-
PDF
- Split View
-
Views
-
Cite
Cite
Chirantana Sengupta, Oindrilla Mukherjee, Rukhsana Chowdhury, Adherence to Intestinal Cells Promotes Biofilm Formation in Vibrio cholerae, The Journal of Infectious Diseases, Volume 214, Issue 10, 15 November 2016, Pages 1571–1578, https://doi.org/10.1093/infdis/jiw435
- Share Icon Share
Abstract
Vibrio cholerae, the etiological agent of cholera, is known to form biofilms to persist in the environment. It is demonstrated here that even during infection, biofilm genes are upregulated, and microscopic observation indicated that biofilm formation is initiated almost immediately after adherence of V. cholerae to intestinal cells. About 7-fold upregulation of the biofilm regulatory gene vpsT was observed within 30 minutes of adherence of V. cholerae to the intestinal cell line INT 407, and a massive induction of about 700-fold was observed in rabbit ileal loops. The upregulation was observed in the classical and El Tor biotype strains of serogroup O1 that is most frequently associated with epidemic cholera. vpsT upregulation was primarily dependent on the virulence master regulator AphA. Of possible clinical relevance was the observation that V. cholerae in the INT 407–associated biofilms was significantly more resistant to antibiotics than unadhered planktonic cells.
Vibrio cholerae is a noninvasive, enteric bacterium that resides in aquatic ecosystems, from where it is transmitted to human hosts through contaminated food and water [1]. Subsequently, V. cholerae colonizes the small intestine and causes the diarrheal disease cholera that is estimated to cause 1.4 million–4.3 million cases and 28 000–142 000 deaths every year, mostly in developing nations [2, 3]. The pathogenicity of V. cholerae is largely due to the production of cholera toxin (CT) and a toxin-coregulated pilus (TCP) that are coordinately regulated by the hierarchical expression of several regulatory factors [4–7]. Other potential toxins and factors necessary for survival of V. cholerae in the intestine and evasion of the host defense system also contribute to the virulence of this important human pathogen [8].
For survival under the diverse environmental conditions found in the host intestine and in natural water reservoirs, V. cholerae has evolved several interesting strategies, some of which are common to bacteria that alternate between 2 lifestyles, while some are unique to V. cholerae [9]. For persistence in environmental water bodies, V. cholerae has been postulated to form biofilms in association with animate and inanimate surfaces. Colonization of chitinous surfaces of zooplankton is important for the persistence of V. cholerae in the aquatic environment, and V. cholerae has evolved several mechanisms that facilitate chitin colonization and biofilm formation [10–15]. In V. cholerae biofilms, polysaccharides (Vibrio polysaccharide [VPS]) and matrix proteins (RbmA, RbmC, and Bap1) are the major extracellular matrix components that encase the bacterial aggregate and attach biofilms to surfaces. Genes involved in VPS production are organized in 2 clusters in the V. cholerae genome, vps-1 (vpsA–vpsK) and vps-2 (vpsL–vpsQ), that are separated by a rbm cluster containing 6 genes encoding matrix proteins [16, 17]. Expression of the biofilm genes is regulated by a complex integrated network of transcriptional activators and repressors in response to multiple environmental signals [18]. VpsR and VpsT positively regulate expression of the vps and matrix protein genes and also regulate expression of each other [19, 20]. Expression of the biofilm genes is regulated positively by cyclic di-GMP and negatively by the quorum-sensing regulator HapR [21–25]. In contrast to the V. cholerae El Tor biotype strains that are predominantly HapR positive, strains like O395 that belong to the classical biotype carry a frameshift mutation in hapR, preventing quorum-sensing regulation of HapR-controlled genes [26, 27].
The role of biofilms in the environmental persistence and transmission of V. cholerae is well established [28]. V. cholerae biofilms in water reservoirs contain concentrated infectious doses of hyperinfective bacteria, the ingestion of which is associated with a high risk of disease [29]. When transmitted to the human body typically by ingestion of contaminated food and water, it has been hypothesized that the clumps of V. cholerae biofilms are disrupted and resuscitated to motile planktonic cells that adhere to intestinal epithelial cells. As infection progresses, V. cholerae are thought to detach from the epithelial cells and are shed back to the intestinal lumen, where physicochemical conditions favor biofilm formation. Finally, high concentrations of V. cholerae in the form of biofilms are shed by the patients. Moreover, these clumps are hyperinfectious and can revive into virulent bacteria in the human intestine, thus contributing to the rapid spread characteristic of cholera epidemics [30, 31]. Although several studies suggest that V. cholerae might form biofilms during infection [32, 33], the timing and regulation of biofilm formation in vivo during V. cholerae infection is still not clear, although it is generally believed that V. cholerae forms biofilms in the later stages of infection before exiting the human body [30].
