-
PDF
- Split View
-
Views
-
Cite
Cite
Shun-Mei Lin, A-Yeung Jang, Yong Zhi, Shuang Gao, Sangyong Lim, Jae Hyang Lim, Joon Young Song, Paul M Sullam, Joon Haeng Rhee, Ho Seong Seo, Vaccination With a Latch Peptide Provides Serotype-Independent Protection Against Group B Streptococcus Infection in Mice, The Journal of Infectious Diseases, Volume 217, Issue 1, 1 January 2018, Pages 93–102, https://doi.org/10.1093/infdis/jix565
- Share Icon Share
Abstract
Streptococcus agalactiae (group B streptococcus [GBS]) is a leading cause of invasive diseases in neonates and severe infections in elderly individuals. GBS serine-rich repeat glycoprotein 1 (Srr1) acts as a critical virulence factor by facilitating GBS invasion into the central nervous system through interaction with the fibrinogen Aα chain. This study revealed that srr1 is highly conserved, with 86.7% of GBS clinical isolates expressing the protein. Vaccination of mice with different Srr1 truncated peptides revealed that only Srr1 truncates containing the latch domain protected against GBS meningitis. Furthermore, the latch peptide alone was immunogenic and elicited protective antibodies, which efficiently enhanced antibody-mediated opsonophagocytic killing of GBS by HL60 cells and provided heterogeneous protection against 4 different GBS serogroups. Taken together, these findings indicated that the latch domain of Srr1 may constitute an effective peptide vaccine candidate for GBS.
Streptococcus agalactiae (group B streptococcus [GBS]) remains a leading cause of sepsis and meningitis in infants [1]. Recent reports indicate that it has also emerged as a frequent cause of invasive infections in adults with underlying medical conditions and in pregnant women [2, 3]. The only available treatment against GBS infections in infants is intrapartum antibiotic prophylaxis, which has been effective in reducing early onset diseases caused by GBS [4]. However, this life-threatening infection in both neonates and elderly individuals continues to result in a high incidence of morbidity and mortality despite use of antibiotic therapy [5]. Therefore, there is an urgent need for the development of a vaccine against GBS for both neonates and adults [6].
Among various vaccine candidates, GBS capsular polysaccharide–protein conjugate vaccine has shown the most promising results to date in clinical studies [7, 8]. Phase 1 and 2 clinical studies with trivalent (Ia, Ib, and III) conjugate vaccine demonstrated serotype-specific antibody responses in >75% of vaccinated pregnant adults [7]. However, although multivalent polysaccharide–protein conjugate vaccines have demonstrated immunogenicity and safety, there have been several concerns regarding the use of this class of vaccines, such as immune interference with similar types of conjugate vaccines, potential problems involving serotype replacement, and reduced efficacy owing to the diverse serotype distribution across and within geographic regions [9–11]. Thus, structurally conserved protein antigens that are essential for GBS virulence and able to confer broad protection against heterologous GBS serogroups are emerging as the most attractive and cost-effective vaccine candidates [12, 13]. In this regard, certain surface proteins of GBS are currently under preclinical investigation as broad-spectrum vaccines [14–18].
In particular, GBS express either one of two allelic serine-rich repeat (SRR) glycoproteins, Srr1 and Srr2, which are members of a large and diverse family of cell wall–anchored adhesins of gram-positive bacteria [19]. The SRR proteins have a highly conserved domain organization that includes a secretion signal sequence, 2 SRR domains that undergo glycosylation, a specialized binding domain between 2 SRR domains, and an LPxTG motif. The binding domain of SRR glycoprotein facilitates bacterial adherence to the host cells [19, 20]. Both Srr1 and Srr2 of GBS can bind the fibrinogen Aα chain through the dock, lock, and latch mechanism. Upon binding, the C-terminal ends of the glycoproteins, composed of a 13–amino acid domain termed “latch,” undergo a conformational change to lock the ligand by forming an intramolecular complementation [21]. Srr2 of GBS strain COH1 was identified as a promising vaccine candidate by a large-scale surface proteomic-based approach [16]. Although our previous studies found that both Srr1 and Srr2 are critical virulence factors in the pathogenesis of meningitis and endocarditis due to GBS [21–24], the use of Srr1 in a vaccine has not yet been investigated. In the present study, we evaluate the efficacy of vaccination with the latch domain of Srr1 in protecting mice against GBS meningitis and examine the mechanism of action of the elicited latch-specific antibodies by assessing GBS binding to fibrinogen and antibody-mediated opsonophagocytic killing of GBS.
