-
PDF
- Split View
-
Views
-
Cite
Cite
Taj Azarian, Lindsay R Grant, Maria Georgieva, Laura L Hammitt, Raymond Reid, Stephen D Bentley, David Goldblatt, Mathuran Santosham, Robert Weatherholtz, Paula Burbidge, Novalene Goklish, Claudette M Thompson, William P Hanage, Kate L O’Brien, Marc Lipsitch, Association of Pneumococcal Protein Antigen Serology With Age and Antigenic Profile of Colonizing Isolates, The Journal of Infectious Diseases, Volume 215, Issue 5, 1 March 2017, Pages 713–722, https://doi.org/10.1093/infdis/jiw628
- Share Icon Share
Abstract
Several Streptococcus pneumoniae proteins play a role in pathogenesis and are being investigated as vaccine targets. It is largely unknown whether naturally acquired antibodies reduce the risk of colonization with strains expressing a particular antigenic variant.
Serum immunoglobulin G (IgG) titers to 28 pneumococcal protein antigens were measured among 242 individuals aged <6 months–78 years in Native American communities between 2007 and 2009. Nasopharyngeal swabs were collected >– 30 days after serum collection, and the antigen variant in each pneumococcal isolate was determined using genomic data. We assessed the association between preexisting variant-specific antibody titers and subsequent carriage of pneumococcus expressing a particular antigen variant.
Antibody titers often increased across pediatric groups before decreasing among adults. Individuals with low titers against group 3 pneumococcal surface protein C (PspC) variants were more likely to be colonized with pneumococci expressing those variants. For other antigens, variant-specific IgG titers do not predict colonization.
We observed an inverse association between variant-specific antibody concentration and homologous pneumococcal colonization for only 1 protein. Further assessment of antibody repertoires may elucidate the nature of antipneumococcal antibody-mediated mucosal immunity while informing vaccine development.
Current pneumococcal conjugate vaccines (PCVs) have significantly reduced invasive disease caused by the included Streptococcus pneumoniae (pneumococcal) serotypes. However, the currently licensed vaccines, PCV-10 and PCV-13, target only 10 or 13 of the approximately 90 recognized pneumococcal capsular serotypes. In addition to incomplete coverage of disease-causing types, significant disadvantages of capsular vaccines include their production cost, production complexity, and serotype replacement. Although PCV formulations are still an attractive vaccine approach, these limitations have motivated pursuit of pneumococcal protein antigens as vaccine candidates. Protein-based vaccines would, in theory, generate robust antibody responses and be efficacious in young children and may decrease carriage [1].
Pneumococcal surface protein A (PspA), pneumococcal surface protein C (PspC), pilus (RrgA/B/C), pneumolysin (Ply) and neuraminidase (NanA) are among the pneumococcal proteins being investigated for use in vaccine formulations [1]. Studies suggest that in some cases combinations of these proteins may elicit better protection than any of the proteins themselves [1, 2]. In humans, antibodies to pneumococcal proteins can be detected during colonization and natural infection, providing protection from subsequent colonization and invasive disease [3–8]. Virolainen et al. showed that among children with invasive pneumococcal infections, those with lowest antibody titers to PspA were infected most frequently with pneumococci [9]. However, animal data show that, although antiprotein antibodies are correlated with protection against subsequent challenge, the mechanism of protection is not necessarily antibody mediated, suggesting antibody levels may correlate with degree of immune response but not necessarily exclusively mediate protection. Evidence of variant-specific protection, in which antibodies to a particular protein antigenic variant correlate with protection against colonization by homologous pneumococci (ie, those with that protein variant), would be more strongly indicative of antiprotein antibodies’ causal role in protection, as has been observed for serotype-specific anticapsular antibodies [10, 11].
At the same time, such evidence would provide a mechanism to explain the high level of sequence variation and signs of diversifying selection at these loci. Although these protein antigens are present in almost all pneumococci, they are also very diverse, and pneumococcal strains differ considerably in the particular antigenic variants they express [12]. Two clear examples are the surface-associated choline-binding proteins PspA and PspC. Both are encoded by polymorphic genes with clear structural variability, which becomes the basis for their division into 3 PspA families and 11 PspC groups [12, 13]. Studies suggest structural differences in these proteins impact the nature and specificity of the antibody response generated toward them. For example, family-specific antibody responses among children exposed to pneumococci possessing family 1 and 2 PspA variants have been observed [14].
Important uncertainties remain about the biologic function of protein antibodies and the extent to which their binding and activity are specific to particular variants of polymorphic antigens. Here, we investigate whether naturally acquired antibodies to protein antigens reduce the risk of nasopharyngeal acquisition (ie, colonization) with strains containing particular variants of diverse proteins such as PspA and PspC. To address this question, we used pneumococcal genomic data to identify variants of 21 pneumococcal protein antigens present in S. pneumoniae carriage isolates. We then assessed the association between antibody titers and subsequent colonization with S. pneumoniae strains expressing an antigen recognized by preexisting antibodies. We posited that individuals who had low antibody titers to a specific protein antigen variant would be more likely to be colonized with S. pneumoniae expressing that variant.
METHODS
Study Population, Serum Collection, and Nasopharyngeal Colonization
Individuals included in this study were a subset of participants in a larger prospective, longitudinal, observational cohort study of pneumococcal carriage among Navajo and White Mountain Apache families described elsewhere [15]. Briefly, participants living on reservations in the southwest United States were enrolled from March 2006 to March 2008. Demographic and epidemiological data are provided in Supplementary Table 1. Serum and nasopharyngeal (NP) specimens were obtained on the initial visit after recruitment, and NP samples were collected at each of 6 follow-up visits at 1-month intervals to determine pneumococcal carriage status [16]. We selected individuals who were negative for carriage at the initial visit, had an available enrollment serum sample, and subsequently had a pneumococcal isolate detected ≥30 days after serum collection. The Navajo Nation, White Mountain Apache tribe and the institutional review boards of the Johns Hopkins Bloomberg School of Public Health, the Navajo Nation, and the Phoenix Area Indian Health Services (IHS) approved this study. Written informed consent was obtained from adult participants and from caregivers of child participants. Assent was obtained from children aged 7–17 years.
Protein Antigen Serology
Serum immunoglobulin G to 28 pneumococcal protein antigens was measured using a direct-binding electrochemiluminescence-based multiplex assay [7]. Antibody levels among participants are expressed as a titer relative to the amount in a reference serum. The 28 antigens represent 21 pneumococcal proteins and alleles or structural variants of polymorphic proteins (Table 1). In particular, antibody titers were measured for variants of PcpC, PspA, pilus subunit RrgB, Ply, and pneumococcal histidine triad D (PhtD). Four PspC variants, representing 4 of the 11 recognized major groups [12], were selected based on their prevalence in a pneumococcal carriage study in Massachusetts [17]. Two variants, var-I and var-II, contain choline-binding domains, and var-III (group 7) and var-IV (group 8) contain the LPXTG (sortase) motif. Truncated PspC constructs were designed to uniquely represent each PspC variant. Truncation removed the proline-rich and cell wall anchor motifs, which are highly homologous to those found in PspA (Supplementary Figure 1).