In this study, we demonstrate that almost immediately after adherence of V. cholerae to the intestinal epithelial cell line INT 407, expression of biofilm genes and regulatory genes are strongly induced and biofilm formation is initiated. Massive induction of the regulatory gene vpsT was also observed in V. cholerae following infection in rabbit ileal loops. The genetic regulatory mechanism of vpsT upregulation has been investigated. Biofilm formation on the surface of INT 407 cells increased the resistance of V. cholerae to antibiotics.
MATERIALS AND METHODS
Bacterial strains and culture conditions, construction of V. cholerae mutants, INT 407 and HepG2 adherence assays, RNA isolation and reverse transcription–polymerase chain reaction, microscopy of V. cholerae infected cells, the antibiotic resistance assay, and ligated rabbit ileal loop assays were performed following standard procedures and are described in the Supplementary Materials.
RESULTS
Induction of Biofilm Genes in V. cholerae Adhered to the Intestinal Epithelial Cell Line INT 407
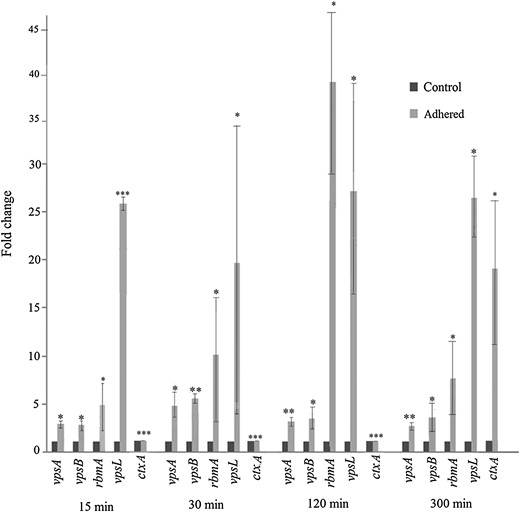
Induction of biofilm genes in INT 407–adhered Vibrio cholerae O395. RNA was extracted from V. cholerae O395 at different times after adherence to INT 407 cells (adhered) or from V. cholerae incubated under identical conditions without the cell line (control), and expression of vps and rbm was estimated by quantitative reverse transcription–polymerase chain reaction. ctxA expression was included as a control. Relative expression levels were calculated as fold induction of gene expression in adhered bacteria, considering the expression in control bacteria arbitrarily as 1. Error bars represent standard deviations. The statistical significance of differences in gene expression between the unadhered control and adhered V. cholerae was obtained by the Student t test. *P ≤ .05, **P ≤ .01, and ***P ≤ .001.
No significant difference in expression of the biofilm genes was observed between unadhered V. cholerae grown in Dulbecco's modified Eagle's medium (DMEM) without the cell line and bacteria isolated from the supernatant of infected INT 407 cells (data not shown), suggesting that biofilm gene induction required direct contact of V. cholerae with the INT 407 cells and was not due to a component secreted by the cell line.
Biofilm gene expression was next examined in V. cholerae adhered to a nonintestinal cell line. All biofilm genes examined were upregulated following adherence of V. cholerae to HepG2 cells (Supplementary Figure 1A). It was also noted that V. cholerae adhered to HepG2 with an efficiency comparable to that observed for INT 407 (data not shown). Interestingly, very little induction of biofilm genes was observed in V. cholerae adhered to a plastic surface (Supplementary Figure 1B). These results indicate that adherence to eukaryotic cells and not mere abiotic surface contact is responsible for the induction of biofilm genes in V. cholerae under the conditions used in this study.