MATERIALS AND METHODS
Reagents
Purified human fibrinogen was from Hematologic Technologies (Essex Junction, VT). Synthetic latch peptide was generated by Peptron (Daejeon, Republic of Korea). All other reagents used in this study were from Sigma-Aldrich (St. Louis, MO).
Bacterial Strains and Growth Conditions
The bacteria used in this study are listed in Supplementary Table 1.
Cloning and Expression of Truncated Srr1 Proteins
Plasmid vectors and polymerase chain reaction (PCR) primers are listed in Supplementary Tables 1 and 2. PCR products from GBS CNCTC10/84 genomic DNA were cloned into pET28a to express 6×His tagged N2N3 (amino acids 303–641), N2 (amino acids 303–467), N3 (amino acids 468–641), or the latch deletion variant N2N3∆latch (amino acids 303–628). Proteins were purified by Ni-NTA agarose (Promega; Madison, WI) according to the manufacturer’s instructions. The endotoxin levels in the purified proteins were <0.5 EU/mg, as assessed using the Limulus Amebocyte Lysate kit (Sigma-Aldrich).
GBS Capsular Serotyping
Isolation of GBS from patients was approved by the Institutional Review Board at Korea University Hospital (protocol KUGH14106), and written informed consent was obtained from all participants. Capsular polysaccharide serotypes were identified by latex agglutination, using a Strep-B-Latex kit (SSI Diagnostica; Hillerod, Denmark).
Multiplex PCR Typing of srr1 and srr2
Bacterial genomic DNA was isolated from the GBS clinical isolates, using Wizard Genomic DNA purification kits (Promega). Multiplex PCR was performed with1 μg of genomic DNA and 10 pmol srr1- and srr2-specific PCR primers, using 2×Taq PCR premix (Enzynomics; Daejeon; Supplementary Table 2). PCR products were visualized on 1% agarose gels stained with RedSafe Nucleic Acid Staining Solution (Intron; SungNam, Republic of Korea).
Animal Experiments
All animal experiments conducted in this study were approved by the Committee on the Use and Care of Animals at the Korea Atomic Energy Research Institute. Male CD-1 mice (4 weeks old) were purchased from Orient Bio (Suwon, Republic of Korea). Mice were intraperitoneally vaccinated twice at 14-day intervals with 100 μL of recombinant Srr1 truncates (10 μg) or bovine serum albumin (BSA)–conjugated latch (BSA-latch; 1 to 25 μg) with 2% aluminum hydroxide gel (Invivogen; San Diego, CA). At 7 days after the second vaccination, blood was collected and antibodies specific to N2N3 or latch were measured by ELISA [25]. Protective effects of Srr1 vaccination against GBS infection were investigated in a mouse model as follows [23]. Seven days after the second vaccination, mice were intravenously infected with 50 μL of GBS (1 × 107 colony-forming units [CFU]), and their survival was monitored for 72 hours. Blood specimens and the brain, spleen, and kidneys were collected at the time of death or at the end of the experiments, for GBS counting. Tissues were homogenized by plunging in 1.5 mL of phosphate-buffered saline through a 40-μm mesh strainer [25], and bacteria numbers were counted by culturing serially diluted blood specimens and tissue homogenates on blood agar plates. For histological study, formalin-fixed brain tissue specimens were embedded in paraffin and stained with hematoxylin and eosin (Sigma-Aldrich). GBS in brain tissue specimens was detected by Gram stain (Sigma-Aldrich).
GBS Invasion Assay
The human brain microvascular endothelial cell line (hBMEC) was obtained from Lonza (Walkersville, MD), and a bacterial invasion assay was performed as described previously [26]. Briefly, GBS was grown to mid-log phase and then added to confluent hBMEC monolayers at a multiplicity of infection of 0.1 (1 × 104 CFU). Thirty minutes after infection, monolayers were treated with penicillin (100 μg/mL) for 30 minutes to remove extracellular GBS, and cells were lysed with 0.1% Triton X-100. Cell lysates were plated on blood agar plates to enumerate the intracellular bacteria. To determine the immune sera inhibitory effects on GBS invasion, 400 μL of GBS (2.5 × 105 CFU) was preincubated with 100 μL of mouse immune serum for 30 minutes at room temperature, and 20 μL of GBS (1 × 104 CFU) was added to the cells [22]. GBS invasion was calculated as [(recovered CFU)/(initial inoculum CFU)] × 100%.