Serotype . | Age group . | . | . | |||||||
---|---|---|---|---|---|---|---|---|---|---|
<1 y . | 1–4 y . | 5–17 y . | >18 y . | Total . | % of Total . | |||||
19A | 5 | 2.07% | 7 | 2.89% | 0 | 0.00% | 10 | 4.13% | 22 | 9.07% |
22A | 4 | 1.65% | 10 | 4.13% | 4 | 1.65% | 3 | 1.24% | 21 | 8.67% |
35B | 6 | 2.48% | 6 | 2.48% | 3 | 1.24% | 6 | 2.48% | 21 | 8.67% |
23B | 2 | 0.83% | 8 | 3.31% | 3 | 1.24% | 4 | 1.65% | 17 | 7.02% |
15A | 1 | 0.41% | 7 | 2.89% | 2 | 0.83% | 3 | 1.24% | 13 | 5.37% |
34 | 0 | 0.00% | 6 | 2.48% | 1 | 0.41% | 6 | 2.48% | 13 | 5.36% |
6B | 1 | 0.41% | 4 | 1.65% | 2 | 0.83% | 5 | 2.07% | 12 | 4.95% |
11D | 2 | 0.83% | 3 | 1.24% | 4 | 1.65% | 2 | 0.83% | 11 | 4.54% |
23A | 1 | 0.41% | 4 | 1.65% | 1 | 0.41% | 4 | 1.65% | 10 | 4.13% |
31 | 2 | 0.83% | 3 | 1.24% | 3 | 1.24% | 0 | 0.00% | 8 | 3.31% |
16F | 0 | 0.00% | 2 | 0.83% | 4 | 1.65% | 2 | 0.83% | 8 | 3.30% |
17F | 2 | 0.83% | 0 | 0.00% | 2 | 0.83% | 4 | 1.65% | 8 | 3.30% |
37 | 2 | 0.83% | 0 | 0.00% | 1 | 0.41% | 4 | 1.65% | 7 | 2.89% |
6A | 0 | 0.00% | 3 | 1.24% | 3 | 1.24% | 0 | 0.00% | 6 | 2.48% |
21 | 0 | 0.00% | 3 | 1.24% | 2 | 0.83% | 1 | 0.41% | 6 | 2.48% |
35F | 2 | 0.83% | 2 | 0.83% | 0 | 0.00% | 2 | 0.83% | 6 | 2.48% |
7B | 1 | 0.41% | 1 | 0.41% | 0 | 0.00% | 4 | 1.65% | 6 | 2.47% |
10A | 0 | 0.00% | 3 | 1.24% | 1 | 0.41% | 1 | 0.41% | 5 | 2.06% |
15C | 1 | 0.41% | 1 | 0.41% | 0 | 0.00% | 3 | 1.24% | 5 | 2.06% |
3 | 0 | 0.00% | 1 | 0.41% | 2 | 0.83% | 1 | 0.41% | 4 | 1.65% |
35A | 0 | 0.00% | 3 | 1.24% | 0 | 0.00% | 1 | 0.41% | 4 | 1.65% |
NT | 2 | 0.83% | 1 | 0.41% | 0 | 0.00% | 1 | 0.41% | 4 | 1.65% |
10B | 0 | 0.00% | 1 | 0.41% | 2 | 0.83% | 0 | 0.00% | 3 | 1.24% |
7C | 0 | 0.00% | 1 | 0.41% | 1 | 0.41% | 1 | 0.41% | 3 | 1.24% |
1 | 0 | 0.00% | 1 | 0.41% | 0 | 0.00% | 2 | 0.83% | 3 | 1.24% |
19F | 1 | 0.41% | 0 | 0.00% | 1 | 0.41% | 0 | 0.00% | 2 | 0.83% |
22F | 0 | 0.00% | 1 | 0.41% | 1 | 0.41% | 0 | 0.00% | 2 | 0.83% |
38 | 0 | 0.00% | 1 | 0.41% | 1 | 0.41% | 0 | 0.00% | 2 | 0.83% |
12A | 0 | 0.00% | 1 | 0.41% | 0 | 0.00% | 1 | 0.41% | 2 | 0.82% |
12B | 0 | 0.00% | 1 | 0.41% | 0 | 0.00% | 1 | 0.41% | 2 | 0.82% |
15B | 0 | 0.00% | 0 | 0.00% | 1 | 0.41% | 0 | 0.00% | 1 | 0.41% |
5 | 0 | 0.00% | 0 | 0.00% | 1 | 0.41% | 0 | 0.00% | 1 | 0.41% |
7A | 1 | 0.41% | 0 | 0.00% | 0 | 0.00% | 0 | 0.00% | 1 | 0.41% |
8 | 0 | 0.00% | 1 | 0.41% | 0 | 0.00% | 0 | 0.00% | 1 | 0.41% |
9A | 0 | 0.00% | 1 | 0.41% | 0 | 0.00% | 0 | 0.00% | 1 | 0.41% |
10F | 0 | 0.00% | 0 | 0.00% | 0 | 0.00% | 1 | 0.41% | 1 | 0.41% |
Total | 36 | 14.9% | 87 | 36.0% | 46.0 | 19% | 73 | 30.2% | 242 | 100.00% |
Serotype . | Age group . | . | . | |||||||
---|---|---|---|---|---|---|---|---|---|---|
<1 y . | 1–4 y . | 5–17 y . | >18 y . | Total . | % of Total . | |||||
19A | 5 | 2.07% | 7 | 2.89% | 0 | 0.00% | 10 | 4.13% | 22 | 9.07% |
22A | 4 | 1.65% | 10 | 4.13% | 4 | 1.65% | 3 | 1.24% | 21 | 8.67% |
35B | 6 | 2.48% | 6 | 2.48% | 3 | 1.24% | 6 | 2.48% | 21 | 8.67% |
23B | 2 | 0.83% | 8 | 3.31% | 3 | 1.24% | 4 | 1.65% | 17 | 7.02% |
15A | 1 | 0.41% | 7 | 2.89% | 2 | 0.83% | 3 | 1.24% | 13 | 5.37% |
34 | 0 | 0.00% | 6 | 2.48% | 1 | 0.41% | 6 | 2.48% | 13 | 5.36% |
6B | 1 | 0.41% | 4 | 1.65% | 2 | 0.83% | 5 | 2.07% | 12 | 4.95% |
11D | 2 | 0.83% | 3 | 1.24% | 4 | 1.65% | 2 | 0.83% | 11 | 4.54% |
23A | 1 | 0.41% | 4 | 1.65% | 1 | 0.41% | 4 | 1.65% | 10 | 4.13% |
31 | 2 | 0.83% | 3 | 1.24% | 3 | 1.24% | 0 | 0.00% | 8 | 3.31% |
16F | 0 | 0.00% | 2 | 0.83% | 4 | 1.65% | 2 | 0.83% | 8 | 3.30% |
17F | 2 | 0.83% | 0 | 0.00% | 2 | 0.83% | 4 | 1.65% | 8 | 3.30% |
37 | 2 | 0.83% | 0 | 0.00% | 1 | 0.41% | 4 | 1.65% | 7 | 2.89% |
6A | 0 | 0.00% | 3 | 1.24% | 3 | 1.24% | 0 | 0.00% | 6 | 2.48% |
21 | 0 | 0.00% | 3 | 1.24% | 2 | 0.83% | 1 | 0.41% | 6 | 2.48% |
35F | 2 | 0.83% | 2 | 0.83% | 0 | 0.00% | 2 | 0.83% | 6 | 2.48% |
7B | 1 | 0.41% | 1 | 0.41% | 0 | 0.00% | 4 | 1.65% | 6 | 2.47% |
10A | 0 | 0.00% | 3 | 1.24% | 1 | 0.41% | 1 | 0.41% | 5 | 2.06% |
15C | 1 | 0.41% | 1 | 0.41% | 0 | 0.00% | 3 | 1.24% | 5 | 2.06% |
3 | 0 | 0.00% | 1 | 0.41% | 2 | 0.83% | 1 | 0.41% | 4 | 1.65% |
35A | 0 | 0.00% | 3 | 1.24% | 0 | 0.00% | 1 | 0.41% | 4 | 1.65% |
NT | 2 | 0.83% | 1 | 0.41% | 0 | 0.00% | 1 | 0.41% | 4 | 1.65% |
10B | 0 | 0.00% | 1 | 0.41% | 2 | 0.83% | 0 | 0.00% | 3 | 1.24% |
7C | 0 | 0.00% | 1 | 0.41% | 1 | 0.41% | 1 | 0.41% | 3 | 1.24% |
1 | 0 | 0.00% | 1 | 0.41% | 0 | 0.00% | 2 | 0.83% | 3 | 1.24% |
19F | 1 | 0.41% | 0 | 0.00% | 1 | 0.41% | 0 | 0.00% | 2 | 0.83% |
22F | 0 | 0.00% | 1 | 0.41% | 1 | 0.41% | 0 | 0.00% | 2 | 0.83% |
38 | 0 | 0.00% | 1 | 0.41% | 1 | 0.41% | 0 | 0.00% | 2 | 0.83% |
12A | 0 | 0.00% | 1 | 0.41% | 0 | 0.00% | 1 | 0.41% | 2 | 0.82% |
12B | 0 | 0.00% | 1 | 0.41% | 0 | 0.00% | 1 | 0.41% | 2 | 0.82% |
15B | 0 | 0.00% | 0 | 0.00% | 1 | 0.41% | 0 | 0.00% | 1 | 0.41% |
5 | 0 | 0.00% | 0 | 0.00% | 1 | 0.41% | 0 | 0.00% | 1 | 0.41% |
7A | 1 | 0.41% | 0 | 0.00% | 0 | 0.00% | 0 | 0.00% | 1 | 0.41% |
8 | 0 | 0.00% | 1 | 0.41% | 0 | 0.00% | 0 | 0.00% | 1 | 0.41% |
9A | 0 | 0.00% | 1 | 0.41% | 0 | 0.00% | 0 | 0.00% | 1 | 0.41% |
10F | 0 | 0.00% | 0 | 0.00% | 0 | 0.00% | 1 | 0.41% | 1 | 0.41% |
