible abscesses (left panel, arrow); microscopically, macroabscesses and microabscesses with rupture causing peritonitis were noted (middle). In the liver of ΔsapA-infected mice (left), only a few microabscesses were identified, and the liver capsule was intact without peritonitis. (All magnifications are original magnifications.)
DISCUSSION
Results of epidemiological studies suggest that many K. pneumoniae infections, including bacteremia and PLA, may originate from gastrointestinal reservoirs [11–15]. However, it remains largely unknown how K. pneumoniae interacts with host intestinal epithelium. The present study demonstrated that a bacteremia-causing isolate induced liver abscess in a mouse gastrointestinal infection model. We revealed a novel role for the K. pneumoniae Sap transporter in bacterial–intestinal cell interactions and virulence, suggesting that K. pneumoniae–intestinal interplay contributes to pathogenesis.
The K. pneumoniae Sap transporter functions in interactions with the host cells. In agreement with our findings, a study of nontypeable H. influenzae showed that a sapA mutant was less adherent and invasive to cultured bronchial and middle ear epithelial cells [38]. We observed that the K. pneumoniae Sap transporter was required for adherence to human liver cells and a variety of epithelial cells, including intestinal, lung, and urinary bladder epithelial cells. The Sap transporter also contributed to K. pneumoniae translocation across the intestinal epithelium. It also promoted K. pneumoniae–macrophage interactions and host secretion of proinflammatory cytokines. Classically, the Sap transporter was identified based on AMP resistance in several gram-negative bacteria [17–24] and heme acquisition in H. influenzae [25]. In K. pneumoniae, we observed involvement of the Sap transporter in bacterial resistance against AMP LL-37 but not in heme iron use. Whether the K. pneumoniae Sap transporter mediates acquisition of other iron sources needs to be further assessed. It is involved in multiple functions relating to pathogenesis, including (at minimum) AMP resistance, epithelial cell attachment and translocation, macrophage interactions, and induction of host innate responses. This may serve as a target for drug design or vaccine development.
Using a murine intragastric infection model, we demonstrated that the Sap transporter–mediated K. pneumoniae virulence. Consistent with our findings, the Sap transporter has been implicated in H. influenzae virulence [20]. In a chinchilla otitis media model, a ΔsapA mutant of nontypeable H. influenzae was significantly attenuated in its ability to survive in the nasopharynx and the middle ear. Our data demonstrated that K. pneumoniae ΔsapA was impaired in the ability to induce mouse death and maintain bacterial loads in liver, spleen, and intestine. Although ΔsapA is more resistant to macrophage recognition and killing, its abilities to adhere to and translocate across the intestinal epithelium and to resist AMP are attenuated. Overall in vivo virulence is reduced. Interactions with intestinal epithelium and AMP, which could happen in the earlier stage of infection, seem to be important for K. pneumoniae pathogenesis. Histopathological examination revealed that ΔsapA exhibited attenuated the ability to induce liver abscess. These data indicate that the Sap transporter is essential for K. pneumoniae to colonize, survive, and spread in the host, which contributes to systemic infection, liver abscess generation, and host death.
The molecular mechanisms whereby the K. pneumoniae Sap transporter contributes to cell adherence and cell interactions remain unclear. Our results revealed that Sap transporter promotes expression of genes encoding type I fimbriae. Thus, its effects on adherence may reflect changes in the production of other K. pneumoniae adhesive molecules. To our knowledge, the present work is the first study to demonstrate that a Sap transporter affects the transcription levels of other genes, suggesting a novel role for it in the regulation of gene expression. Type I fimbria is an adhesin mediating the adherence of K. pneumoniae to ciliated tracheal cells in vitro [36] and a virulence factor in a mouse model of K. pneumoniae urinary tract infection [39, 40]. However, type I fimbriae do not affect the ability of K. pneumoniae to colonize the intestine or the lung in vivo [39]. Different from type I fimbriae, the Sap transporter contributed to gastrointestinal colonization and infection in vivo. We hypothesize that it may affect the production of other factors (other than type I fimbriae) involved in bacterial interactions with intestinal cells. Further investigations will be needed to identify K. pneumoniae genes influenced by the Sap transporter and to elucidate potential cross-talk or regulatory networks among the affected factors.
This study has some limitations. First, we used the human and mouse cell lines; primary cells were not used to confirm the results. Second, in vivo translocation of K. pneumoniae in intestine was not directly demonstrated. Third, the mutation of sapA seemed to be polar. The phenotype could be attributed to sapA, sapB, or sapC. Fourth, we did not use the differentiated Caco-2 monolayers for library screening or the pulmonary and urinary epithelial cells that generate TJs to mimic barrier functions for the adherence assays. The factors involved in the interactions with differentiated epithelium could be neglected.
In summary, we demonstrated in the current study, for the first time, that the Sap transporter contributes to K. pneumoniae pathogenesis. Our data showed that the K. pneumoniae Sap transporter mediates bacterial–host cell interactions, epithelial colonization, and virulence. This study offers new insights into the interactions of K. pneumoniae with host gastrointestinal cells and suggests possible mechanisms whereby gastrointestinal K. pneumoniae could escape the gut to cause systemic infection.
Supplementary Data
Supplementary materials are available at The Journal of Infectious Diseases online. Consisting of data provided by the authors to benefit the reader, the posted materials are not copyedited and are the sole responsibility of the authors, so questions or comments should be addressed to the corresponding author.
Notes
Acknowledgments. We thank Hung Hung, PhD and Hsien-Ho Lin, MD, ScD (both from the Institute of Epidemiology and Preventive Medicine, National Taiwan University) for advice and assistance with statistical analysis. We also thank Dai-Yun Du (from the I-Shou University) for assistance with AMP experiments and the Biomedical Engineering and Biotechnology Center of the I-Shou University for assistance with confocal microscopy and 3-dimensional image analysis.
Financial support. This study was supported by the Ministry of Science and Technology, National Taiwan University, National Taiwan University Hospital, E-Da Hospital, and the E-Da Hospital-National Taiwan University Hospital Joint Research Program.
Potential conflicts of interest. All authors: No reported conflicts of interest. All authors have submitted the ICMJE Form for Disclosure of Potential Conflicts of Interest. Conflicts that the editors consider relevant to the content of the manuscript have been disclosed.