GBS Binding to Immobilized Fibrinogen
GBS binding capacity to the fibrinogen was measured as described previously [21]. Purified fibrinogen (1 μg/mL) was immobilized in 96-well microtiter plates (SPL; Seoul, Republic of Korea). Then, 90 µL GBS (109 CFU/well) was preincubated with 10 µL of immune sera for 30 minutes and added onto a fibrinogen-coated plate. After incubation for 30 minutes at 37°C, wells were washed to remove unbound bacteria and stained with crystal violet (0.5% v/v).
Adoptive Transfer of Sera or CD4+ T Cells
Spleen cell suspensions were made by passing spleen specimens through a nylon cell strainer (BD; La Jolla, CA), and red blood cells were lysed with FACS Lysing Solution (BD). CD4+ T cells were isolated using CD4+ T-cell isolation affinity columns (Miltenyi Biotech; Bergisch Gladbach, Germany). CD4+ T cells (4 × 105 cells/mice) or sera (100 μL) were administered intraperitoneally to naive CD-1 mice. Twelve hours after serum or cell administration, mice were intravenously infected with GBS, and survival was monitored for 72 hours.
Splenocyte Proliferation
Splenocytes (1 × 105 cells/well) were plated onto round-bottomed 96-well plates (SPL) and incubated with phosphate-buffered saline, concanavalin A (1 μg/well), or latch peptide for 72 hours, and splenocyte proliferation was determined by the Cell Counting Kit-8 (Dojindo Technologies; Rockville, MD).
Flow Cytometry
Intracellular cytokine staining of CD4+ T cells was performed as described previously [27]. Splenocytes (1 × 106) were stimulated with 10 μg of latch peptide for 3 hours, and intracellular protein transportation was blocked with GolgiPlug (BD). Cell surface Fc receptors were blocked using Fc Block (BD) for 30 minutes at 4°C, and then cells were stained with surface markers (FITC-αCD3 and PE-αCD4), fixed, and permeabilized with Cytofix/Cytoperm solution (BD) for 30 minutes at 4°C followed by intracellular cytokine staining using PerCP-α–interferon γ (IFN-γ). All samples were run on a BD FACSVerse flow cytometer, and results were analyzed with FlowJo software (Ashland, OR).
Cytokine Measurement
The culture supernatants of splenocytes prepared as described above were collected, and interleukin 5, IFN-γ, interleukin 2, and interleukin 6 levels were measured with enzyme immunoassay kits (BD).
Data Analysis
Data are expressed as mean values ± standard deviations. Data in the bar graphs and bacterial numbers between groups were compared by unpaired t tests. Survival of mice was analyzed by Kaplan-Meier analysis, and the statistical significance of the difference was tested by the log-rank test in GraphPad Prism, version 6.0. Differences with P values of <.05 were considered significant.
RESULTS
Epidemiological Analysis of Srr1 and Srr2 Expression in GBS Clinical Isolates
A total of 165 clinical isolates of GBS were collected between January 2013 and January 2014 at Korea University Hospital (Seoul). Capsular serotyping of GBS clinical isolates showed 14 isolates of serotype Ia, 30 of Ib, 6 of II, 39 of III, 2 of IV, 20 of V, 15 of VI, 13 of VIII, and 1 of IX. Twenty-five isolates were either nontypeable (14 isolates) or multitypeable (11 isolates; Supplementary Table 3). PCR analysis of srr1 or srr2 expression found that 86.7% of GBS isolates (143) expressed srr1 whereas only 1.2% (2) expressed srr2. Notably, 12.1% of isolates (20) did not express either srr1 or srr2.
Srr1-N2N3 Domain Latch Sequence Is a Critically Important Epitope for Protection Against GBS Infection
We previously reported that the binding region of Srr1 (Srr1-N2N3) acts as a critical domain in the pathogenesis of GBS infection by interacting with human fibrinogen on the brain microvascular endothelial cell surface. Based on the clinical importance of GBS binding to fibrinogen, we investigated whether this domain might be used as a vaccine against GBS infections. Several truncated forms of Srr1-N2N3 were developed as shown in Supplementary Figure 1, and their immunogenicity was tested. As shown in Figure 1A, all mouse groups vaccinated with either N2N3, N2, N3, or N2N3Δlatch showed significantly higher levels of N2N3-specific immunoglobulin G (IgG) in serum as compared to mice vaccinated with adjuvant. N2N3-specific immunoglobulin M (IgM) serum levels were also tested; however, no significant difference was seen as compared to the adjuvant group (Supplementary Figure 2A). Moreover, no differences were observed in N2N3-specific IgG isotype levels between protein-vaccinated groups (Supplementary Figure 2B–E). These findings indicate that all of the N2N3 truncates used in this study are similarly immunogenic in mice.
