-
PDF
- Split View
-
Views
-
Cite
Cite
Edward Essuman, Bryan Grabias, Nitin Verma, Joanna K Chorazeczewski, Abhai K Tripathi, Godfree Mlambo, Ebenezer A Addison, Albert G B Amoah, Isabella Quakyi, Miranda S Oakley, Sanjai Kumar, A Novel Gametocyte Biomarker for Superior Molecular Detection of the Plasmodium falciparum Infectious Reservoirs, The Journal of Infectious Diseases, Volume 216, Issue 10, 15 November 2017, Pages 1264–1272, https://doi.org/10.1093/infdis/jix442
- Share Icon Share
Abstract
Complete malaria eradication and optimal use of transmission-reducing interventions require knowledge of submicroscopic infectious reservoirs among asymptomatic individuals. Even submicroscopic levels of Plasmodium falciparum gametocytes can infect mosquitoes and promote onward transmission. Most efforts to identify gametocyte carriers use polymerase chain reaction amplification of the gametocyte-specific transcript Pfs25.
To expand the repertoire of biomarkers available for superior gametocyte detection, we compared the gene expression profiles of gametocytes and asynchronous blood-stage P. falciparum parasites by microarray technology. This allowed the identification of 56 molecules abundantly expressed in the gametocyte stage of the parasite. The analytical sensitivity for gametocyte detection was evaluated for 25 genes with the highest expression levels.
One candidate, Pfg17, exhibited superior analytical sensitivity against a panel of gametocyte-spiked whole blood, detecting 10 gametocytes/mL; in comparison, Pfs25 detected only 25.3 gametocytes/mL. Pfg17 also exhibited superior clinical sensitivity, identifying 19.1% more samples from blood-film microscopy–negative Ghanaian children and 40% more samples from asymptomatic adults as gametocyte positive.
Cumulatively, our results suggest Pfg17 is an excellent biomarker for detecting asymptomatic infectious reservoirs otherwise missed by the most sensitive molecular method available. Our study has also improved the repertoire of transmission-stage antigens available for evaluation as candidate vaccines.
Approximately half of the world’s population lives in an area of active malaria transmission and is at risk for the disease [1]. Worldwide malaria elimination and eradication efforts have led to extraordinary scale-up of malaria control interventions over the last decade, with significantly greater access to effective treatments for clinical malaria, mass drug administration, as well as widespread individual use of insecticide-treated bed nets and indoor residual spraying. These interventions have led to a 37% decline in the global malaria incidence and a 60% decrease in malaria-specific mortality rates between 2000 and 2015 [2]. Despite these important gains, malaria remains a persistent public health threat, causing 438 000 deaths in 2015 [1].
Future malaria control strategies require proper identification of the reservoirs of transmission for effective allocation of control and eradication resources. Recent studies have improved our understanding of the contribution of different population characteristics (eg, clinical disease vs asymptomatic infection, age, and immunity status) to malaria transmission [3–5]. Individuals with clinical malaria are thought to be the most effective transmitters of infecting parasites to mosquitoes [6]; among the asymptomatic individuals, both microscopically positive and submicroscopic infections contribute to transmission, although submicroscopic individuals do so at a much lower rate [7–9].
Application of molecular tools has significantly improved the measurement of asymptomatic infections, including those in individuals who carry submicroscopic levels of gametocytes and are potentially infectious to mosquitoes [10]. Nucleic acid tests for the detection of circulating gametocytes generally use reverse-transcription polymerase chain reaction (RT-PCR) amplification of transcripts for the Plasmodium-specific marker Pfs25 [11]. Pfs25 protein is not expressed in the gametocyte stage and is found on only the surface of zygotes during transformation into ookinetes [12]. Additionally, Pfs25 is thought to be a female-specific marker [13] incapable of detecting male gametocytes, which typically constitute 30% of the total P. falciparum gametocyte burden at higher parasite densities [14]. Current nucleic acid tests using Pfs25 have reported sensitivities of approximately 20–100 gametocytes/mL [11, 15]. Identification of novel molecules more abundant and specific to the gametocyte stage of the parasite life cycle could potentially enhance the sensitivity of gametocyte detection assays, resulting in improved estimation of gametocyte carriage and more-accurate models of malaria transmission in areas of endemicity.
To increase the repertoire of sexual-stage parasite genes as biomarkers of detection and infectivity, we performed global gene expression profiling of P. falciparum stage V gametocytes by complementary RNA (cRNA) microarray technology. To ensure high specificity to parasite sexual stages, transcript expression levels in cultured gametocytes were compared directly to those for blood-stage parasites. Our study identified 56 gametocyte-specific genes that had >50-fold enhanced gametocyte expression. To find novel targets for gametocyte detection, the 25 most abundantly enriched gametocyte transcripts were used in a RT-PCR–based screening assay. Analytical sensitivity for each novel biomarker was compared to the ability of Pfs25-specific primers to detect serially diluted cultured gametocytes spiked into whole-blood specimens.
Our data demonstrate that, among the 25 genes screened, 2 novel and uncharacterized molecules, Pfg84 and Pfg136, exhibited comparable performance to primers specific to the gold standard, Pfs25. Specifically, amplification of Pfs25 allowed detection of 25.3 gametocytes/mL in whole-blood specimens. In contrast, primers specific to sequences from Pfg136 and Pfg84 in RT-PCR achieved sensitivity limits of 70.7 and 100 gametocytes/mL, respectively. However, the best-performing candidate was Pfg17, which could consistently detect as few as 10 gametocytes/mL. We therefore compared the ability of Pfs25 and Pfg17 to detect gametocytes in Ghanaian blood samples. Pfg17 identified 19.1% more samples as gametocyte positive in blood-film microscopy–negative children, while the overall rates of reactivity were similar in blood-film microscopy–positive children. Pfg17 was also superior at detecting asymptomatic reservoirs among Ghanaian adults, with 40% more samples being identified as gametocyte positive. The use of Pfg17 as a new marker for gametocyte infection would allow the development of a molecular assay with superior sensitivity and specificity for detection of submicroscopic reservoirs of transmission that would have otherwise remained undetected. Novel P. falciparum gametocyte–specific genes identified our data set can also be evaluated as candidate transmission-blocking vaccines and biomarkers of infectivity to mosquitoes.