Total | 36 | 14.9% | 87 | 36.0% | 46.0 | 19% | 73 | 30.2% | 242 | 100.00% |
Serotype . | Age group . | . | . | |||||||
---|---|---|---|---|---|---|---|---|---|---|
<1 y . | 1–4 y . | 5–17 y . | >18 y . | Total . | % of Total . | |||||
19A | 5 | 2.07% | 7 | 2.89% | 0 | 0.00% | 10 | 4.13% | 22 | 9.07% |
22A | 4 | 1.65% | 10 | 4.13% | 4 | 1.65% | 3 | 1.24% | 21 | 8.67% |
35B | 6 | 2.48% | 6 | 2.48% | 3 | 1.24% | 6 | 2.48% | 21 | 8.67% |
23B | 2 | 0.83% | 8 | 3.31% | 3 | 1.24% | 4 | 1.65% | 17 | 7.02% |
15A | 1 | 0.41% | 7 | 2.89% | 2 | 0.83% | 3 | 1.24% | 13 | 5.37% |
34 | 0 | 0.00% | 6 | 2.48% | 1 | 0.41% | 6 | 2.48% | 13 | 5.36% |
6B | 1 | 0.41% | 4 | 1.65% | 2 | 0.83% | 5 | 2.07% | 12 | 4.95% |
11D | 2 | 0.83% | 3 | 1.24% | 4 | 1.65% | 2 | 0.83% | 11 | 4.54% |
23A | 1 | 0.41% | 4 | 1.65% | 1 | 0.41% | 4 | 1.65% | 10 | 4.13% |
31 | 2 | 0.83% | 3 | 1.24% | 3 | 1.24% | 0 | 0.00% | 8 | 3.31% |
16F | 0 | 0.00% | 2 | 0.83% | 4 | 1.65% | 2 | 0.83% | 8 | 3.30% |
17F | 2 | 0.83% | 0 | 0.00% | 2 | 0.83% | 4 | 1.65% | 8 | 3.30% |
37 | 2 | 0.83% | 0 | 0.00% | 1 | 0.41% | 4 | 1.65% | 7 | 2.89% |
6A | 0 | 0.00% | 3 | 1.24% | 3 | 1.24% | 0 | 0.00% | 6 | 2.48% |
21 | 0 | 0.00% | 3 | 1.24% | 2 | 0.83% | 1 | 0.41% | 6 | 2.48% |
35F | 2 | 0.83% | 2 | 0.83% | 0 | 0.00% | 2 | 0.83% | 6 | 2.48% |
7B | 1 | 0.41% | 1 | 0.41% | 0 | 0.00% | 4 | 1.65% | 6 | 2.47% |
10A | 0 | 0.00% | 3 | 1.24% | 1 | 0.41% | 1 | 0.41% | 5 | 2.06% |
15C | 1 | 0.41% | 1 | 0.41% | 0 | 0.00% | 3 | 1.24% | 5 | 2.06% |
3 | 0 | 0.00% | 1 | 0.41% | 2 | 0.83% | 1 | 0.41% | 4 | 1.65% |
35A | 0 | 0.00% | 3 | 1.24% | 0 | 0.00% | 1 | 0.41% | 4 | 1.65% |
NT | 2 | 0.83% | 1 | 0.41% | 0 | 0.00% | 1 | 0.41% | 4 | 1.65% |
10B | 0 | 0.00% | 1 | 0.41% | 2 | 0.83% | 0 | 0.00% | 3 | 1.24% |
7C | 0 | 0.00% | 1 | 0.41% | 1 | 0.41% | 1 | 0.41% | 3 | 1.24% |
1 | 0 | 0.00% | 1 | 0.41% | 0 | 0.00% | 2 | 0.83% | 3 | 1.24% |
19F | 1 | 0.41% | 0 | 0.00% | 1 | 0.41% | 0 | 0.00% | 2 | 0.83% |
22F | 0 | 0.00% | 1 | 0.41% | 1 | 0.41% | 0 | 0.00% | 2 | 0.83% |
38 | 0 | 0.00% | 1 | 0.41% | 1 | 0.41% | 0 | 0.00% | 2 | 0.83% |
12A | 0 | 0.00% | 1 | 0.41% | 0 | 0.00% | 1 | 0.41% | 2 | 0.82% |
12B | 0 | 0.00% | 1 | 0.41% | 0 | 0.00% | 1 | 0.41% | 2 | 0.82% |
15B | 0 | 0.00% | 0 | 0.00% | 1 | 0.41% | 0 | 0.00% | 1 | 0.41% |
5 | 0 | 0.00% | 0 | 0.00% | 1 | 0.41% | 0 | 0.00% | 1 | 0.41% |
7A | 1 | 0.41% | 0 | 0.00% | 0 | 0.00% | 0 | 0.00% | 1 | 0.41% |
8 | 0 | 0.00% | 1 | 0.41% | 0 | 0.00% | 0 | 0.00% | 1 | 0.41% |
9A | 0 | 0.00% | 1 | 0.41% | 0 | 0.00% | 0 | 0.00% | 1 | 0.41% |
10F | 0 | 0.00% | 0 | 0.00% | 0 | 0.00% | 1 | 0.41% | 1 | 0.41% |
Total | 36 | 14.9% | 87 | 36.0% | 46.0 | 19% | 73 | 30.2% | 242 | 100.00% |
Serotype . | Age group . | . | . | |||||||
---|---|---|---|---|---|---|---|---|---|---|
<1 y . | 1–4 y . | 5–17 y . | >18 y . | Total . | % of Total . | |||||
19A | 5 | 2.07% | 7 | 2.89% | 0 | 0.00% | 10 | 4.13% | 22 | 9.07% |
22A | 4 | 1.65% | 10 | 4.13% | 4 | 1.65% | 3 | 1.24% | 21 | 8.67% |
35B | 6 | 2.48% | 6 | 2.48% | 3 | 1.24% | 6 | 2.48% | 21 | 8.67% |
23B | 2 | 0.83% | 8 | 3.31% | 3 | 1.24% | 4 | 1.65% | 17 | 7.02% |
15A | 1 | 0.41% | 7 | 2.89% | 2 | 0.83% | 3 | 1.24% | 13 | 5.37% |
34 | 0 | 0.00% | 6 | 2.48% | 1 | 0.41% | 6 | 2.48% | 13 | 5.36% |
6B | 1 | 0.41% | 4 | 1.65% | 2 | 0.83% | 5 | 2.07% | 12 | 4.95% |
11D | 2 | 0.83% | 3 | 1.24% | 4 | 1.65% | 2 | 0.83% | 11 | 4.54% |
23A | 1 | 0.41% | 4 | 1.65% | 1 | 0.41% | 4 | 1.65% | 10 | 4.13% |
31 | 2 | 0.83% | 3 | 1.24% | 3 | 1.24% | 0 | 0.00% | 8 | 3.31% |
16F | 0 | 0.00% | 2 | 0.83% | 4 | 1.65% | 2 | 0.83% | 8 | 3.30% |
17F | 2 | 0.83% | 0 | 0.00% | 2 | 0.83% | 4 | 1.65% | 8 | 3.30% |
37 | 2 | 0.83% | 0 | 0.00% | 1 | 0.41% | 4 | 1.65% | 7 | 2.89% |
6A | 0 | 0.00% | 3 | 1.24% | 3 | 1.24% | 0 | 0.00% | 6 | 2.48% |
21 | 0 | 0.00% | 3 | 1.24% | 2 | 0.83% | 1 | 0.41% | 6 | 2.48% |
35F | 2 | 0.83% | 2 | 0.83% | 0 | 0.00% | 2 | 0.83% | 6 | 2.48% |
7B | 1 | 0.41% | 1 | 0.41% | 0 | 0.00% | 4 | 1.65% | 6 | 2.47% |
10A | 0 | 0.00% | 3 | 1.24% | 1 | 0.41% | 1 | 0.41% | 5 | 2.06% |
15C | 1 | 0.41% | 1 | 0.41% | 0 | 0.00% | 3 | 1.24% | 5 | 2.06% |
3 | 0 | 0.00% | 1 | 0.41% | 2 | 0.83% | 1 | 0.41% | 4 | 1.65% |
35A | 0 | 0.00% | 3 | 1.24% | 0 | 0.00% | 1 | 0.41% | 4 | 1.65% |
NT | 2 | 0.83% | 1 | 0.41% | 0 | 0.00% | 1 | 0.41% | 4 | 1.65% |
10B | 0 | 0.00% | 1 | 0.41% | 2 | 0.83% | 0 | 0.00% | 3 | 1.24% |
7C | 0 | 0.00% | 1 | 0.41% | 1 | 0.41% | 1 | 0.41% | 3 | 1.24% |
1 | 0 | 0.00% | 1 | 0.41% | 0 | 0.00% | 2 | 0.83% | 3 | 1.24% |
19F | 1 | 0.41% | 0 | 0.00% | 1 | 0.41% | 0 | 0.00% | 2 | 0.83% |
22F | 0 | 0.00% | 1 | 0.41% | 1 | 0.41% | 0 | 0.00% | 2 | 0.83% |
38 | 0 | 0.00% | 1 | 0.41% | 1 | 0.41% | 0 | 0.00% | 2 | 0.83% |
12A | 0 | 0.00% | 1 | 0.41% | 0 | 0.00% | 1 | 0.41% | 2 | 0.82% |
12B | 0 | 0.00% | 1 | 0.41% | 0 | 0.00% | 1 | 0.41% | 2 | 0.82% |
15B | 0 | 0.00% | 0 | 0.00% | 1 | 0.41% | 0 | 0.00% | 1 | 0.41% |
5 | 0 | 0.00% | 0 | 0.00% | 1 | 0.41% | 0 | 0.00% | 1 | 0.41% |
7A | 1 | 0.41% | 0 | 0.00% | 0 | 0.00% | 0 | 0.00% | 1 | 0.41% |
8 | 0 | 0.00% | 1 | 0.41% | 0 | 0.00% | 0 | 0.00% | 1 | 0.41% |
9A | 0 | 0.00% | 1 | 0.41% | 0 | 0.00% | 0 | 0.00% | 1 | 0.41% |