![Protective effects of vaccination with Srr1 truncates against group B streptococcus (GBS). Mice (n = 10) were vaccinated with Srr1 truncates N2N3, N2, N3, and N2N3∆latch, with 2% Alhydrogel adjuvant. A, Serum levels of immunoglobulin G (IgG) were measured 7 days after the second vaccination. B, Seven days after the second vaccination, mice were intravenously challenged with GBS CNCTC10/84 (107 colony-forming units [CFU]), and mouse survival was recorded. **P < .01 and ***P < .001, compared with mice vaccinated with adjuvant. C, Brains of mice (n = 5) were dissected at the time of death or upon euthanasia at the end of experiment and homogenized in phosphate-buffered saline. GBS counts were measured in the serially diluted brain tissue homogenates. ***P < .001, compared with mice vaccinated with adjuvant. D, Human brain microvascular endothelial cell line monolayers were infected with GBS at a multiplicity of infection of 0.1 for 30 minutes, followed by washing and incubation for 30 minutes with penicillin (100 μg/mL). Cells were then lysed with 0.1% Triton X-100 and plated onto blood agar plates to count GBS. GBS organisms were preincubated for 30 minutes with immune serum from mice vaccinated with adjuvant, N2N3, N2, N3, or N2N3∆latch before cell infection. Data are expressed as means ± SD. ***P < .001, compared with cells incubated with adjuvant-vaccinated serum.](https://oup.silverchair-cdn.com/oup/backfile/Content_public/Journal/jid/217/1/10.1093_infdis_jix565/5/m_jix56501.jpeg?Expires=1749934531&Signature=aBT7fHMAnmiJJHwXqtX6GhrX2wNQD-GX4QPI7ryXGZhblLnN-b1mxB~zOsNJpB3~vC71eryy~k3~K-5gT-s324onHFVOTFszBZ6RHwRorO4PkS6eLEbHhLvfD~K6ipwnCxH3gW5uHWGJB4pCw45-81N4yAUm2yjwmMF4C70X0GTyOGcXM3Y20A0NztEjG0DaE5KDoNnHs4bLJ3EQzbiF3FAnxmXn4PVZ22de8-F-Zjhs78m8B34BU-4TXIlPUMaynO83tvF8bTLVgv8B~ApS6C6D3yIlBqgmrINxtlBJdP3q61yS1PsPXm8ldAxNH5tGysXKkI7ztvLiZB1n86KnSA__&Key-Pair-Id=APKAIE5G5CRDK6RD3PGA)
Protective effects of vaccination with Srr1 truncates against group B streptococcus (GBS). Mice (n = 10) were vaccinated with Srr1 truncates N2N3, N2, N3, and N2N3∆latch, with 2% Alhydrogel adjuvant. A, Serum levels of immunoglobulin G (IgG) were measured 7 days after the second vaccination. B, Seven days after the second vaccination, mice were intravenously challenged with GBS CNCTC10/84 (107 colony-forming units [CFU]), and mouse survival was recorded. **P < .01 and ***P < .001, compared with mice vaccinated with adjuvant. C, Brains of mice (n = 5) were dissected at the time of death or upon euthanasia at the end of experiment and homogenized in phosphate-buffered saline. GBS counts were measured in the serially diluted brain tissue homogenates. ***P < .001, compared with mice vaccinated with adjuvant. D, Human brain microvascular endothelial cell line monolayers were infected with GBS at a multiplicity of infection of 0.1 for 30 minutes, followed by washing and incubation for 30 minutes with penicillin (100 μg/mL). Cells were then lysed with 0.1% Triton X-100 and plated onto blood agar plates to count GBS. GBS organisms were preincubated for 30 minutes with immune serum from mice vaccinated with adjuvant, N2N3, N2, N3, or N2N3∆latch before cell infection. Data are expressed as means ± SD. ***P < .001, compared with cells incubated with adjuvant-vaccinated serum.