MATERIALS AND METHODS
Asexual-Stage P. falciparum Culture
Asynchronous P. falciparum culture (NF54) was performed as described previously [16]. Briefly, parasites were cultured in human type O red blood cells from blood donors in modified Roswell Park Memorial Institute (RPMI) 1640 medium with 25 mM HEPES, equilibrated with 5% CO2, 5% O2, and 90% N2 and containing 10% pooled heat-inactivated human type O serum (obtained commercially and by the National Institutes of Health Blood Bank).
P. falciparum Gametocyte Culture
The human malaria parasite P. falciparum (NF54) was cultured in vitro according to the method previously described by Trager and Jenson [17], with minor modifications. Parasites were cultured using blood type O human erythrocytes at 4% hematocrit in complete medium (RPMI 1640 medium supplemented with 25 mM HEPES, 10 mM glutamine, 0.074 mM hypoxanthine, and 10% type O human serum). Erythrocytes were collected weekly from healthy donors under an institutional review board–approved protocol. Cultures were maintained under standard conditions at 37°C in a candle jar made of glass desiccators. Culture was initiated at 0.5% asynchronous asexual parasitemia from low-passage stock and maintained up to day 18 with daily medium changes. To block reinvasion of remaining asexual parasites and obtain pure and near synchronous gametocytes, cultures were treated with 50 mM N-acetyl-D-glucosamine for 72 hours between days 7 and 10.
RNA Preparation From P. falciparum Gametocyte–Spiked Panel Members and Ghanaian Blood Samples
Human whole-blood specimens were spiked and serially diluted with known counts of cultured P. falciparum stage V gametocytes to determine the limit of detection of Pfs25 and other potential novel molecules. Dried blood from capillary tube samples from Ghanaian individuals was rehydrated in 1× phosphate-buffered saline. For both preparations, red blood cells were lysed with 0.15% saponin. Pellets were spun down and lysed in 1 mL of Trizol reagent. Total RNA was extracted using chloroform, precipitated with 70% ethanol, and then purified using affinity columns of the Qiagen RNeasy Kit (Qiagen, Valencia, CA) according to the Qiagen protocols. Total RNA was eluted into a final volume of 60 μL. RT-PCR analysis for Pfs25 and the other molecules was performed using the iQ SYBR Green Supermix 1-step kit from Bio-Rad.
Microarray Studies
To identify P. falciparum gametocyte–enriched genes, we performed microarray analysis comparing cultured gametocytes with cultured asynchronous intraerythrocytic parasites in 2 independent experiments. Gametocytes and asynchronous blood-stage parasites were snap frozen in Trizol reagent and stored at −80°C until use. RNA was then purified, amplified, and labeled as described previously [18]. Equimolar amounts of labeled cRNA transcripts were then hybridized to the Agilent SurePrint oligonucleotide array (Agilent Technologies), containing 44 000 features (5648 distinct 60-mer probes for Pf 3D7 transcripts each spotted 8 times). The chip was scanned and underwent image analysis using Feature extraction software, version 9, and the microarray data set was analyzed using NIAID microarray database tools (available at: http://mAdb.niaid.nih.gov).
Real-Time PCR Amplification of Gametocyte Transcripts
Real-time PCR analyses and calculations were performed on a QuantStudio 3 Real-time PCR Thermocycler system (Applied Biosystems, Foster City, CA). RNA extracted from unspiked blood samples was used as a negative control. RT-PCR was performed using an annealing temperature of 57°C and data collection temperature of 65°C over 40 amplification cycles. Amplification of Pfs25 and the other novel biomarkers was performed in a total reaction volume of 20 µL, testing 2 µL of isolated RNA. Cutoffs for the identification of positive samples were determined from the mean threshold cycle values of these negative controls minus 2 standard deviations.
Clinical Study Site and Data Collection
Capillary blood samples used for this study were from Kpone Katamanso District Hospital, situated in the Greater Accra Region of Ghana, a malaria-endemic country that recorded about 10 million malaria cases during 2015. The study site was developed for multidisciplinary malaria studies by the University of Ghana School of Public Health [19]. Kpone Katamanso District is a peri-urban district that occupies an area of 215.4 km2, is located in the coastal-savannah zone, and has perennial malaria transmission, although intensity varies throughout the year. The district has an estimated population of 50 225. The study protocol was approved by the Ghana Health Service ethical review committee (GHS/RDD/ERC/Admin/16/158) and the Food and Drug Administration Research Involving Human Subjects Committee (protocol 09-122B). Study participants were children and asymptomatic adults aged 1 month to 16 years who were screened in the Kpone Katamanso District Hospital. Samples from children were screened for asexual-stage parasite infection, using blood-film microscopy. Adult samples were evaluated for blood-stage parasitemia by RT-PCR amplification of the P. falciparum 18S ribosomal gene.
Statistical Analysis
Statistical significance was calculated using a Student t test or analysis of variance where appropriate. The statistical significance of differences between paired Pfs25 and Pfg17 tests of clinical samples was evaluated using the McNemar test.