Adherence to INT 407 Promotes V. cholerae Biofilm Formation
![Biofilm formation and antibiotic resistance of INT 407–adhered Vibrio cholerae O395. A, Representative CLSM Z-stack images of INT 407–adhered V. cholerae O395 at different times after adherence. B, The mean thickness (± standard deviation [SD]) of biofilms formed at different times after adherence. Results represent the average of at least 3 independent experiments. C, INT 407–adhered or unadhered V. cholerae O395 were treated with the antibiotics chloramphenicol (Cm), rifampicin (Rf), tetracycline (Tc), or azithromycin (Az) for 30 minutes or 60 minutes 2 hours after adherence, and the number of colony-forming unit (CFU) was estimated. The number of CFU is expressed relative to that of untreated V. cholerae, arbitrarily taken as 100. Error bars represent SDs. *P ≤ .05. Abbreviation: NS, not significant.](https://oup.silverchair-cdn.com/oup/backfile/Content_public/Journal/jid/214/10/10.1093_infdis_jiw435/3/m_jiw43502.jpeg?Expires=1748031579&Signature=WAu2WZakgqjV6Z0LIrukQzkeXLSRl1RzdHavJvo~dSQb0H5mZkMIz7NqJGsTS9F3Yu2dh6vD1PVFQhuMEURiDH5TxbselQzUK7FPnic9u4Go1wNOgrVYyxqqKzyu8Mwb02wnp23x2SMj3cea-YBaVZJdlk78W2lk53XXXQDo8JUNvkje5z7eRiOsiu70xJ43pX9xJ84DpZr77NCiC2q-~Qtv6BdR6e43-FDJXqDGJYG5fdSmvMhyUFa7G9vBvu-kCtSYCZaVB~RHW6ZEynCJ1dshkSQ9HoH4EkB5SOVo06TtfWXZPw1WgWnci9bjQ2vwGEiBo53xffEmmT6I~P4Unw__&Key-Pair-Id=APKAIE5G5CRDK6RD3PGA)
Biofilm formation and antibiotic resistance of INT 407–adhered Vibrio cholerae O395. A, Representative CLSM Z-stack images of INT 407–adhered V. cholerae O395 at different times after adherence. B, The mean thickness (± standard deviation [SD]) of biofilms formed at different times after adherence. Results represent the average of at least 3 independent experiments. C, INT 407–adhered or unadhered V. cholerae O395 were treated with the antibiotics chloramphenicol (Cm), rifampicin (Rf), tetracycline (Tc), or azithromycin (Az) for 30 minutes or 60 minutes 2 hours after adherence, and the number of colony-forming unit (CFU) was estimated. The number of CFU is expressed relative to that of untreated V. cholerae, arbitrarily taken as 100. Error bars represent SDs. *P ≤ .05. Abbreviation: NS, not significant.
Antibiotic Susceptibility
Bacteria residing in biofilm structures are often less susceptible to antibiotics than planktonic cells [34]. To examine whether biofilm formation on INT 407 cells conferred antibiotic resistance to adhered V. cholerae, the susceptibility of unadhered and INT 407–adhered V. cholerae to the antibiotics chloramphenicol, rifampicin, tetracycline, and azithromycin was assayed. The results indicated that INT 407–adhered V. cholerae was generally less susceptible to all antibiotics tested (Figure 2C). Treatment with chloramphenicol reduced the number of colony-forming units (CFU) of unadhered bacteria by >100-fold, and treatment with tetracycline and azithromycin decreased the number of CFU of unadhered bacteria by about 10-fold within 60 minutes. Treatment with these antibiotics, however, had practically no effect on viability of INT 407–adhered V. cholerae (Figure 2C). Rifampicin exerted a more drastic effect on V. cholerae viability. Upon treatment with rifampicin, the number of CFU of unadhered bacteria was reduced by 105–106-fold, whereas a more moderate decrease of 102–103-fold was observed in INT 407–adhered V. cholerae (Figure 2C). These results suggested that adherence to epithelial surfaces and biofilm formation increases antibiotic resistance of V. cholerae.
Expression of Biofilm Regulatory Genes in INT 407–Adhered V. cholerae
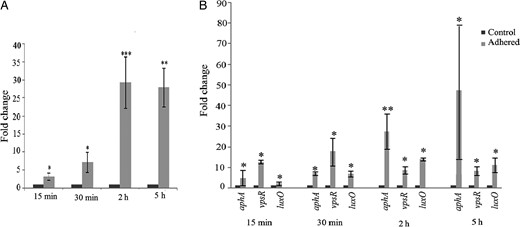
Induction of biofilm regulatory genes in INT 407–adhered Vibrio cholerae O395. RNA was extracted from V. cholerae O395 at different times after adherence to INT 407 cells (adhered) or from unadhered V. cholerae incubated without cell line (control), and expression of vpsT (A) or aphA, vpsR, and luxO (B) was estimated by quantitative reverse transcription–polymerase chain reaction. Relative expression levels were calculated as fold change of gene expression in adhered bacteria, considering the expression in unadhered control bacteria arbitrarily as 1. Error bars represent standard deviations. The statistical significance of differences in gene expression between the control and adhered V. cholerae was obtained by the Student t test. *P ≤ .05, **P ≤ .01, and ***P ≤ .001.