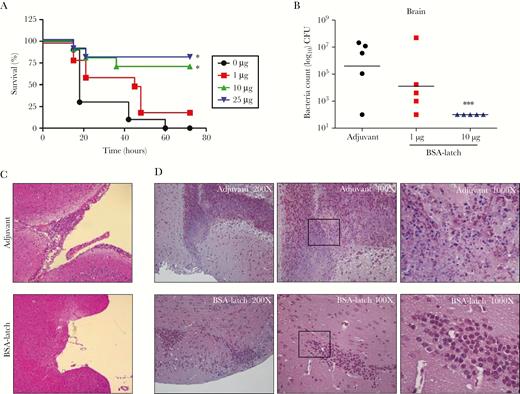
Protective effects of latch vaccination against group B streptococcus (GBS) infections in mice. Mice (n = 5) were vaccinated with bovine serum albumin–conjugated latch peptide (BSA-latch) twice at 14-day intervals, followed by challenge intravenously with 107 colony-forming units of GBS CNCTC10/84. A, Survival of mice was recorded for 3 days. B–D, Brains of mice were dissected at the time of death or after euthanasia at the end of the experiment. Brain tissue specimens were homogenized in phosphate-buffered saline, and GBS counts were measured in the serially diluted brain tissue homogenates (B). Formalin-fixed, paraffin-embedded brain tissue sections were stained with hematoxylin and eosin (C) and Gram stain (D). *P < .05 and ***P < .001, compared with adjuvant vaccinated mice.
We next tested whether the N2N3-specific antibodies induced by different Srr1 truncates were functionally effective against GBS infection. Mice vaccinated with different Srr1 truncates were intravenously challenged with GBS strain CNCTC10/84 (serotype V), and their survival was monitored. All of the mice vaccinated with adjuvant died by 36 hours after GBS infection (Figure 1B). However, >80% of mice vaccinated with either N2N3 or N3 survived through the end of the experiment. Notably, despite similar N2N3-specific IgG serum levels, vaccination afforded no protective effect in mice vaccinated with N2 or N2N3Δlatch. Moreover, GBS numbers in brain specimens were significantly decreased in mice vaccinated with either N2N3 or N3 as compared to those vaccinated with N2 or N2N3Δlatch (Figure 1C).
Because Srr1 is necessary for GBS invasion into the brain endothelium [21–23], we next tested whether antibodies raised by each Srr1 truncate could block GBS invasion into hBMECs. As shown in Figure 1D, GBS pretreatment with sera from the mice vaccinated with N2N3 or N3 significantly inhibited GBS invasion into hBMECs, whereas sera from the mice vaccinated with adjuvant, N2, or N2N3∆latch had no effect. Taken together, these data strongly suggest that Srr1-N2N3 is highly immunogenic and that the Srr1 latch sequence constitutes a critically important epitope required for the protective immune responses against GBS infection and invasion into the brain.
Vaccination With Latch Peptide Elicits Protective Immune Responses Against GBS Infection
We next examined whether vaccination with latch peptide could protect against GBS infection. Mice were vaccinated intraperitoneally with BSA-latch, followed by intravenous challenge with GBS. As shown in Figure 2A, vaccination with 10 or 25 μg of BSA-latch protected mice from death following GBS infection. In addition, vaccination with BSA-latch significantly inhibited the bacterial load in the blood, brain, kidneys, and spleen (Figure 2B and Supplementary Figure 3). Consistent with the survival and microbiological assay findings, the brains of mice vaccinated with adjuvant showed pathological changes characteristic of meningitis, including serious meningeal thickening, severe tissue destruction, neutrophil infiltration, and large areas of cerebral necrosis, following GBS infection. Conversely, no obvious pathological alterations were found in BSA-latch recipients following GBS infection (Figure 2C). Furthermore, numerous sites of dark purple–stained GBS were found in Gram-stained brain tissues from mice that were vaccinated with adjuvant and infected with GBS, whereas vaccination with BSA-latch greatly reduced the number of GBS-positive brain tissue sites (Figure 2D). These results showed that vaccination with latch could provide protection against GBS meningitis in mice.