RESULTS
Expression Profile of the P. falciparum Gametocyte
To identify gametocyte-specific biomarkers that are differentially expressed, we performed microarray analysis comparing RNA isolated from P. falciparum gametocyte and asynchronous intraerythrocytic cultures in 2 independent experiments. We chose conservative criteria that required ≥50-fold gametocyte upregulation in all 4 replicates and a P value of ≤ .01 to select genes for further study. Using these criteria, there were 44 significant genes in the first microarray experiment and 50 significant genes in the second microarray experiment involving 56 genes (the majority of genes were present in both data sets; Supplementary Table 1). Of these 56 genes, 29 have annotated functions (Table 1), whereas 27 encode conserved Plasmodium proteins or, probably, proteins with unknown functions.
Gametocyte-Enriched Transcripts With Annotated Functions Identified by Microarray
Gene . | Protein Description . | Function . | Fold Change . |
---|---|---|---|
PF3D7_1031000 | 25-kDa ookinete surface antigen precursor (Pfs25) | … | 168.0 |
PF3D7_1207700 | Blood-stage antigen 41–3 precursor | … | 90.1 |
PF3D7_0630000 | CPW-WPC family protein | … | 65.2 |
PF3D7_0216000 | DEAD/DEAH box helicase | … | 91.1 |
PF3D7_1351600 | Glycerol kinase | Glycerol sequestration | 106.6 |
PF3D7_0708600 | Inner-membrane-complex protein 1d, putative | … | 55.0 |
PF3D7_0724900 | Kinesin-19, putative | Motor protein | 82.0 |
PF3D7_1216500 | Male development gene 1 | … | 85.1 |
PF3D7_0530800 | CPW-WPC family protein | … | 54.2 |
PF3D7_1250100 | Osmiophilic body protein G377 | Gametocyte erythrocte egress | 69.9 |
PF3D7_1346800 | 6-cysteine protein | Gametocyte immune evasion | 84.4 |
PF3D7_0621400 | Pf77 protein | Subpellicular membrane | 78.2 |
PF3D7_1129600 | Phosphatidylinositol-4-phosphate-5-kinase | … | 68.7 |
PF3D7_1033800 | Plasmepsin VII | Mosquito midgut invasion | 59.3 |
PF3D7_1477700 | Plasmodium-exported protein (PHISTa) | … | 83.4 |
PF3D7_1302100 | Gamete antigen 27/25 | Role in gametocytogenesis | 61.3 |
PF3D7_0930000 | Procollagen lysine 5-dioxygenase | … | 90.7 |
PF3D7_1453500 | Pyridine nucleotide transhydrogenase, putative | … | 93.8 |
PF3D7_0828000 | Rhomboid protease ROM3 | Role in sporozoite production | 59.0 |
PF3D7_1201600 | NIMA-related kinase 3 | … | 93.0 |
PF3D7_0816500 | Small heat shock protein HSP20, putative | … | 88.4 |
PF3D7_0625100 | Sphingomyelin synthase 2 | … | 80.1 |
PF3D7_0518800 | Secreted ookinete protein, putative | … | 124.5 |
PF3D7_0630200 | Secreted ookinete protein, putative | … | 75.2 |
PF3D7_0904200 | PH domain–containing protein, putative | … | 176.7 |
PF3D7_1210200 | Zinc finger protein, putative | … | 173.2 |
PF3D7_1220000 | Zinc finger protein, putative | … | 69.8 |
PF3D7_1358600 | Zinc finger protein, putative | … | 144.1 |
PF3D7_0517500 | UTP-glucose-1-phosphate uridylyltransferase, putative | … | 67.3 |
Gene . | Protein Description . | Function . | Fold Change . |
---|---|---|---|
PF3D7_1031000 | 25-kDa ookinete surface antigen precursor (Pfs25) | … | 168.0 |
PF3D7_1207700 | Blood-stage antigen 41–3 precursor | … | 90.1 |
PF3D7_0630000 | CPW-WPC family protein | … | 65.2 |
PF3D7_0216000 | DEAD/DEAH box helicase | … | 91.1 |
PF3D7_1351600 | Glycerol kinase | Glycerol sequestration | 106.6 |
PF3D7_0708600 | Inner-membrane-complex protein 1d, putative | … | 55.0 |
PF3D7_0724900 | Kinesin-19, putative | Motor protein | 82.0 |
PF3D7_1216500 | Male development gene 1 | … | 85.1 |
PF3D7_0530800 | CPW-WPC family protein | … | 54.2 |
PF3D7_1250100 | Osmiophilic body protein G377 | Gametocyte erythrocte egress | 69.9 |
PF3D7_1346800 | 6-cysteine protein | Gametocyte immune evasion | 84.4 |
PF3D7_0621400 | Pf77 protein | Subpellicular membrane | 78.2 |
PF3D7_1129600 | Phosphatidylinositol-4-phosphate-5-kinase | … | 68.7 |
PF3D7_1033800 | Plasmepsin VII | Mosquito midgut invasion | 59.3 |
PF3D7_1477700 | Plasmodium-exported protein (PHISTa) | … | 83.4 |
PF3D7_1302100 | Gamete antigen 27/25 | Role in gametocytogenesis | 61.3 |
PF3D7_0930000 | Procollagen lysine 5-dioxygenase | … | 90.7 |
PF3D7_1453500 | Pyridine nucleotide transhydrogenase, putative | … | 93.8 |
PF3D7_0828000 | Rhomboid protease ROM3 | Role in sporozoite production | 59.0 |
PF3D7_1201600 | NIMA-related kinase 3 | … | 93.0 |
PF3D7_0816500 | Small heat shock protein HSP20, putative | … | 88.4 |
PF3D7_0625100 | Sphingomyelin synthase 2 | … | 80.1 |
PF3D7_0518800 | Secreted ookinete protein, putative | … | 124.5 |
PF3D7_0630200 | Secreted ookinete protein, putative | … | 75.2 |
PF3D7_0904200 | PH domain–containing protein, putative | … | 176.7 |
PF3D7_1210200 | Zinc finger protein, putative | … | 173.2 |
PF3D7_1220000 | Zinc finger protein, putative | … | 69.8 |
PF3D7_1358600 | Zinc finger protein, putative | … | 144.1 |
PF3D7_0517500 | UTP-glucose-1-phosphate uridylyltransferase, putative | … | 67.3 |
