-
PDF
- Split View
-
Views
-
Cite
Cite
Caroline M. Lai, Karl W. Boehme, Andrea J. Pruijssers, Vrajesh V. Parekh, Luc Van Kaer, Charles A. Parkos, Terence S. Dermody, Endothelial JAM-A Promotes Reovirus Viremia and Bloodstream Dissemination, The Journal of Infectious Diseases, Volume 211, Issue 3, 1 February 2015, Pages 383–393, https://doi.org/10.1093/infdis/jiu476
- Share Icon Share
Abstract
Viruses that cause systemic disease often spread through the bloodstream to infect target tissues. Although viremia is an important step in the pathogenesis of many viruses, how viremia is established is not well understood. Reovirus has been used to dissect mechanisms of viral pathogenesis and is being evaluated in clinical trials as an oncolytic agent. After peroral entry into mice, reovirus replicates within the gastrointestinal tract and disseminates systemically via hematogenous or neural routes. Junctional adhesion molecule-A (JAM-A) is a tight junction protein that serves as a receptor for reovirus. JAM-A is required for establishment of viremia and viral spread to sites of secondary replication. JAM-A also is expressed on the surface of circulating hematopoietic cells. To determine contributions of endothelial and hematopoietic JAM-A to reovirus dissemination and pathogenesis, we generated strains of mice with altered JAM-A expression in these cell types and assessed bloodstream spread of reovirus strain type 1 Lang (T1L), which disseminates solely by hematogenous routes. We found that endothelial JAM-A but not hematopoietic JAM-A facilitates reovirus T1L bloodstream entry and egress. Understanding how viruses establish viremia may aid in development of inhibitors of this critical step in viral pathogenesis and foster engineering of improved oncolytic viral vectors.
Clinical manifestations of viral infections are often dictated by tropism of the virus for a particular cell type or organ. Many viruses spread systemically by bloodstream routes to reach sites at which disease is produced. Knowledge gained from studies to determine precisely how viruses disseminate can be used to block this key step in viral pathogenesis and improve vector targeting for clinical applications.
Mammalian orthoreoviruses (reoviruses) are nonenveloped, double-stranded RNA viruses that are being tested in phase 1–3 clinical trials as anticancer therapeutics [1]. Although reoviruses infect virtually all mammals, disease is limited to the very young [1]. Following peroral inoculation of newborn mice, reovirus replicates in the small intestine and systemically disseminates hematogenously or neurally, reaching high titers in most visceral organs [2–5]. Viremia is a well-established, essential step in reovirus pathogenesis [2]; however, how the virus gains entry into and amplifies to high titers within the bloodstream is unknown.
Reovirus engages 2 types of cellular receptors, sialylated glycans and junctional adhesion molecule-A (JAM-A), using attachment protein σ1 [6–11]. Reovirus binding to sialylated glycans enhances the neurovirulence of serotype 3 reovirus strains [12], whereas binding to JAM-A is required for establishment of viremia and subsequent bloodstream spread to sites of secondary replication of all reovirus serotypes [2]. However, JAM-A is not required for reovirus replication at sites of secondary replication [2]. JAM-A is a tight junction protein that serves to maintain barrier function of endothelium and epithelium [13–15]. JAM-A also is expressed on hematopoietic cells and platelets, promoting leukocyte extravasation from the bloodstream and platelet activation, respectively [13, 16–18]. Reovirus infection of polarized endothelial cells is dependent on engagement of both sialic acid and JAM-A [19]. Although JAM-A is required for establishment of reovirus viremia in mice [2], it is not known whether expression of JAM-A on endothelial cells, hematopoietic cells, or both promotes reovirus seeding of or exit from the vasculature.