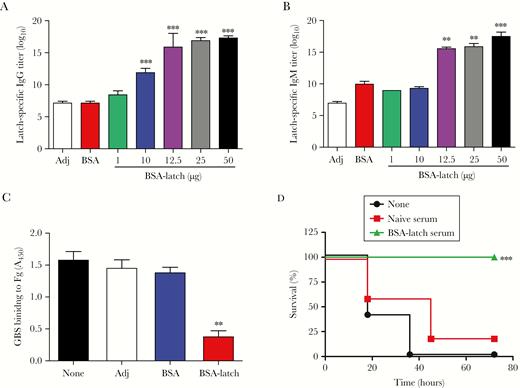
Protective effects of latch-specific antibodies against group B streptococcus (GBS). Mice (n = 5) were vaccinated with indicated concentrations of bovine serum albumin–conjugated latch peptide (BSA-latch) twice intraperitoneally at 14-day intervals. A and B, Serum levels of latch-specific immunoglobulin G (IgG; A) and immunoglobulin M (IgM; B) were analyzed 7 days after the second vaccination. Data are expressed as means ± SD. **P < .01 and ***P < .001, compared with adjuvant (Adj). C, GBS CNCTC 10/84 (109 colony-forming units) was incubated with 10 μL of immune serum from the mice vaccinated with Adj, BSA, or BSA-latch and added to the well coated with human fibrinogen. After incubation for 30 minutes, wells were washed and stained with 0.5% crystal violet. Data are expressed as means ± SD. **P < .01, compared with Adj. D, Serum (100 μL) from naive or BSA-latch–vaccinated mice was intraperitoneally inoculated into naive CD-1 mice (n = 5). At 12 hours after inoculation, mice were challenged intravenously with GBS CNCTC 10/84, and mouse survival was monitored for 72 hours. *P < .05, compared with unvaccinated mice.
To characterize the immune responses driven by latch vaccination, 5 mice were vaccinated intraperitoneally with BSA-latch, and levels of latch-specific IgG and IgM antibodies were measured 7 days after vaccination. As shown in Figure 3A and 3B, BSA-latch vaccination significantly enhanced IgG and IgM serum levels in a dose-dependent manner. Furthermore, levels of all IgG isotypes were significantly increased in the mice vaccinated with BSA-latch (Supplementary Figure 4A–D), and relative analysis showed that IgG1 (average titer, 158400) was the most predominant isotype, whereas levels of IgG2a (average titer, 15200) and IgG3 (average titer, 5900) were relatively lower, indicating that the Th2 response was greater than the Th1 response.
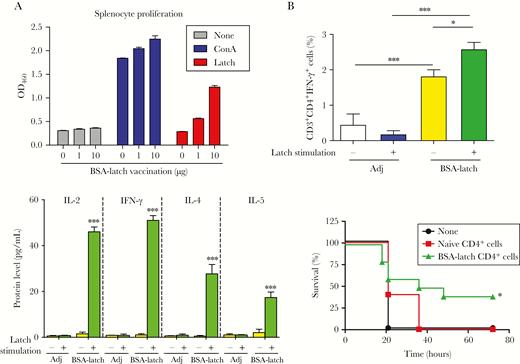
Protective effect of latch-specific CD4+ T cells against group B streptococcus (GBS). Mice (n = 5) were vaccinated with 10 µg of bovine serum albumin–conjugated latch peptide (BSA-latch) twice intraperitoneally at 14-day intervals, and the spleen was dissected from the mice 7 days after vaccination. A, Splenocytes were incubated with 1 or 10 µg of latch peptide for 48 hours, and proliferation of splenocytes was measured. Data are expressed as means ± SD. B, Splenocytes were incubated with 10 µg of latch peptide, and percentages of interferon γ (IFN-γ)–secreting CD4+ T cells were measured by flow cytometry. Data are expressed as means ± SD. C, Splenocytes were incubated with 10 µg of latch peptide, and expression of interleukin 2 (IL-2), interleukin 4 (IL-4), interleukin 5 (IL-5), and IFN-γ were measured in culture supernatants by enzyme-linked immunosorbent assay. Data are expressed as means ± SD. D, Splenic CD4+ T cells from naive mice (n = 5) or BSA-latch–vaccinated mice were pooled and transferred intraperitoneally to CD-1 mice (n = 5). At 12 hours after inoculation, mice were challenged intravenously with GBS CNCTC10/84, and mouse survival was monitored for 72 hours. *P < .05, **P < .01, and ***P < .001, compared with unvaccinated mice. Adj, adjuvant; ConA, concanavalin A.
To examine the specificity of latch-specific IgG, purified N2N3 and N2N3∆latch proteins were analyzed by Western blotting, using mouse anti-N2N3 or anti-latch serum (Supplementary Figure 5). Mouse anti-N2N3 IgG reacted strongly with both N2N3 and N2N3∆latch protein, whereas mouse anti-latch IgG reacted strongly with N2N3 but at much lower levels with N2N3∆latch protein. This finding confirmed the specificity of mouse anti-latch antibody to the latch sequence in Srr1.