10F | 0 | 0.00% | 0 | 0.00% | 0 | 0.00% | 1 | 0.41% | 1 | 0.41% |
Total | 36 | 14.9% | 87 | 36.0% | 46.0 | 19% | 73 | 30.2% | 242 | 100.00% |
Genome Sequencing and Protein Antigen Identification
Genomic DNA from S. pneumoniae isolates were sequenced on the Illumina HiSeq to produce paired-end 100-basepair reads at ≥30-fold coverage. Serotypes were determined by mapping reads to concatenated capsule polysaccharide locus (CPS) sequences of 90 pneumococcal serotypes using SRST2 [18, 19]. De novo genome assemblies were generated with Velvet [20] and annotated using Prokka [21]. Pangenome analysis was conducted with Roary [22] to cluster and abstract protein antigen genes. The coding sequence for each protein antigen in the MSD assay was downloaded from KEGG (http://www.kegg.jp/), and orthologs from S. pneumoniae reference strains were identified (Table 1). The MSD index variant, S. pneumoniae reference orthologs, and de novo assembled protein antigen genes were aligned with PRANK using a codon-aware algorithm [23]. The diversity of each protein antigen among sequenced isolates was assessed to classify each antigen as polymorphic or conserved. Maximum likelihood phylogenies were inferred from the alignments using RAxML version 8.0.0. For polymorphic antigens, 1 reference variant was selected from each monophyletic clade and used to construct a protein database for SRST2 [19]. To determine the antigenic profile of each carried pneumococci, reads were mapped to each variant, and the highest scoring match was reported.
Statistical Analysis
To investigate correlation among participants’ antiprotein titers and association with age, we performed hierarchical clustering of 28 titers using the mclust v5.2 package in R. We then assessed the association between titers and subsequent carriage of a pneumococcus possessing alleles against which the participant had antibodies. For each polymorphic protein antigen, log antibody titers were compared among individuals carrying a pneumococcus with the respective variant. Statistical significance was assessed using analysis of variance and Tukey’s honest significant difference. For the pilus (RrgA/B), which is present in only a fraction of pneumococci, we assessed antipilus titers between individuals colonized with piliated and nonpiliated strains.
RESULTS
This analysis included 242 participants who had new pneumococcal colonization ≥30 days after serum collection (range = 30–225 days; median = 69). Individuals ranged in age from <1 month to 78 years and had 34 pneumococcal serotypes identified in addition to 4 nontypable isolates (Supplementary Table 2). For 2 participants, 2 serotypes were identified, and a single serotype was selected for whole-genome sequencing (WGS). Among participants, 14 carried pneumococci that were PCV-7 vaccine types, and the distribution of PCV-7 types did not significantly differ by age group (Fisher’s exact, P = .28).
Classification of Pneumococcal Protein Antigens
Phylogenetic analysis identified 11 polymorphic protein antigens and 10 that were largely conserved. Table 2 lists the variant frequencies for the 21 protein antigens. In some cases, the protein antigen variant was unable to be assigned; therefore, counts for some antigens do not total 242. We measured antibody titers to multiple variants of 3 proteins with polymorphisms (PspC, PspA, and RrgB). For the remaining 10 proteins, we measured antibody titers for 1 variant and compared titers among individuals with carriage isolates possessing polymorphic variants.
Protein Antigens and Variant Frequencies Identified Through Genomic Analysis of Pneumococcal Carriage Isolates
Polymorphic . | ||||||||
---|---|---|---|---|---|---|---|---|
Antigen . | . | Variant . | strain . | Accesion/gene . | Count . | Freq . | Average pairwise nucleotide distance p-distance . | |
I. | PspC | Pneumococcal surface protein C | Var-I* | ND6053 | ERR129207 | 203 | 83.9% | .03 (0.025-0.025) |
Var-II* | CH2016 | ERR129074 | 31 | 12.8% | .007 (0.006–0.008) | |||
Var-III* | BR1086 | ERR129054 | 3 | 1.2% | .005 (0.001–0.009) | |||
Var-IV* | MD5090 | ERR129180 | 1 | 0.4% | - | |||
II. | PspA | Pneumococcal surface protein A | Family 1* | D39 | SPD_0126 | 166 | 68.6% | .20 (0.199–0.201) |
Family 2* | TIGR4 | SP_0117 | 69 | 28.5% | ||||
Family 3 | BG6380 | AF071823 | 4 | 1.7% | ||||
III. | RrgA | RrgA pilus subunit, adhesin | Var-I* | TIGR4 | SP_0462 | 24 | 88.9% | .03 (0.027–0.037) |
Var-II | 670-6B | SP670_0540 | 3 | 11.1% | ||||
IV. | RrgB | RrgB pilus subunit, backbone | Var-I* | 670-6B | SP670_0541 | 4 | 13.8% | .22 (0.199–0.233) |
Var-II* | TIGR4 | SP_0463 | 11 | 37.9% | ||||
Var-III* | 23F_Taiwan_15 | EF560629: 5159–7123 | 14 | 48.3% | ||||
V. | NanA | Neuraminidase | Var-I* | D39 | SPD_1504 | 7 | 3.0% | .04 (0.041–0.042) |
Var-II | INV200 | SPNINV200_15140 | 75 | 32.2% | ||||
Var-III | ATCC 700669 | SPN23F16920 | 151 | 64.8% | ||||
VI. | SP0609 | Amino acid ABC transporter | Var-I* | TIGR4 | SPD_0530 | 161 | 66.8% | .02 (0.015–0.015) |
Var-II | ATCC 700669 | SPN23F05490 | 80 | 33.2% | ||||
VII. | SP2194 | ATP-dependent Clp protease | Var-I | Taiwan19F-14 | SPT_2213 | 222 | 91.7% | .01 (0.010–0.010) |