vpsT expression is controlled by a number of regulatory pathways involving the quorum-sensing regulator LuxO [21, 35], the virulence regulator AphA [36], and the biofilm regulator VpsR [20]. Although expression of all 3 regulatory genes was induced in INT 407–adhered V. cholerae, maximum induction of about 27-fold was observed in aphA expression (Figure 3B). To examine whether LuxO, VpsR, and AphA have a role in vpsT induction following adherence, deletion mutations were constructed in the corresponding genes. The ΔluxO, ΔvpsR, and ΔaphA mutants adhered to INT 407 with equal efficiency as the parental O395 WT strain (Supplementary Figure 3A). vpsT expression was next examined in the unadhered and INT 407–adhered mutant strains.
Role of the Virulence Master Regulator AphA in vpsT Induction in Adhered V. cholerae
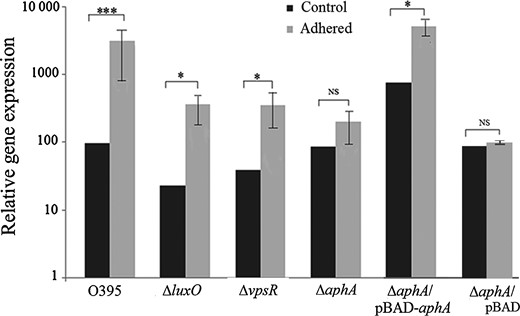
Expression of vpsT in ΔluxO, ΔvpsR, and ΔaphA mutants. RNA was extracted from O395, ΔluxO, ΔvpsR, ΔaphA ΔaphA/pBAD-aphA, and ΔaphA/pBAD 2 hours after adherence to INT 407 cells (adhered) or from unadhered bacteria incubated without the cell line (control), and expression of vpsT was estimated by quantitative reverse transcription–polymerase chain reaction. Relative expression levels were calculated as induction of gene expression in adhered bacteria, considering the expression in unadhered bacteria arbitrarily as 100. Error bars represent standard deviations. The statistical significance of differences in gene expression between the unadhered and adhered V. cholerae was obtained by the Student t test. *P ≤ .05 and ***P ≤ .001. Abbreviation: NS, not significant.
To further examine the role of LuxO, VpsR, and AphA in biofilm formation in INT 407–adhered V. cholerae, biofilms formed by ΔluxO, ΔvpsR, and ΔaphA mutants following adherence to INT 407 was examined. Extensive biofilm formation was observed in INT 407 adhered ΔluxO mutant, biofilm formation in the adhered ΔvpsR mutant was reduced, and practically no biofilm formation was observed in INT 407–adhered ΔaphA and ΔvpsT mutants (Supplementary Figure 4), confirming that AphA and VpsT are necessary for biofilm formation in INT 407–adhered V. cholerae, that VpsR also has a role, but that LuxO is not required for the process.
In unadhered bacteria, deletion of luxO and vpsR resulted in a decrease in vpsT expression (Figure 4), as has been reported earlier [20, 21]. However, practically no difference in vpsT expression was observed between WT and ΔaphA in vitro in unadhered bacteria (Figure 4), although elsewhere it was reported that AphA is a transcriptional activator of vpsT under laboratory conditions [36]. The reason for this discrepancy is not clear, although differences in strains and growth conditions may have an effect. Complementation of the ΔaphA mutant by ectopic expression of aphA from plasmid pBAD-aphA (Supplementary Figure 3B) increased vpsT expression both in unadhered and adhered bacteria (Figure 4).
Since it has been reported from in vitro studies that VpsR and LuxO are transcriptional activators of aphA [35, 36], the expression of aphA was examined in unadhered and INT 407–adhered ΔvpsR and ΔluxO mutants. Although in unadhered bacteria, aphA expression was significantly reduced in mutant strains as compared to the WT strain, induction of aphA in INT 407–adhered ΔvpsR and ΔluxO mutants as compared to unadhered bacteria was similar to that in the WT strain (Supplementary Figure 3C). These results suggested that VpsR and LuxO do not have a significant role in aphA induction in INT 407–adhered V. cholerae.