Gametocyte-Enriched Transcripts With Annotated Functions Identified by Microarray
Gene . | Protein Description . | Function . | Fold Change . |
---|---|---|---|
PF3D7_1031000 | 25-kDa ookinete surface antigen precursor (Pfs25) | … | 168.0 |
PF3D7_1207700 | Blood-stage antigen 41–3 precursor | … | 90.1 |
PF3D7_0630000 | CPW-WPC family protein | … | 65.2 |
PF3D7_0216000 | DEAD/DEAH box helicase | … | 91.1 |
PF3D7_1351600 | Glycerol kinase | Glycerol sequestration | 106.6 |
PF3D7_0708600 | Inner-membrane-complex protein 1d, putative | … | 55.0 |
PF3D7_0724900 | Kinesin-19, putative | Motor protein | 82.0 |
PF3D7_1216500 | Male development gene 1 | … | 85.1 |
PF3D7_0530800 | CPW-WPC family protein | … | 54.2 |
PF3D7_1250100 | Osmiophilic body protein G377 | Gametocyte erythrocte egress | 69.9 |
PF3D7_1346800 | 6-cysteine protein | Gametocyte immune evasion | 84.4 |
PF3D7_0621400 | Pf77 protein | Subpellicular membrane | 78.2 |
PF3D7_1129600 | Phosphatidylinositol-4-phosphate-5-kinase | … | 68.7 |
PF3D7_1033800 | Plasmepsin VII | Mosquito midgut invasion | 59.3 |
PF3D7_1477700 | Plasmodium-exported protein (PHISTa) | … | 83.4 |
PF3D7_1302100 | Gamete antigen 27/25 | Role in gametocytogenesis | 61.3 |
PF3D7_0930000 | Procollagen lysine 5-dioxygenase | … | 90.7 |
PF3D7_1453500 | Pyridine nucleotide transhydrogenase, putative | … | 93.8 |
PF3D7_0828000 | Rhomboid protease ROM3 | Role in sporozoite production | 59.0 |
PF3D7_1201600 | NIMA-related kinase 3 | … | 93.0 |
PF3D7_0816500 | Small heat shock protein HSP20, putative | … | 88.4 |
PF3D7_0625100 | Sphingomyelin synthase 2 | … | 80.1 |
PF3D7_0518800 | Secreted ookinete protein, putative | … | 124.5 |
PF3D7_0630200 | Secreted ookinete protein, putative | … | 75.2 |
PF3D7_0904200 | PH domain–containing protein, putative | … | 176.7 |
PF3D7_1210200 | Zinc finger protein, putative | … | 173.2 |
PF3D7_1220000 | Zinc finger protein, putative | … | 69.8 |
PF3D7_1358600 | Zinc finger protein, putative | … | 144.1 |
PF3D7_0517500 | UTP-glucose-1-phosphate uridylyltransferase, putative | … | 67.3 |
Gene . | Protein Description . | Function . | Fold Change . |
---|---|---|---|
PF3D7_1031000 | 25-kDa ookinete surface antigen precursor (Pfs25) | … | 168.0 |
PF3D7_1207700 | Blood-stage antigen 41–3 precursor | … | 90.1 |
PF3D7_0630000 | CPW-WPC family protein | … | 65.2 |
PF3D7_0216000 | DEAD/DEAH box helicase | … | 91.1 |
PF3D7_1351600 | Glycerol kinase | Glycerol sequestration | 106.6 |
PF3D7_0708600 | Inner-membrane-complex protein 1d, putative | … | 55.0 |
PF3D7_0724900 | Kinesin-19, putative | Motor protein | 82.0 |
PF3D7_1216500 | Male development gene 1 | … | 85.1 |
PF3D7_0530800 | CPW-WPC family protein | … | 54.2 |
PF3D7_1250100 | Osmiophilic body protein G377 | Gametocyte erythrocte egress | 69.9 |
PF3D7_1346800 | 6-cysteine protein | Gametocyte immune evasion | 84.4 |
PF3D7_0621400 | Pf77 protein | Subpellicular membrane | 78.2 |
PF3D7_1129600 | Phosphatidylinositol-4-phosphate-5-kinase | … | 68.7 |
PF3D7_1033800 | Plasmepsin VII | Mosquito midgut invasion | 59.3 |
PF3D7_1477700 | Plasmodium-exported protein (PHISTa) | … | 83.4 |
PF3D7_1302100 | Gamete antigen 27/25 | Role in gametocytogenesis | 61.3 |
PF3D7_0930000 | Procollagen lysine 5-dioxygenase | … | 90.7 |
PF3D7_1453500 | Pyridine nucleotide transhydrogenase, putative | … | 93.8 |
PF3D7_0828000 | Rhomboid protease ROM3 | Role in sporozoite production | 59.0 |
PF3D7_1201600 | NIMA-related kinase 3 | … | 93.0 |
PF3D7_0816500 | Small heat shock protein HSP20, putative | … | 88.4 |
PF3D7_0625100 | Sphingomyelin synthase 2 | … | 80.1 |
PF3D7_0518800 | Secreted ookinete protein, putative | … | 124.5 |
PF3D7_0630200 | Secreted ookinete protein, putative | … | 75.2 |
PF3D7_0904200 | PH domain–containing protein, putative | … | 176.7 |
PF3D7_1210200 | Zinc finger protein, putative | … | 173.2 |
PF3D7_1220000 | Zinc finger protein, putative | … | 69.8 |
PF3D7_1358600 | Zinc finger protein, putative | … | 144.1 |
PF3D7_0517500 | UTP-glucose-1-phosphate uridylyltransferase, putative | … | 67.3 |
Next we conducted a systematic ranking of highly expressed gametocyte-specific genes identified in our microarray analysis that could be evaluated as novel gametocyte detection markers. We plotted the fold change and cy3 signal for each gene in our data set (Figure 1) and hypothesized that the genes with a high fold change and low cy3 signal (a surrogate measure of low transcript abundance in blood-stage parasites) would be a suitable candidate as a gametocyte detection marker. We found that Pfs25 (PF10_0303) was transcriptionally overexpressed in gametocytes by 168-fold as compared to asynchronous intraerythrocytic parasites. Using these selection criteria, we designed primers specific for the 25 most enriched gametocyte-specific genes in our data set to identify novel biomarkers that could improve gametocyte detection and evaluated their analytical sensitivity against a P. falciparum gametocyte reference panel.