Var-II* | TIGR4 | SP_2194 | 20 | 8.3% | ||||
VIII. | PhtD | Pneumococcal histidine triad D | Var-I | ATCC 700669 | SPN23F09290 | 168 | 70.9% | .06 (0.060–0.064) |
Var-II* | D39 | SPD_0889 | 69 | 29.1% | ||||
IX. | StkP | Serine threonine kinase protein | Var-I | D39 | SPD_1542 | 181 | 74.8% | .007 (0.007–0.007) |
Var-II* | TIGR4 | SP_1732 | 61 | 25.2% | ||||
X. | StrH | Beta-N-acetylhexosaminidase | Var-I* | D39 | SPD_0063 | 101 | 42.1% | .007 (0.007–0.007) |
Var-II | TIGR4 | SP_0057 | 139 | 57.9% | ||||
XI. | Ply | Pneumolysin | Var-I* | D39 | SPD_1726 | 29 | 12.0% | .003 (0.003–0.003) |
Var-II* | TIGR4 | SP_1923 | 213 | 88.0% | ||||
Conserved | ||||||||
Antigen | Variant | strain | Accession/gene | - | - | |||
XII. | LysM | LysM domain-containing protein | Var-I* | TIGR4 | SP_0107 | - | - | .004 (0.003–0.004) |
XIII. | LytB | Endo-beta-N-acetylglucosaminidase | Var-I* | D39 | SPD_0853 | - | - | .008 (0.008–0.008) |
XIV. | LytC | Lysozyme (C-ter) | Var-I* | D39 | SPD_1403 | - | - | .005 (0.005–0.005) |
XV. | PcpA | Choline binding protein | Var-I* | D39 | SPD_1965 | - | - | .003 (0.003–0.003) |
XVI. | PcsB | Secreted 45 kDa protein | Var-I* | TIGR4 | SP_2216 | - | - | .006 (0.006–0.007) |
XVII. | PhtE | Truncated histidine triad protein | Var-I* | D39 | SPD_0890 | - | - | .008 (0.008–0.008) |
XVIII. | PiaA | Part of iron uptake ABC transporter | Var-I* | D39 | SPD_0915 | - | - | .002 (0.002–0.002 |
XIX. | PiuA | Part of iron uptake ABC transporter | Var-I* | D39 | SPD_1652 | - | - | .005 (0.005–0.005) |
XX. | PsaA | Pneumococcal surface adhesin A | Var-I* | TIGR4 | SP_1650 | - | - | .003 (0.002–0.003) |
XXI. | SP2027 | Conserved hypothetical protein | Var-I* | TIGR4 | SP_2027 | - | - | .005 (0.005–0.006) |
Polymorphic . | ||||||||
---|---|---|---|---|---|---|---|---|
Antigen . | . | Variant . | strain . | Accesion/gene . | Count . | Freq . | Average pairwise nucleotide distance p-distance . | |
I. | PspC | Pneumococcal surface protein C | Var-I* | ND6053 | ERR129207 | 203 | 83.9% | .03 (0.025-0.025) |
Var-II* | CH2016 | ERR129074 | 31 | 12.8% | .007 (0.006–0.008) | |||
Var-III* | BR1086 | ERR129054 | 3 | 1.2% | .005 (0.001–0.009) | |||
Var-IV* | MD5090 | ERR129180 | 1 | 0.4% | - | |||
II. | PspA | Pneumococcal surface protein A | Family 1* | D39 | SPD_0126 | 166 | 68.6% | .20 (0.199–0.201) |
Family 2* | TIGR4 | SP_0117 | 69 | 28.5% | ||||
Family 3 | BG6380 | AF071823 | 4 | 1.7% | ||||
III. | RrgA | RrgA pilus subunit, adhesin | Var-I* | TIGR4 | SP_0462 | 24 | 88.9% | .03 (0.027–0.037) |
Var-II | 670-6B | SP670_0540 | 3 | 11.1% | ||||
IV. | RrgB | RrgB pilus subunit, backbone | Var-I* | 670-6B | SP670_0541 | 4 | 13.8% | .22 (0.199–0.233) |
Var-II* | TIGR4 | SP_0463 | 11 | 37.9% | ||||
Var-III* | 23F_Taiwan_15 | EF560629: 5159–7123 | 14 | 48.3% | ||||
V. | NanA | Neuraminidase | Var-I* | D39 | SPD_1504 | 7 | 3.0% | .04 (0.041–0.042) |
Var-II | INV200 | SPNINV200_15140 | 75 | 32.2% | ||||
Var-III | ATCC 700669 | SPN23F16920 | 151 | 64.8% | ||||
VI. | SP0609 | Amino acid ABC transporter | Var-I* | TIGR4 | SPD_0530 | 161 | 66.8% | .02 (0.015–0.015) |
Var-II | ATCC 700669 | SPN23F05490 | 80 | 33.2% | ||||
VII. | SP2194 | ATP-dependent Clp protease | Var-I | Taiwan19F-14 | SPT_2213 | 222 | 91.7% | .01 (0.010–0.010) |
Var-II* | TIGR4 | SP_2194 | 20 | 8.3% | ||||
VIII. | PhtD | Pneumococcal histidine triad D | Var-I | ATCC 700669 | SPN23F09290 | 168 | 70.9% | .06 (0.060–0.064) |
Var-II* | D39 | SPD_0889 | 69 | 29.1% | ||||
IX. | StkP | Serine threonine kinase protein | Var-I | D39 | SPD_1542 | 181 | 74.8% | .007 (0.007–0.007) |
Var-II* | TIGR4 | SP_1732 | 61 | 25.2% | ||||
X. | StrH | Beta-N-acetylhexosaminidase | Var-I* | D39 | SPD_0063 | 101 | 42.1% | .007 (0.007–0.007) |
Var-II | TIGR4 | SP_0057 | 139 | 57.9% | ||||
XI. | Ply | Pneumolysin | Var-I* | D39 | SPD_1726 | 29 | 12.0% | .003 (0.003–0.003) |
Var-II* | TIGR4 | SP_1923 | 213 | 88.0% | ||||
Conserved | ||||||||
Antigen | Variant | strain | Accession/gene | - | - | |||
XII. | LysM | LysM domain-containing protein | Var-I* | TIGR4 | SP_0107 | - | - | .004 (0.003–0.004) |
XIII. | LytB | Endo-beta-N-acetylglucosaminidase | Var-I* | D39 | SPD_0853 | - | - | .008 (0.008–0.008) |
XIV. | LytC | Lysozyme (C-ter) | Var-I* | D39 | SPD_1403 | - | - | .005 (0.005–0.005) |
XV. | PcpA | Choline binding protein | Var-I* | D39 | SPD_1965 | - | - | .003 (0.003–0.003) |
XVI. | PcsB | Secreted 45 kDa protein | Var-I* | TIGR4 | SP_2216 | - | - | .006 (0.006–0.007) |
XVII. | PhtE | Truncated histidine triad protein | Var-I* | D39 | SPD_0890 | - | - | .008 (0.008–0.008) |
XVIII. | PiaA | Part of iron uptake ABC transporter | Var-I* | D39 | SPD_0915 | - | - | .002 (0.002–0.002 |
XIX. | PiuA | Part of iron uptake ABC transporter | Var-I* | D39 | SPD_1652 | - | - | .005 (0.005–0.005) |
XX. | PsaA | Pneumococcal surface adhesin A | Var-I* | TIGR4 | SP_1650 | - | - | .003 (0.002–0.003) |
XXI. | SP2027 | Conserved hypothetical protein | Var-I* | TIGR4 | SP_2027 | - | - | .005 (0.005–0.006) |
The antigen name and function are listed with the number of variants tested. Variants with measured titers are specified with an asterisk.