In Vivo Expression of vpsT
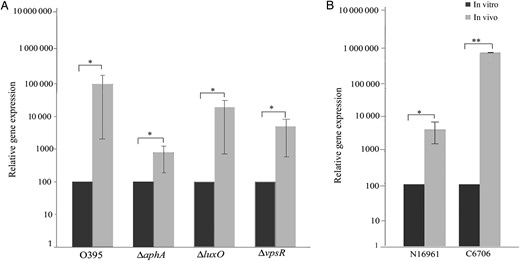
vpsT expression in ligated ileal loops. To examine the expression of vpsT in vivo, ligated rabbit ileal loops were inoculated with Vibrio cholerae strains O395, ΔluxO, ΔvpsR, and ΔaphA (A) or El Tor strains N16961 and C6706 (B). After 4 hours, bacteria were recovered from each loop, RNA was extracted, and vpsT expression was examined by quantitative reverse transcription–polymerase chain reaction. Relative expression levels were calculated as induction of gene expression in in vivo–grown bacteria, considering the expression in bacteria grown in vitro in LB medium arbitrarily as 100. Error bars represent standard deviations. The statistical significance of differences in gene expression between in vivo and in vitro grown bacteria was obtained by the Student t test. *P ≤ .1 and **P ≤ .01.
Expression of vpsT was also examined in the ΔluxO, ΔvpsR, and ΔaphA mutants grown in ligated rabbit ileal loops. vpsT upregulation was 3.5-fold lower in the ΔluxO mutant and 14-fold lower in the ΔvpsR mutant as compared to that in the WT bacteria (Figure 5A). However, induction of vpsT was much lower (86-fold) in the ΔaphA mutant (Figure 5A). These results suggested that AphA was required for complete vpsT upregulation in rabbit ileal loops, similar to the results obtained with INT 407–adhered V. cholerae. Although VpsR appears to have an additional effect, LuxO had relatively very little effect on host-dependent vpsT induction.
Induction of vpsT in V. cholerae El Tor Strains in Rabbit Ileal Loops
The V. cholerae O1 serogroup, most often associated with cholera epidemics, consists of 2 major biotypes, classical and El Tor [1]. Since expression of biofilm genes and the regulatory gene vpsT was strongly upregulated in the classical biotype strain O395 following adherence to intestinal cells or inoculation into rabbit intestine, attempts were made to examine vpsT expression in the El Tor biotype strains N16961 and C6706. However, vpsT expression in the strains N16961 and C6706 following adherence to INT 407 cells could not be estimated because extensive detachment and lysis of the INT 407 cells occurred within 30 minutes of adherence of the El Tor strains. Hence, the strains N16961 and C6706 were grown in vivo in ligated rabbit ileal loops, and vpsT expression was examined in bacteria recovered from the loops. Massive induction of vpsT (>1000-fold) was observed in strain C6706, while in strain N16961 vpsT expression was about 50-fold higher in rabbit intestine as compared to that in vitro when grown in LB medium (Figure 5B).
DISCUSSION
Environmental persistence of V. cholerae can be largely attributed to the ability of the organism to form biofilms preferentially on phytoplankton, zooplankton and chitin rain [12, 28]. Indeed, V. cholerae has served as a model organism for studying biofilm formation on animate and inanimate surfaces in environmental water reservoirs. However, some intriguing evidence has suggested that biofilms might have a role in the colonization by and pathogenesis of V. cholerae [30]. Mutants defective in biofilm formation exhibit significantly reduced intestinal colonization [33], and patches of biofilm like aggregates were observed along the villous axis and crypts following V. cholerae infection in rabbits [32]. In this study, expression of biofilm genes was examined in V. cholerae following adherence to the intestinal epithelial cell line INT 407 and also using the rabbit ligated ileal loop model. Expression of vps genes and the biofilm regulatory genes, particularly vpsT, was found to be strongly induced in the V. cholerae strains both in vitro, within 15 minutes after adherence of the bacteria to INT 407 cells (Figures 1 and 3), and in vivo in the rabbit intestine (Figure 5). The induction was observed in all V. cholerae strains examined in this study, which belong to the epidemic causing classical and El Tor biotypes. Induction required direct contact of the bacteria with the INT 407 cells and was not due to a component secreted by the cell line (data not shown). Furthermore, microscopic observation indicated that biofilm formation was initiated by 30 minutes in INT 407–adhered V. cholerae (Figure 2). Biofilm gene induction was also observed in a nonintestinal cell line (Supplementary Figure 1).