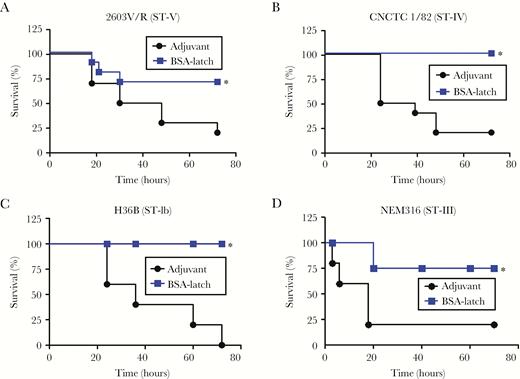
Cross-serotype protection against Srr1-expressing group B streptococcus (GBS) serotypes by latch vaccination. Mice (n = 5) were vaccinated intraperitoneally with 10 µg of bovine serum albumin–conjugated latch (BSA-latch) twice at 14-day intervals, and mice were challenged intravenously with GBS strains 2603V/R (ST-V; A), CNCTC1/82 (ST-IV; B), H36B (ST-1b; C), or NEM316 (ST-III; D) 7 days after the second vaccination. Survival of mice was monitored for 72 hours. *P < .05, compared with mice vaccinated with adjuvant.
To examine the functional efficacy of latch-specific antibodies, we first determined whether latch-specific antibodies inhibited GBS binding to fibrinogen. As shown in Figure 3C, GBS binding to immobilized human fibrinogen was significantly inhibited by GBS pretreatment with mouse anti-latch antibodies, whereas no significant reduction was seen in adjuvant or BSA-vaccinated mice antibodies. As opsonophagocytic killing is an important host defense mechanism against GBS, we next measured the functional efficacy of latch-specific antibodies, using the oposonophagocytic killing assay as previously described [28, 29]. Adjuvant or serum from BSA-vaccinated mice showed no significant opsonic indices, whereas serum from BSA-latch–vaccinated mice showed opsonic indices of 315 (against A909, serotype Ia), 595 (against NEM316, serotype III), and 918 (against CNCTC 10/84, serotype V) indicating that 315-, 595-, and 918-fold diluted serum could opsonize 50% of each serotype by HL60 cells, respectively (Supplementary Figure 6).
Next, we tested whether the adoptive transfer of latch-immune serum to naive mice provided protection against GBS infection. As shown in Figure 3D, 80% of mice that received unvaccinated naive serum had died by 48 hours after infection, whereas adoptive transfer of latch-immune serum provided complete protection against GBS infection. Collectively, these data suggested that vaccination with latch peptide protects mice against GBS infection by eliciting latch-specific antibodies that effectively inhibit GBS binding to fibrinogen and induce antibody-mediated opsonophagocytic killing of GBS by phagocytes.
Antigen-Specific CD4+ T-Cell Responses Against Latch Provide Protection Against GBS Infection
Antigen-specific T-cell responses are critical immune responses mediating peptide/protein vaccine-mediated immune responses against extracellular pathogens. To assess the lymphocyte proliferation response to latch peptide antigen, splenocytes isolated from the mice vaccinated with BSA-latch were stimulated ex vivo with latch peptide (1 µg) or concanavalin A (0.1 µg) for 3 days. Stimulation with concanavalin A greatly enhanced splenocyte proliferation regardless of prior vaccination status (Figure 4A). However, stimulation with latch peptide enhanced splenocyte proliferation in a dose-dependent manner only in cells from mice vaccinated with BSA-latch. To analyze the effect of latch vaccination on antigen-specific CD4+ T-cell responses, the proportion of IFN-γ–expressing CD4+ T cells was measured after latch stimulation in splenocytes. As shown in Figure 4B, a significantly higher number of IFN-γ–expressing CD4+ T cells was detected in splenocytes from mice vaccinated with latch than in splenocytes from adjuvant-vaccinated control mice. Moreover, stimulation with latch further enhanced the proportion of IFN-γ–expressing CD4+ T cells in latch-vaccinated splenocytes. Notably, no significant increase in the number of latch-specific CD8+ T cells was found in latch-vaccinated mice, compared with adjuvant-vaccinated control mice (data not shown). In addition, significant increases in both Th1- and Th2-specific cytokines were detected in splenocytes from mice vaccinated with latch as compared to adjuvant-vaccinated control mice. Adoptive transfer of CD4+ T cells isolated from the spleens of latch-vaccinated mice showed significantly higher survival rates following GBS infection than those receiving CD4+ T cells from unvaccinated mice (naive CD4+ T cells; Figure 4D). Taken together, these data provide experimental evidence that latch peptide is highly immunogenic in mice and that vaccination therewith induces high antigen-specific antibody responses and T-cell–mediated effective cellular immune responses against GBS infection.