Protein Antigens and Variant Frequencies Identified Through Genomic Analysis of Pneumococcal Carriage Isolates
Polymorphic . | ||||||||
---|---|---|---|---|---|---|---|---|
Antigen . | . | Variant . | strain . | Accesion/gene . | Count . | Freq . | Average pairwise nucleotide distance p-distance . | |
I. | PspC | Pneumococcal surface protein C | Var-I* | ND6053 | ERR129207 | 203 | 83.9% | .03 (0.025-0.025) |
Var-II* | CH2016 | ERR129074 | 31 | 12.8% | .007 (0.006–0.008) | |||
Var-III* | BR1086 | ERR129054 | 3 | 1.2% | .005 (0.001–0.009) | |||
Var-IV* | MD5090 | ERR129180 | 1 | 0.4% | - | |||
II. | PspA | Pneumococcal surface protein A | Family 1* | D39 | SPD_0126 | 166 | 68.6% | .20 (0.199–0.201) |
Family 2* | TIGR4 | SP_0117 | 69 | 28.5% | ||||
Family 3 | BG6380 | AF071823 | 4 | 1.7% | ||||
III. | RrgA | RrgA pilus subunit, adhesin | Var-I* | TIGR4 | SP_0462 | 24 | 88.9% | .03 (0.027–0.037) |
Var-II | 670-6B | SP670_0540 | 3 | 11.1% | ||||
IV. | RrgB | RrgB pilus subunit, backbone | Var-I* | 670-6B | SP670_0541 | 4 | 13.8% | .22 (0.199–0.233) |
Var-II* | TIGR4 | SP_0463 | 11 | 37.9% | ||||
Var-III* | 23F_Taiwan_15 | EF560629: 5159–7123 | 14 | 48.3% | ||||
V. | NanA | Neuraminidase | Var-I* | D39 | SPD_1504 | 7 | 3.0% | .04 (0.041–0.042) |
Var-II | INV200 | SPNINV200_15140 | 75 | 32.2% | ||||
Var-III | ATCC 700669 | SPN23F16920 | 151 | 64.8% | ||||
VI. | SP0609 | Amino acid ABC transporter | Var-I* | TIGR4 | SPD_0530 | 161 | 66.8% | .02 (0.015–0.015) |
Var-II | ATCC 700669 | SPN23F05490 | 80 | 33.2% | ||||
VII. | SP2194 | ATP-dependent Clp protease | Var-I | Taiwan19F-14 | SPT_2213 | 222 | 91.7% | .01 (0.010–0.010) |
Var-II* | TIGR4 | SP_2194 | 20 | 8.3% | ||||
VIII. | PhtD | Pneumococcal histidine triad D | Var-I | ATCC 700669 | SPN23F09290 | 168 | 70.9% | .06 (0.060–0.064) |
Var-II* | D39 | SPD_0889 | 69 | 29.1% | ||||
IX. | StkP | Serine threonine kinase protein | Var-I | D39 | SPD_1542 | 181 | 74.8% | .007 (0.007–0.007) |
Var-II* | TIGR4 | SP_1732 | 61 | 25.2% | ||||
X. | StrH | Beta-N-acetylhexosaminidase | Var-I* | D39 | SPD_0063 | 101 | 42.1% | .007 (0.007–0.007) |
Var-II | TIGR4 | SP_0057 | 139 | 57.9% | ||||
XI. | Ply | Pneumolysin | Var-I* | D39 | SPD_1726 | 29 | 12.0% | .003 (0.003–0.003) |
Var-II* | TIGR4 | SP_1923 | 213 | 88.0% | ||||
Conserved | ||||||||
Antigen | Variant | strain | Accession/gene | - | - | |||
XII. | LysM | LysM domain-containing protein | Var-I* | TIGR4 | SP_0107 | - | - | .004 (0.003–0.004) |
XIII. | LytB | Endo-beta-N-acetylglucosaminidase | Var-I* | D39 | SPD_0853 | - | - | .008 (0.008–0.008) |
XIV. | LytC | Lysozyme (C-ter) | Var-I* | D39 | SPD_1403 | - | - | .005 (0.005–0.005) |
XV. | PcpA | Choline binding protein | Var-I* | D39 | SPD_1965 | - | - | .003 (0.003–0.003) |
XVI. | PcsB | Secreted 45 kDa protein | Var-I* | TIGR4 | SP_2216 | - | - | .006 (0.006–0.007) |
XVII. | PhtE | Truncated histidine triad protein | Var-I* | D39 | SPD_0890 | - | - | .008 (0.008–0.008) |
XVIII. | PiaA | Part of iron uptake ABC transporter | Var-I* | D39 | SPD_0915 | - | - | .002 (0.002–0.002 |
XIX. | PiuA | Part of iron uptake ABC transporter | Var-I* | D39 | SPD_1652 | - | - | .005 (0.005–0.005) |
XX. | PsaA | Pneumococcal surface adhesin A | Var-I* | TIGR4 | SP_1650 | - | - | .003 (0.002–0.003) |
XXI. | SP2027 | Conserved hypothetical protein | Var-I* | TIGR4 | SP_2027 | - | - | .005 (0.005–0.006) |
Polymorphic . | ||||||||
---|---|---|---|---|---|---|---|---|
Antigen . | . | Variant . | strain . | Accesion/gene . | Count . | Freq . | Average pairwise nucleotide distance p-distance . | |
I. | PspC | Pneumococcal surface protein C | Var-I* | ND6053 | ERR129207 | 203 | 83.9% | .03 (0.025-0.025) |
Var-II* | CH2016 | ERR129074 | 31 | 12.8% | .007 (0.006–0.008) | |||
Var-III* | BR1086 | ERR129054 | 3 | 1.2% | .005 (0.001–0.009) | |||
Var-IV* | MD5090 | ERR129180 | 1 | 0.4% | - | |||
II. | PspA | Pneumococcal surface protein A | Family 1* | D39 | SPD_0126 | 166 | 68.6% | .20 (0.199–0.201) |
Family 2* | TIGR4 | SP_0117 | 69 | 28.5% | ||||
Family 3 | BG6380 | AF071823 | 4 | 1.7% | ||||
III. | RrgA | RrgA pilus subunit, adhesin | Var-I* | TIGR4 | SP_0462 | 24 | 88.9% | .03 (0.027–0.037) |
Var-II | 670-6B | SP670_0540 | 3 | 11.1% | ||||
IV. | RrgB | RrgB pilus subunit, backbone | Var-I* | 670-6B | SP670_0541 | 4 | 13.8% | .22 (0.199–0.233) |
Var-II* | TIGR4 | SP_0463 | 11 | 37.9% | ||||
Var-III* | 23F_Taiwan_15 | EF560629: 5159–7123 | 14 | 48.3% | ||||
V. | NanA | Neuraminidase | Var-I* | D39 | SPD_1504 | 7 | 3.0% | .04 (0.041–0.042) |
Var-II | INV200 | SPNINV200_15140 | 75 | 32.2% | ||||
Var-III | ATCC 700669 | SPN23F16920 | 151 | 64.8% | ||||
VI. | SP0609 | Amino acid ABC transporter | Var-I* | TIGR4 | SPD_0530 | 161 | 66.8% | .02 (0.015–0.015) |
Var-II | ATCC 700669 | SPN23F05490 | 80 | 33.2% | ||||
VII. | SP2194 | ATP-dependent Clp protease | Var-I | Taiwan19F-14 | SPT_2213 | 222 | 91.7% | .01 (0.010–0.010) |
Var-II* | TIGR4 | SP_2194 | 20 | 8.3% | ||||
VIII. | PhtD | Pneumococcal histidine triad D | Var-I | ATCC 700669 | SPN23F09290 | 168 | 70.9% | .06 (0.060–0.064) |
Var-II* | D39 | SPD_0889 | 69 | 29.1% | ||||
IX. | StkP | Serine threonine kinase protein | Var-I | D39 | SPD_1542 | 181 | 74.8% | .007 (0.007–0.007) |
Var-II* | TIGR4 | SP_1732 | 61 | 25.2% | ||||
X. | StrH | Beta-N-acetylhexosaminidase | Var-I* | D39 | SPD_0063 | 101 | 42.1% | .007 (0.007–0.007) |
Var-II | TIGR4 | SP_0057 | 139 | 57.9% | ||||
XI. | Ply | Pneumolysin | Var-I* | D39 | SPD_1726 | 29 | 12.0% | .003 (0.003–0.003) |
Var-II* | TIGR4 | SP_1923 | 213 | 88.0% | ||||
Conserved | ||||||||
Antigen | Variant | strain | Accession/gene | - | - | |||
XII. | LysM | LysM domain-containing protein | Var-I* | TIGR4 | SP_0107 | - | - | .004 (0.003–0.004) |
XIII. | LytB | Endo-beta-N-acetylglucosaminidase | Var-I* | D39 | SPD_0853 | - | - | .008 (0.008–0.008) |
XIV. | LytC | Lysozyme (C-ter) | Var-I* | D39 | SPD_1403 | - | - | .005 (0.005–0.005) |
XV. | PcpA | Choline binding protein | Var-I* | D39 | SPD_1965 | - | - | .003 (0.003–0.003) |
XVI. | PcsB | Secreted 45 kDa protein | Var-I* | TIGR4 | SP_2216 | - | - | .006 (0.006–0.007) |
XVII. | PhtE | Truncated histidine triad protein | Var-I* | D39 | SPD_0890 | - | - | .008 (0.008–0.008) |
XVIII. | PiaA | Part of iron uptake ABC transporter | Var-I* | D39 | SPD_0915 | - | - | .002 (0.002–0.002 |
XIX. | PiuA | Part of iron uptake ABC transporter | Var-I* | D39 | SPD_1652 | - | - | .005 (0.005–0.005) |
XX. | PsaA | Pneumococcal surface adhesin A | Var-I* | TIGR4 | SP_1650 | - | - | .003 (0.002–0.003) |
XXI. | SP2027 | Conserved hypothetical protein | Var-I* | TIGR4 | SP_2027 | - | - | .005 (0.005–0.006) |
The antigen name and function are listed with the number of variants tested. Variants with measured titers are specified with an asterisk.
Protein Antigen Serology
Participants’ responses to different PspC variants were positively correlated (r = 0.29–0.90; P < .05 for all correlations), such that those with high PspC var-I titers also had high titers to the other PspC variants (Figure 1 and Supplementary Figure 2). Titers against 2 of the 3 RrgB variants were highly correlated with each other (0.96; P < .01) and less correlated with those against var-III (Figure 1 and Supplementary Figure 3). Among other protein antigens, we found significant correlation between variants of the same antigen (Figure 1). There was also high correlation among antigens, including PiuA, PiaA, PcsB, Spr2021, PcpA, CbpA, PhtE, and PhtD.