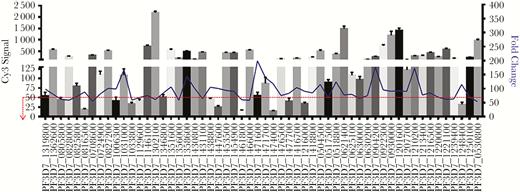
Gene expression profiling of gametocyte-enriched transcripts by complementary RNA (cRNA) microarray technology. Labeled cRNA extracts prepared from both stage V gametocytes and asynchronous blood-stage parasite culture were hybridized to an Agilent SurePrint oligonucleotide array containing 44 000 features. Criteria for the selection of differentially expressed genes included >50-fold upregulation in the Plasmodium falciparum gametocyte and a P value of <.01 across 4 replicates. In this analysis, 56 unique transcripts were identified as significantly enriched in the gametocyte stage. Selection of specific markers that were further evaluated in a reverse-transcription polymerase chain reaction gametocyte-detection assay was additionally guided by the blood-stage–specific cRNA label (cy3) intensity level. High fold upregulation and low cy3 intensity would ensure that sensitive and specific biomarkers are evaluated for gametocyte detection.
Comparison of Analytical Sensitivity Between Pfs25 and Pfg17 for Detection of Gametocytes in Whole-Blood Specimens
Cultured P. falciparum stage V gametocytes were spiked into whole-blood specimens and serially diluted to generate samples containing 10 000 parasites/mL, 1000 parasites/mL, 100 parasites/mL, 10 parasites/mL, and 1 parasite/mL. To evaluate the analytical sensitivity of novel biomarkers for gametocyte detection, primers were designed using BLAST searches to ensure target specificity, while Pfs25 primer sequences were based on the work by Wampfler et al [11] (Supplemental Table 2). Pfs25 demonstrated clear and reproducible detection of as few as 25.3 gametocytes/mL (Figure 2). Of the 25 novel highly abundant molecules tested, several demonstrated clear and consistent performance for amplification of parasite RNA. For example, Pfg84 was able to detect as few as 100 gametocytes. Similarly, Pfg136 displayed a detection limit of 70.7 gametocytes/mL. However, by far the best results, when compared to Pfs25 detection, were obtained with primers targeting Pfg17, which consistently detected as few as 10 gametocytes/mL (Figure 2). While Pfg17 amplification of the 1 parasite/mL sample exhibited mean threshold cycle values slightly above the negative controls, the standard error was too high to allow adequate detection when assessed by t testing (P > .05). Additionally, at this gametocyte dilution, typically not all replicates reliably exhibit Pfg17 amplification. We therefore chose to compare the clinical sensitivity of Pfg17 to that of Pfs25 for the detection of P. falciparum gametocytes in blood specimens collected from children and adults living in a malaria-endemic area of Ghana.
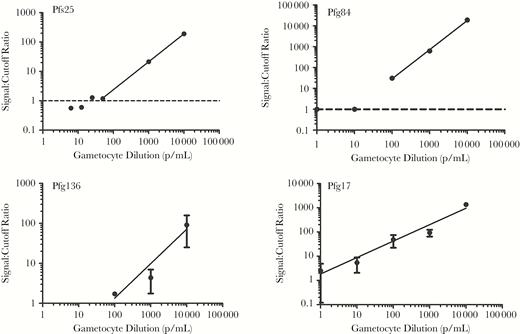
Analytical sensitivity of different primer sets for a stage V gametocyte–spiked reference panel. Primers against the gold standard gametocyte antigen Pfs25 were compared to novel transcripts identified by microarray comparison of gametocyte and blood-stage parasite gene expression profiles. RNA extracted from gametocytes serially diluted into whole human blood was evaluated by reverse-transcription polymerase chain reaction. Primers specific to Pfg136 achieved a detection limit of 70.7 gametocytes/mL, while Pfg84 detected as few as 100 gametocytes/mL. The gold standard Pfs25 demonstrated clear and reproducible detection of as few as 25.3 gametocytes/mL. Pfg17 was the only transcript evaluated that was superior to Pfs25, detecting as few as 10 gametocytes/mL.