Vaccination With Latch Peptide Provides Cross-Protection Against Different Capsule Serogroups
Next, we determined whether latch vaccination provides protection against different GBS serotypes other than serotype V (CNCTC10/84). Srr1 expressing 4 different serotypes—Ib (H36B), III (NEM316), IV (CNCTC1/82), and V (2603V/R)—were tested. As shown in Figure 5, BSA-latch vaccination provided protection against all of the serotypes tested. In note, expression of Srr1 in these strains was confirmed by Western blotting (Supplementary Figure 7). In addition, we also tested whether BSA-latch vaccination provides protection against GBS strain COH1 (serotype III), which expresses Srr2 but not Srr1. As shown in Supplementary Figure 8, BSA-latch vaccination had no protective effect against infection with strain COH1.
DISCUSSION
During the past 30 years, considerable progress has been made in the prevention and treatment of GBS-associated disease [6, 30, 31]. Nonetheless, GBS still remains one of the leading causes of death for infants worldwide and has emerged as a frequent cause of invasive infections in adults with underlying medical conditions [32–36]. Thus, the development of a GBS vaccine is of high importance. Although trivalent polysaccharide conjugate vaccines (PCVs) targeting GBS capsular polysaccharide have undergone early stages of clinical trials and pentavalent PCVs are under development [6, 30, 31], recent epidemiological and immunological studies indicate that the use of multivalent PCVs for preventing GBS infections may be of limited efficacy because of their limited coverage, owing to serotype specific response, potential problems of serotype replacement across and within regions, and high production costs. In the present study, we also found that only 66% of clinical isolates belonged to pentavalent vaccine serotypes (ie, Ia, Ib, II, III, and V). Therefore, there is an urgent need to develop broad-spectrum vaccines for GBS.
SRR glycoproteins are well-conserved cell wall–anchored proteins expressed by the majority of Gram-positive bacteria and involved in bacterial adherence and invasion during infections. The GBS SRR adhesin Srr1, which our epidemiological study showed was expressed by the majority (86.7%) of GBS clinical isolates, has also been linked to pathogenicity in multiple studies [21–24, 37]. Our results further showed that the binding domain of Srr1 elicited highly protective immune responses against GBS infection and that the Srr1 binding domain latch sequence was critical to elicit the protective humoral and adaptive immune response. Another important result presented in this study was that latch vaccination provided cross-protection against heterologous serogroups of GBS. Thus, heterologous protection against different serotypes by the latch vaccine is of particular relevance.
Moreover, we found that 52% of serotype III isolates (20 of 39) did not express either srr1 or srr2. Our unpublished data revealed that these isolates belong to 1 sequence type (ST27) and may express a novel Srr adhesin. Further study of this unique serotype III isolate may provide additional insights into the molecular pathogenesis of GBS infection and facilitate the development of a broad-spectrum vaccine.
In summary, this is the first report that a latch-based vaccine could provide serotype-independent protection against GBS. Given that its homologs appeared to be expressed by other Gram-positive bacteria, this peptide may constitute a promising candidate for a novel vaccine target. By considering the appearance of serotype replacement and switching in GBS clinical isolates, the findings of this study, which revealed a serotype-independent protection mechanism against GBS, may be useful for clinical application.
Supplementary Data
Supplementary materials are available at The Journal of Infectious Diseases online. Consisting of data provided by the authors to benefit the reader, the posted materials are not copyedited and are the sole responsibility of the authors, so questions or comments should be addressed to the corresponding author.
Notes
Financial support. This work was supported by the National Research Foundation of Korea (grant NRF-2015R1D1A1A01059338 to J. H. L. and grants NRF-2017M2A2A6A02020925 and NRF-2015M2A2A6A04044375 to H. S. S.), the Ministry of Food and Drug Administration (16172MFDS264 to J. Y. S.), the Ewha Womans University School of Medicine (intramural research promotion grants to J. H. L.), the Nuclear Research and Development Program of the Ministry of Science and Information and Communication Technology (to S.-M. L.), and the National Institutes of Health (R01A141513 to P. M. S.).
Potential conflicts of interest. All authors: No reported conflicts of interest. All authors have submitted the ICMJE Form for Disclosure of Potential Conflicts of Interest. Conflicts that the editors consider relevant to the content of the manuscript have been disclosed.
References