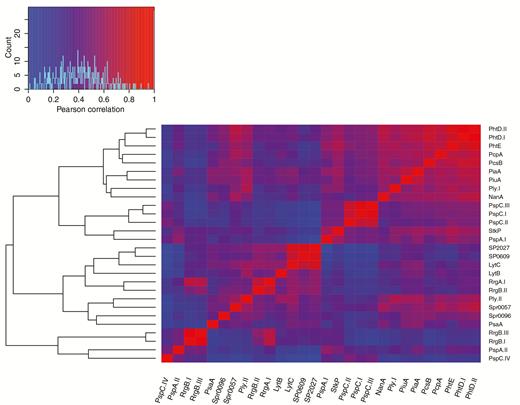
Pearson correlations of log10 antibody titers for 28 protein antigens clustered heuristically by correlation value. Correlations between normalized antibody titers of protein antigens were clustered using heuristic methods. Protein antigens, including multiple variants of the same antigen, are labeled on the x- and y-axes, and the heatmap displays the correlation values between antigens. The dendrogram on the left represents the results of the heuristic clustering of correlated antibody titers. Significant correlation between variants of the same antigen was observed, as well as high correlation among several antigens, which likely exist on the same genomic background.
Antibody titers for most proteins increased with age, including PspC variants (Figure 2A), PspA variants (Figure 2B), Ply variants (Figure 2D), and others (Supplementary Figure 4). Antipilus (RrgA/B) titers did not vary across pediatric ages; however, adults (aged ≥18 years) had significantly higher anti-RrgA/B titers compared with pediatric participants (aged <18 years; P < .001) (Figure 2C). For PspA (P < .01), StkP (P < .01) and PhtD (P < .01), participants aged 6–12 months and 12–24 months had lower titers than participatns aged <6 months. Among most protein antigens, the magnitudes of titers were comparable, with the exception of PspC var-IV titers, which were an order of magnitude lower across all ages compared with other PspC variants.
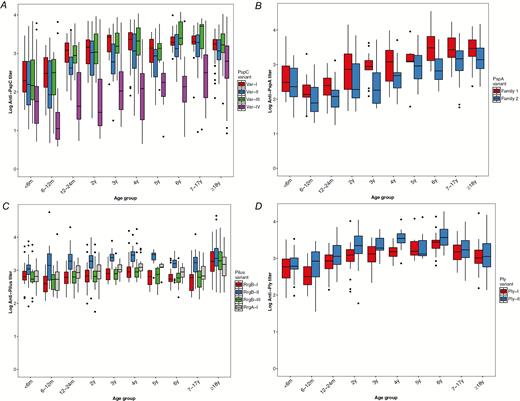
Protein antibody titers by age for variants of PspC, PspA, pilus, and ply. Antibody levels were measured using direct-binding electrochemiluminescence-based multiplex assay and are expressed as a titer relative to the amount in a reference serum. Structural variants of polymorphic proteins were measured individually and were compared among participant age groups. A, Variant-specific anti-PspC antibodies to var-I (ND6053), var-II (CH2016), var-III (BR1086), and var-IV (MD5090). B, Variant-specific anti-PspA antibodies to family 1 and family 2. C, Antipilus antibodies to RrgA-I (TIGR4) and RrgB pilus variants RrgB-I (670-6B), RrgB-II (Taiwan 23F), and RrgB-III (TIGR4). D, Variant-specific antipneumolysin (Ply) antibodies to variants I and II.
Hierarchical clustering of individual sera by the patterns of scaled antibody titers identified 2 clusters. The larger cluster (A) included substantial numbers of participants from all age groups, whereas cluster B was comprised largely of participants aged <5 years (86.8%) (Supplementary Figure 5). This cluster of pediatric participants had antibody titers below the population mean for a large proportion of protein antigens, compared with pediatric participants in cluster A, suggesting this population was either comparatively unexposed to pneumococcal protein antigens or unresponsive to them.
Analysis of Individual Protein Sequence Variation and Association With Antibody Titers
PspC
Among PspC variants, var-I was the most prevalent (84.1% of carriage isolates), followed by var-II (12%). Var-I corresponds to groups 2, 3, and 6 of PspC proteins based on sequence identity and structural organization, and var-II corresponds to group 4 (Table 1) [12]. The low prevalence of other PspC variants largely limited statistical comparison of anti-PspC titers to var-I and var-II. Because age may mediate pneumococcal carriage, we first investigated carriage of the PspC var-I by age group and found that it did not differ significantly between pediatric and adult participants (χ2 = 0.03; P = .87). Similarly, PspC variants II–IV did not significantly differ by age group. Anti-PspC titers did not differ significantly by pneumococcal carriage isolate variant for var-II (P = .18), var-III (P = .23), or var-IV (P = .53) (Figure 3A); however, differences in anti–var-I titers among participants carrying PspC variants approached statistical significance [F[3] = 2.41; P = .07]. We collapsed non–var-I PspC variants into a single category and compared titers between var-I and non–var-I. We found anti–var-I titers were significantly lower among individuals who went on to acquire strains possessing var-I (median log antibody titer 3.20 [var-I] vs 3.31 [non–var-I]; P = .02) (Figure 3B). This correlation was found in all age groups but only significant for participants aged <1 year (Supplementary Figure 6). In a logistic regression model controlling for age, low var-I titers were significantly associated with subsequent carriage of a strain carrying the var-I variant (odds ratio [OR] = 0.36; 95% confidence interval [CI] = .14–.81; P = .02). This suggests that low anti–var-I titers may be positively associated with acquisition of a strain possessing the PspC var-I.
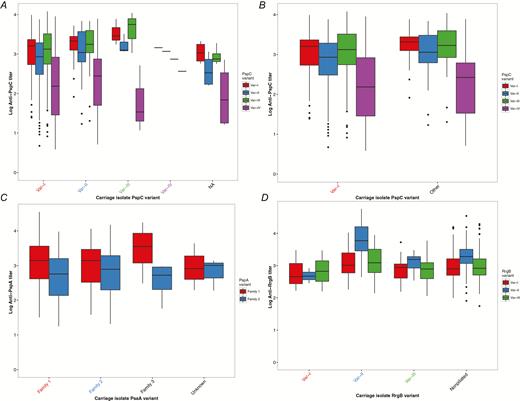
Protein antibody titers among participants carrying pneumococcal strains with specific polymorphic protein-antigen variants. Serum was collected from participants at enrollment, and nasopharyngeal swabs for pneumococcal carriage detection were collected at least 30 days after serum collection. The protein antigen variant in each pneumococcal isolate was determined using genomic data. Antibody levels were measured using direct-binding electrochemiluminescence-based multiplex assay and are expressed as a titer relative to the amount in a reference serum. Structural variants of polymorphic proteins were measured individually. The y-axis represents the variant-specific antibody titers, and the carriage isolate protein antigen variant is specified on the x-axis with labels colored to match the corresponding titers. If susceptibility to a strain possessing a specific variant were observed, the respective antibody titer would be the lowest among all other titers. A, Anti-PspC titers versus carriage isolate PspC variants I-IV and nontypable. B, Anti-PspC titers veresus carriage isolate PspC var-I and combined var-II–IV. C, Anti-PspA family 1 and family 2 titers versus carriage isolate PspA variants families 1, 2, 3, and unknown. D, Antipilus titers versus carriage isolate RrgB variants I–III and not present.
PspA
Among pneumococci in this sample, we identified all 3 families of PspA variants described by Hollingshead and colleagues [13]. Most isolates (70%) were family 1 PspA variant (Table 1). Additionally, 1% of strains (n = 3) were a PspA variant that formed an outgroup to families 1–3. Anti-PspA antibody titers were assessed among individuals carrying 4 PspA variants (family 1–3 and unknown). Among participants carrying pneumococcal strains with polymorphic PspA variants, anti-PspA titers for family 1 and 2 did not significantly differ (P = .53 and P = .62, respectively) (Figure 3C). Additionally, carriage of PspA variants was not found to vary by age.