Pfg17 possesses a predicted signal peptide, a transmembrane domain, and 2 low-complexity regions near the N-terminus. It shares moderate homology with other unknown proteins in other Plasmodium species. However, no conserved domains or protein motifs were identified. Specifically, sequence alignment with published P. falciparum protein sequences revealed that amino acids 3–65 in Pfg17 shares 30% identity with a putative cyclin-dependent kinase (XP_001351461.1) and that amino acids 9–21 of Pfg17 are 69% identical to another conserved Plasmodium protein with unknown function (XP_001350208.1). Analysis of Pfg17 peptide sequence by Protein Homology/Analogy Recognition Engine V2.0, using a hidden Markov model, also suggests limited homology with the Rap/Ran GAP (GTPase-activating protein) superfamily of proteins.
Superior Clinical Sensitivity of Pfg17 in Detecting Submicroscopic Malaria Parasite Reservoirs
The clinical sensitivity of the P. falciparum gametocyte gene encoding Pfg17 was determined in blood samples from 80 children (47 had negative results 33 had positive results of blood-film microscopy) and 30 adults living in P. falciparum–endemic areas in Ghana. Submicroscopic reservoirs are individuals who are negative for parasitemia by blood-film microscopy but test positive by PCR. While equally effective at identifying the presence of P. falciparum gametocytes in blood-film microscopy–positive individuals, Pfg17 identified approximately 19.1% (9 of 47) more blood-film microscopy–negative children as gametocyte positive (P < .05; Figure 3) than Pfs25. Furthermore, among all blood-film microscopy–positive children, the asexual parasite burden assessed by blood-film microscopy correlated positively with an earlier threshold amplification cycle (and thus higher abundance) of both Pfs25 and Pfg17 (Supplementary Figure 1). When the data were stratified by age group, other noteworthy trends emerged. Although the differences were not statistically significant, younger children (ages 0–5 years) had marginally higher rates of blood-film microscopy–confirmed asexual blood-stage parasitemia (51.1% versus 45.9% in older children), while older children (ages 6–16 years) generally had higher rates of PCR-confirmed gametocytemia when measured with both Pfs25 and Pfg17 (Figure 3 and Table 2). These results could suggest that the infective reservoir for P. falciparum malaria resides within older children who may harbor asexual parasite burdens below the detection limit of most commonly used screening methods. Importantly, across both these groups, Pfg17 consistently identified higher numbers of individuals as gametocyte positive than Pfs25, suggesting superiority at identifying potential carriers that would have otherwise been missed (Figure 3).
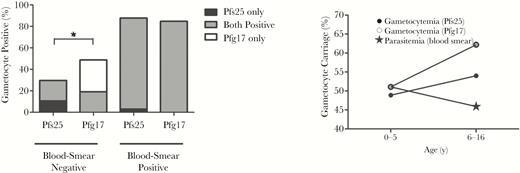
Detection of the malaria infectious reservoir in Ghanaian children. RNA was extracted from blood samples acquired from Ghanaian children aged 0–16 years and evaluated for gametocyte carriage by reverse-transcription polymerase chain reaction, using primers specific for Pfs25 and Pfg17. Asexual parasitemia was confirmed via blood-film microscopy. While Pfs25 and Pfg17 displayed no significant difference in sensitivity among positive specimens (n = 33), Pfg17 detected gametocytes in 19.1% more of the blood-film microscopy–negative samples (n = 47). Data were further stratified by age to evaluate any apparent differences in asexual- and sexual-stage parasite prevalence. While no differences observed were statistically significant, older children aged 6–16 years generally had overall higher rates of gametocyte carriage and lower rates of asexual-stage parasitemia. *P <.05 by the McNemar test.
Prevalence of Blood-Stage and Sexual-Stage Parasitemia in Ghanaian Children, Stratified by Age
Characteristic . | Patient Age . | |
---|---|---|
0–5 y . | 6–15 y . | |
Blood-film microscopy positive, % | 51.1 | 45.9 |
Pfs25 gametocyte positive, % | 48.9 | 54.0 |
Pfg17 gametocyte positive, % | 51.1 | 62.2 |
Characteristic . | Patient Age . | |
---|---|---|
0–5 y . | 6–15 y . | |
Blood-film microscopy positive, % | 51.1 | 45.9 |
Pfs25 gametocyte positive, % | 48.9 | 54.0 |
Pfg17 gametocyte positive, % | 51.1 | 62.2 |
Prevalence of Blood-Stage and Sexual-Stage Parasitemia in Ghanaian Children, Stratified by Age
Characteristic . | Patient Age . | |
---|---|---|
0–5 y . | 6–15 y . | |
Blood-film microscopy positive, % | 51.1 | 45.9 |
Pfs25 gametocyte positive, % | 48.9 | 54.0 |
Pfg17 gametocyte positive, % | 51.1 | 62.2 |
Characteristic . | Patient Age . | |
---|---|---|
0–5 y . | 6–15 y . | |
Blood-film microscopy positive, % | 51.1 | 45.9 |
Pfs25 gametocyte positive, % | 48.9 | 54.0 |
Pfg17 gametocyte positive, % | 51.1 | 62.2 |
To evaluate overall gametocyte prevalence among older populations, additional samples obtained from a cohort of asymptomatic adults (ages 32–60 years) were evaluated for gametocytemia. Asexual-stage parasitemias in this cohort were not evaluated by blood-film microscopy, so RT-PCR for both asexual- and sexual-stage parasitemia using primers specific to 18S and Pfs25/Pfg17, respectively, was performed. Seventy percent of these adult samples (21 of 30) were reactive as measured by P. falciparum blood-stage 18S primers in our RT-PCR assay. Of the 21 specimens positive for blood-stage parasites by PCR, 33.3% (7 of 21) were positive for gametocytes by Pfs25 and 66.7% (14 of 21) were positive by Pfg17 (Table 3). Among the 9 adult samples determined to be negative for blood-stage parasites by 18S RT-PCR, 2 were identified as positive by both Pfs25 and Pfg17, 1 was reactive to Pfs25 alone, and 6 were identified as positive by Pfg17 alone. It is unclear whether gametocytemia in the absence of confirmed blood-stage parasitemia in these cases is simply reflective of a more sensitive PCR assay for the gametocyte markers or of low-density asexual infections with relatively higher burdens of gametocyte carriage or whether these particular patients reflect self-treated blood-stage parasite infections with latent gametocytemias [20, 21]. Cumulatively, among all adult samples tested, Pfs25 identified overall 10 of 30 adults (33%) as gametocyte carriers, while Pfg17 identified 22 of 30 individuals (73.3%) as carriers for a statistically significant improvement in sensitivity (P < .05; Figure 4). Among all age groups, Pfg17 identified 18.2% more samples (20 of 110) as gametocyte positive.