A number of different pathways involving different regulators and responding to different stimuli have been reported to be involved in V. cholerae biofilm formation [18, 28, 30]. The biofilm regulator vpsT, which was strongly induced following adherence of V. cholerae to INT 407 cells (Figure 3A), is known to be positively regulated by several regulators, including LuxO, VpsR, and AphA [20, 21, 35, 36]. While all these regulators are induced following adherence of V. cholerae to INT 407, immediate and maximum upregulation was observed for aphA (Figure 3B). Moreover, by use of ΔluxO and ΔvpsR mutants, we demonstrated that, although these regulators are required for vpsT expression in vitro, they had little or no effect on vpsT induction in INT 407–adhered V. cholerae (Figure 4) or V. cholerae grown in rabbit ileal loops (Figure 5). Significantly different results were obtained with the ΔaphA mutant. Relatively very little upregulation of vpsT expression was observed in the ΔaphA mutant following adherence to INT 407 cells (Figure 4) or when grown in rabbit intestine (Figure 5). Hence, it is reasonable to conclude that the transcriptional regulator required for intestinal cell contact–dependent upregulation of vpsT expression in V. cholerae is primarily AphA, VpsR has an additional effect, whereas LuxO has practically no effect. This is supported by microscopic observations of biofilm formation by INT 407–adhered ΔaphA, ΔvpsR, and ΔluxO mutants (Supplementary Figure 4).
Of particular interest and possible relevance to the infection process is the timing of biofilm initiation after adherence of V. cholerae to the intestinal cells. Expression of aphA and vpsT is upregulated almost immediately after contact of V. cholerae with the INT 407 cells (Figure 3), and biofilm initiation was observed by 30 minutes after adherence (Figure 2). However, it has been reported that, under similar conditions, the virulence genes were upregulated much later, at about 2 hours after adherence [38]. Taken together, these results suggest that biofilm formation by adhered V. cholerae actually precedes virulence expression. A probable reason could be that premature production of virulence factors, particularly the pilus TCP, before V. cholerae enters the mucous layer in the intestine could result in clumping of the bacteria in the intestinal lumen, which would permit V. cholerae to be easily flushed out. Early induction of biofilm genes would allow adherence of the bacteria to the mucosa before the virulence factors are expressed. It is possible that, during infection, V. cholerae cells initially use AphA to start biofilm production through the activation of vpsT expression, facilitating the bacterial colonization of intestinal surfaces. Furthermore, of great clinical significance is the observation that biofilm formation at early stages of infection would provide resistance to the infecting adhered bacteria against antimicrobial agents (Figure 2C).
Many studies performed so far have led to the postulation that V. cholerae enters the human body in the form of clumps of biofilms, which disperse into individual cells after reaching the intestine. Finally, V. cholerae again assembles into biofilm-like structures before exiting the human body [29–31]. The present study indicates that planktonic V. cholerae cells in the intestine may form biofilms on the epithelial surface through massive induction of vpsT and other biofilm genes. The induction of vpsT in animal infections by strains belonging to classical and El Tor biotypes examined was even higher than that observed in INT 407–adhered V. cholerae. It may be mentioned in this context that biofilm formation has also been reported to be highly activated upon entry of V. cholerae into the Drosophila intestine [39, 40]. The fate of the intestinal cell–associated V. cholerae biofilm at later stages of infection is as yet unknown and needs to be investigated.
Notes
Acknowledgments. We thank all members of the laboratory, for cooperation and helpful discussions; Kalidas Paul, for suggestions and excellent technical support; and Saurabh Bhattacharya, for help with animal experiments. C. S. G. thanks the Council of Scientific and Industrial Research.
Financial support. This work was supported by the Indian Institute of Chemical Biology, Council of Scientific and Industrial Research (research fellowship to C. S. G.).
Potential conflicts of interest. All authors: No reported conflicts. All authors have submitted the ICMJE Form for Disclosure of Potential Conflicts of Interest. Conflicts that the editors consider relevant to the content of the manuscript have been disclosed.
References