Type 1 Pilus (RrgA-C)
Using the presence of RrgB as a marker, we found 11% of carriage strains were piliated (Table 1). Among piliated strains (n = 24), RrgB var-II was most common, followed closely by var-III. Piliated strains were most often serotype 35B (42.9%), 7B (21.4%), or 19A (14.3%). Carriage of a piliated pneumococcus did not significantly differ between pediatric and adult participants (χ2 = 0.49; P = .49). Anti-RrgB antibody titers of var-II and var-I did not significantly differ by carriage variant (P = .38 and P = .37, respectively) (Figure 3C). However, we found that anti-RrgB var-III titers were significantly higher (P < .001) among participants with subsequent carriage strains possessing var-III RrgB. This is contrary to what would be expected if higher variant specific titers were protective against colonization with respective strains. Anti-RrgA titers also did not significantly differ among carriage variants (P = .15). Last, we assessed anti-RrgB titers among carriage variants, comparing participants carrying piliated and nonpiliated strains. Anti-RrgB titers did not significantly differ among participants subsequently colonized with piliated or nonpiliated strains (Supplementary Figure 7).
Other Polymorphic Protein Antigens
For the polymorphic protein antigens NanA, SP0609, SP2194, PhtD, StkP, and StrH, where antibody titers were measured for only 1 variant, we compared titers among participants carrying pneumococci with heterologous antigen variants (Supplementary Figure 8). Distribution of NanA variants I–III did not differ between pediatric and adult participants (P = .32). Anti-NanA titers varied among participants (P = .08), with var-I titers marginally lower among participants carrying pneumococci with var-I. Interestingly, var-I was the least common, present in only 3.0% of carriage isolates. No statistically significant differences in antiprotein antibody titers were observed among the remaining polymorphic proteins. Additionally, with the exception of SP0609, for which the var-I was more prevalent among pediatric participants (P = .001), protein antigen variant frequencies did not differ by age group.
DISCUSSION
Protein-based vaccines aim to further reduce the morbidity of pneumococcal disease; however, a clearer understanding of the dynamics of protein antigen immunity and pneumococcal carriage is required. As with previous studies, we found that adult sera possessed antibodies to multiple antigens and to multiple variants of variable antigens and antibody titers usually increased across participant age groups, with adults having higher overall mean antiprotein titers [7, 14]. For PspA, StkP, and PhtD, a significant reduction in protein antibodies was observed between infants aged <6 months and children aged 6–24 months, likely resulting from the loss of maternally acquired antibodies and slower acquisition in infancy and toddlerhood than for other antigens. Although increasing natural immunity with age has been shown in previous studies of pneumococcal protein antigens [5, 7, 24], this is the first study to assess variant-specific antibodies and their effect on protection against homologous pneumococcal colonization. We found increased carriage of pneumococci expressing var-I PspC among participants with lower anti-PspC var-I titers. However, among all other protein antigens, including PspA, we found no difference in antiprotein antibody titers by subsequent colonization status with those variants. Because our study was designed to focus on variant-specific protection by serum antibody against colonization with a particular antigenic variant, we did not assess antibody levels in individuals who were subsequently not colonized with pneumococcus, so we were unable to assess the absolute level of protection against colonization associated with particular levels of serum antibodies. Our results suggest that whatever protection these antibodies offer, it is modestly variant specific for PspC and not measurably variant specific for other antigens.
PspC and PspA are structurally similar surface proteins [25] known for their interaction with the host immune system [26, 27], involvement in host epithelial adherence [28], and high recombination rates [17, 29]. Host immune evasion is often invoked to explain the apparent increased recombination rates, sequence diversity, and evidence of diversifying selection in pspA and pspC; however, there is no direct experimental evidence for this [30, 31]. Studies so far have implicated anti-PspC in protection against colonization, whereas anti-PspA seems important for protection against invasive disease [14]. The growing interest in both PspC and PspA as vaccine candidates underscores the need for better understanding and characterization of the nature of anti-PspA and anti-PspC immunity.
In this study, we investigated 4 variants of PspC, each representing 1 of the 11 recognized PspC groups [12]. Our data suggest there may exist specificity against colonization based on exposure history, where colonization with a rare PspC variant leaves individuals susceptible to colonization with the most prevalent circulating strain. This observation is consistent with the concept of balancing selection, in which the selective pressure of host immunity is sufficient to impact the frequency of specific PspC variants in the overall pneumococcal population. In general, this hypothesis is supported by the observation of high rates of recombination in the PspC locus, which may provide a way for pneumococci to obtain a PspC variant with low population-level host immunity. However, these underlying population dynamics require further exploration.
In prior studies, anti-PspA titers in children reflected the PspA family to which they had been exposed [14], whereas adults often possessed high titers to both major families. Among participants in this study, family 2 PspA variants were found in only 27% of carriage isolates, and mean anti-PspA family 2 variant titers were consistently lower among each age group. However, we found that anti-PspA titers were not predictive of the subsequently carried strain. In the context of previous studies demonstrating the protective role of anti-PspA antibodies, it appears that the cross-reactivity of antibodies may provide a broad level of protection from all PspA families.
The pneumococcal pilus, comprised of proteins RrgA, RrgB, and RrgC, facilitates binding to lung epithelial cells and colonization [32] and has been explored as a potential vaccine candidate [33]. The pilus operon has been shown to recombine and be acted on by positive selection [34]. Immunization with pilus subunits has been shown to provide protection against systemic challenge with piliated pneumococcal strains [35]. Previously estimated to be present in approximately 30% of pneumococci [36], pilus was present in only 11% of our studied strains. We found that, although antipilus antibody titers were significantly higher among adults compared with children, there was no difference in the proportion of carried strains that were piliated between these age groups. Furthermore, antipilus titers were not predictive of either carriage of piliated strains or RrgB variant among carried piliated strains. This was unexpected considering the high anti-RrgB titers in adults and the elevated RrgB var-III titers compared with variants I and II, which were less prevalent among piliated strains (Figure 2C). The low prevalence of piliated strains in the pneumococcal isolates from this study or variations in pilus expression [37] may have reduced our ability to detect differential pneumococcal carriage according to antibody titers.
Although for the majority of protein antigen variants we failed to find any variant-specific protective effect against carriage, it should be noted that immunity generated by naturally acquired antibodies is likely more complex than a simple variant-specific protective effect. Several prior studies have suggested some degree of cross-reactivity between antibodies to 1 variant of a protein and pneumococci carrying another. This could be consistent with subtle selective pressures imposed by modestly greater protection against homologous versus heterologous variants, which may be hard to measure in a host population. Furthermore immune responses against different proteins may have cumulative effects, such that the variant specificity of antibodies to a particular protein would be obscured when considering the ability of these antibodies to protect against a strain to which the individual may have many other effective antiprotein antibody responses. Indeed, challenge studies of protein-based vaccines in animal models have clearly shown that combination vaccines including 2–3 pneumococcal proteins are more efficacious for protection against invasive challenge [1, 2]. This likely reflects the in vivo interaction of host immunity with multiple pneumococcal antigens; however, this interaction is difficult to investigate. We observed a correlation of antiprotein titers among several antigen variants, suggesting that individuals likely possess a repertoire of antibodies to a specific set of protein antigen variants.
Certain limitations should be considered when interpreting our results. We selected genetic variants of protein antigens based on extant literature and phylogenetic analysis, identifying 1 strain from each monophyletic clade to represent a putatively antigenically distinct variant. However, each of these protein variants possesses several distinct epitopes, each likely generating a different antibody response. It is conceivable that antibodies against 1 protein antigen are in fact a mixture of antibodies of varying specificity, complicating the characterization of the overall response against 1 antigen. This is certainly a confounding factor in our analysis, and future studies should assess pneumococcal antiprotein antibody repertoires and functionally characterize distinct protein antigen variants (eg, PspC) to determine the nature of the antibody response they elicit and further our understanding of protection while informing vaccine development. Second, the exposure histories of participants are unknown, and participants were seemingly less likely to be exposed to rare protein variants in the pneumococcal population. Overall, using a large sample of pneumococcal strains and sera from those who carried these strains we found only modest evidence for variant specificity of protection against pneumococcal carriage.
Supplementary Data
Supplementary materials are available at The Journal of Infectious Diseases online. Consisting of data provided by the authors to benefit the reader, the posted materials are not copyedited and are the sole responsibility of the authors, so questions or comments should be addressed to the corresponding author.
Notes
Financial support. This work was supported by R01 R01AI048935, the Grand Challenges in Global Health initiative through the Bill & Melinda Gates Foundation, the Native American Research Centers for Health (U26IHS300013/03), the Centers for Disease Control and Prevention National Vaccine Program Office, and the Thrasher Research Fund (02820-9).
Potential conflicts of interest. All authors: No reported conflicts. All authors have submitted the ICMJE Form for Potential Conflicts of Interest. Conflicts that the editors consider relevant to the content of the manuscript have been disclosed.