Performance Comparison of Pfs25 and Pfg17 for Gametocyte Detection in Asymptomatic Adults Screened for Blood-Stage Parasite Reactivity with 18S Primers
18S Primer Reactivity . | Adults Screened, No. . | Pfg17 Status . | Pfs25 Status . | ||
---|---|---|---|---|---|
Negative . | Positive . | Negative . | Positive . | ||
Positive | 21 | 7 | 14 | 14 | 7 |
Negative | 9 | 1 | 8 | 6 | 3 |
Total | 30 | 8 | 22 | 20 | 10 |
18S Primer Reactivity . | Adults Screened, No. . | Pfg17 Status . | Pfs25 Status . | ||
---|---|---|---|---|---|
Negative . | Positive . | Negative . | Positive . | ||
Positive | 21 | 7 | 14 | 14 | 7 |
Negative | 9 | 1 | 8 | 6 | 3 |
Total | 30 | 8 | 22 | 20 | 10 |
Performance Comparison of Pfs25 and Pfg17 for Gametocyte Detection in Asymptomatic Adults Screened for Blood-Stage Parasite Reactivity with 18S Primers
18S Primer Reactivity . | Adults Screened, No. . | Pfg17 Status . | Pfs25 Status . | ||
---|---|---|---|---|---|
Negative . | Positive . | Negative . | Positive . | ||
Positive | 21 | 7 | 14 | 14 | 7 |
Negative | 9 | 1 | 8 | 6 | 3 |
Total | 30 | 8 | 22 | 20 | 10 |
18S Primer Reactivity . | Adults Screened, No. . | Pfg17 Status . | Pfs25 Status . | ||
---|---|---|---|---|---|
Negative . | Positive . | Negative . | Positive . | ||
Positive | 21 | 7 | 14 | 14 | 7 |
Negative | 9 | 1 | 8 | 6 | 3 |
Total | 30 | 8 | 22 | 20 | 10 |
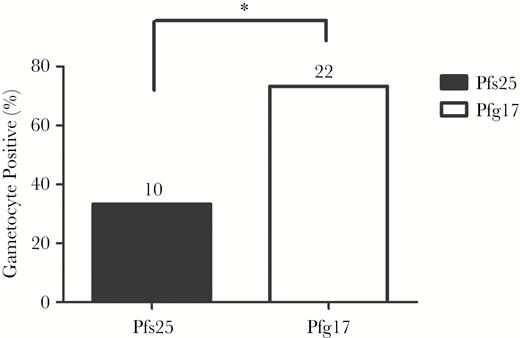
Detection of the malarial parasite infectious reservoir in asymptomatic Ghanaian adults. Gametocyte carriage was assessed in specimens from asymptomatic adults (n = 30) whose blood-film microscopy status was unknown. RT-PCR analysis using blood-stage 18S primers was used to identify specimens positive for asexual-stage parasites. Follow-up reverse-transcription polymerase chain reaction (RT-PCR) amplification of Pfs25 and Pfg17 was evaluated to determine gametocyte carriage. Seventy percent of these adult samples (21 of 30) were reactive, as measured by Plasmodium blood-stage 18S primers in our RT-PCR assay. Of the 21 PCR-positive blood-stage specimens, 33.3% (7 of 21) were positive for gametocytes by Pfs25, and 66.7% (14 of 21) were positive by Pfg17. Among the 9 adult specimens that were negative for blood-stage parasites by 18S RT-PCR, 2 were identified as positive by both Pfs25 and Pfg17, 1 was reactive to Pfs25 alone, and 6 were identified as positive by Pfg17 alone. *P <.05 by the McNemar test.
To further validate the use of Pfg17 as a diagnostic marker of gametocyte carriage, we investigated the genetic diversity and variation in the gene encoding Pfg17 by aligning the amplified sequence obtained from the Pfg17-specific primers to 202 unique isolates of the Pfg17 gene acquired across different age groups, geographic locations, years, and time points of specimen collection (PlasmoDB). The Pfg17 amplification target used for gametocyte detection demonstrated 100% sequence conservation among all isolates analyzed.
DISCUSSION
Eradication of malaria will require further knowledge of the submicroscopic malaria parasite infectious reservoir. As low as 1 gametocyte/μL of blood could still be infectious to mosquitoes [22]. It is therefore critical to identify potentially asymptomatic, low-gametocytemic persons perpetuating the transmission cycle without being adequately treated. The major objective of this study was to identify and evaluate highly sensitive and specific biomarkers for the detection of P. falciparum gametocytes in blood. We first compared the transcriptomes of the P. falciparum gametocyte and asynchronous blood-stage parasites by microarray analysis to identify highly upregulated gametocyte-specific transcripts. Using this approach, we identified 56 statistically significant genes that were abundantly overexpressed (≥50-fold) in gametocytes. Previous studies have also used a genomics approach to map the transcriptome/proteome of the gametocyte stage of the malaria parasite [23, 24]. Among the 56 statistically significant genes, we chose to further evaluate those that had a low cy3 signal and were therefore transcriptionally absent during the blood stage of the malaria parasite. By this logic, we selected 25 gametocyte-specific genes that had >50-fold expression in microarray analyses.
In systematic analytical studies using a validated P. falciparum gametocyte panel, Pfg17 was the only molecule that has demonstrated better analytical sensitivity than Pfs25 (detecting fewer parasites/mL). Pfg17 was abundantly (by 98.4-fold) and uniquely (cy3 signal, 54.7) expressed in gametocytes as compared to blood-stage parasites. Pfg17 also demonstrated higher clinical sensitivity than Pfs25 in blood-film microscopy–negative and asymptomatic individuals (Pfg17 identified 20% more children and 40% more adults as gametocyte positive).
Silvestrini et al has previously demonstrated that Pfg17 is expressed in stage I, II, and V gametocytes [23]. In subsequent studies, Frech et al demonstrated that Pfg17 is absent in P. vivax malaria and proposed that this gene could have a possible role in human virulence [25]. More recently, Coleman et al showed that knock down of P. falciparum histone deacetylase 2 results in transcriptional upregulation of genes associated with gametocyte conversion including Pfg17 [26]. However, the biological role and cellular function of Pfg17 has not been established. The fact that the nucleotide sequence of Pfg17 is conserved among P. falciparum isolates makes it an attractive detection target.
The question of which group of individuals in a population is the most significant contributor of onward transmission in mosquitoes is important from a malaria control perspective; however, the relationship between the gametocyte burden and key discriminatory factors such as asexual-stage parasite burden or individual age is not always clear. Some reports have demonstrated a positive association between asexual- and sexual-stage parasite densities [27, 28], while others show the inverse [29]. Our RT-PCR analysis of blood samples acquired from Ghanaian children demonstrates that the relative gametocyte burden, as measured by RT-PCR amplification of Pfs25 and Pfg17, exhibited a statistically significant positive correlation with higher asexual-stage parasite burdens, as measured by blood-film microscopy (Pfs25, P = .0006; Pfg17, P = .0028; Supplementary Figure 1). These data corroborate observations in high-transmission areas, where high asexual-stage parasitemias and similarly high concomitant gametocytemias are often observed in younger children [30]. More recently, in a study in Malawi, 5–15-year-old school children had a higher odds than >16-year-old adults in being gametocyte carriers, as determined by a molecular test [31].
Despite children bearing higher asexual- and sexual-stage parasite burdens, some reports have failed to identify a distinct difference in infectiousness or transmission potential among different age groups [32, 33]. Indeed, the relationship between overall gametocyte burden and infectiousness to a mosquito vector may not be direct, with higher gametocyte levels not necessarily ensuring effective transmission [9, 34]. More–in-depth analyses that adjust for age-specific mosquito biting rates and larger representation in the population have identified adults as larger overall contributors to the infectious reservoir [35]. In individuals with no detectable asexual-stage parasite infection, the proportion of gametocytemic individuals increases with age [36]. Furthermore, sera from older infected individuals have also shown a lower propensity for transmission-reducing activity as measured in a standard membrane feeding assay [37], despite the absence of any age-dependent correlation for the presence of potential transmission-blocking antibodies to both Pfs230 and Pfs48/45 [38]. Cumulatively these data, when combined with our observations, suggests that the most important component of the infectious reservoir of malaria resides in older, asymptomatic adults. This population is precisely where our newly discovered gametocyte biomarker, Pfg17, most clearly outperforms Pfs25.
The usefulness of higher sensitivity molecular tests in detecting submicroscopic individuals who may be infectious to mosquitoes in malaria control strategies is under discussion [10]. Contrary to conventional wisdom, higher proportions of asymptomatic submicroscopic infections were observed in low-transmission areas (eg, Southeast Asia) than in high-transmission settings [39]. Thus, it is clear that a molecular test based on Pfg17 would assist in determining the true gametocyte burden in both high- and low-transmission P. falciparum–endemic settings. Additionally, Pfg17-based assays may be more effective than Pfs25 in measuring the efficacy of antigametocyte drugs and vaccines in areas of endemicity. The other applications of our data set identifying novel P. falciparum gametocyte–specific genes include their evaluation in a candidate transmission-blocking vaccine and as biomarkers of infectiousness to mosquitoes.
Supplementary Data
Supplementary materials are available at The Journal of Infectious Diseases online. Consisting of data provided by the authors to benefit the reader, the posted materials are not copyedited and are the sole responsibility of the authors, so questions or comments should be addressed to the corresponding author.
Notes
Acknowledgments. We thank the Ghanaian individuals whose blood samples were used in this study.
Financial support. This work was funded by the PATH Malaria Vaccine Initiative and the Food and Drug Administration.
Potential conflicts of interest. All authors: No reported conflicts of interest. All authors have submitted the ICMJE Form for Disclosure of Potential Conflicts of Interest. Conflicts that the editors consider relevant to the content of the manuscript have been disclosed.
References
Author notes
E. E. and B. G. are co–first authors.