To determine whether endothelial or hematopoietic JAM-A facilitates reovirus bloodstream dissemination, mice with diminished expression of endothelial cell-specific JAM-A (EndoJAM-AKD; knock-down [KD]) were established and infected by peroral and intravascular routes. Because EndoJAM-AKD mice also lack JAM-A expression in hematopoietic cells, mice lacking or expressing JAM-A solely in the hematopoietic cell compartment (HematoJAM-AKO [knock-out (KO)] and HematoJAM-A, respectively) also were established to determine whether hematopoietic JAM-A contributes to reovirus bloodstream spread. We found that EndoJAM-AKD mice had lower levels of viremia and lower viral titers at sites of secondary replication following either peroral or intravascular reovirus inoculation. In addition, HematoJAM-AKO mice had viral titers in blood and at sites of secondary replication similar to those observed in wild-type mice following either inoculation route. In contrast, HematoJAM-A mice had low levels of viremia and diminished titers at sites of secondary replication following peroral or intravascular reovirus inoculation. Together, these data suggest that endothelial JAM-A but not hematopoietic JAM-A is required for bloodstream dissemination of reovirus.
METHODS
Viruses and Cells
Murine L929 fibroblast cells were grown in suspension or monolayer cultures as described previously [3, 20]. Reovirus strain T1L is a laboratory stock. Strain rsT3D/T1LS1 was recovered by reverse genetics [3]. Virus was purified from infected L929 cells as described elsewhere [21]. Viral titers were determined by plaque assay using L929 cells [22].
Generation of Mouse Strains
Primer Sequences Used to Genotype Mouse Strains With Altered Junctional Adhesion Molecule-A Expressiona
Mouse Strain . | Region Amplified . | Forward Primer . | Reverse Primer . |
---|---|---|---|
JAM-A f/f (WT) | JAM-A Exon 1 | TCT TTT CAC CAA TCG GAA CG | AAA AAC TCT AGG AAC TCA CCC AGG A |
JAM-AKO (KO) | JAM-A Exon 1 | TCT TCT TCA GAC GCC GAA CCT | CCT CTC TTT TCA CCA ATC GGA |
Tek-Cre (EKD) | Tek P to Cre | CCC TGT GCT CAG ACA GAA ATG AGA | CGC ATA ACC AGT GAA ACA GCA TTG C |
Vav-Cre (HKO) | Vav P to Cre | GAA GGA ACG AGG GTG CAC | TGC CTG TCC CTG AAC ATG TC |
Vav-Cre (HKO) | Cre to Vav E | ATG CAG GCT GGT GGC TGG | GGC TCG CGA GGT TTT ACT TGC |
Vav-JAM (HJ) | Vav P to JAM-A | GAA GGA ACG AGG GTG CAC | GTG CAG GTC AAT TTG ATG GAC TCG |
Vav-JAM (HJ) | JAM-A to Vav E | CAG CTG TCC TGG TAA CAC TGA TTC | GGC TCG CGA GGT TTT ACT TGC |
Mouse Strain . | Region Amplified . | Forward Primer . | Reverse Primer . |
---|---|---|---|
JAM-A f/f (WT) | JAM-A Exon 1 | TCT TTT CAC CAA TCG GAA CG | AAA AAC TCT AGG AAC TCA CCC AGG A |
JAM-AKO (KO) | JAM-A Exon 1 | TCT TCT TCA GAC GCC GAA CCT | CCT CTC TTT TCA CCA ATC GGA |
Tek-Cre (EKD) | Tek P to Cre | CCC TGT GCT CAG ACA GAA ATG AGA | CGC ATA ACC AGT GAA ACA GCA TTG C |
Vav-Cre (HKO) | Vav P to Cre | GAA GGA ACG AGG GTG CAC | TGC CTG TCC CTG AAC ATG TC |
Vav-Cre (HKO) | Cre to Vav E | ATG CAG GCT GGT GGC TGG | GGC TCG CGA GGT TTT ACT TGC |
Vav-JAM (HJ) | Vav P to JAM-A | GAA GGA ACG AGG GTG CAC | GTG CAG GTC AAT TTG ATG GAC TCG |
Vav-JAM (HJ) | JAM-A to Vav E | CAG CTG TCC TGG TAA CAC TGA TTC | GGC TCG CGA GGT TTT ACT TGC |
In cases in which 2 sets of primers for a particular mouse strain are shown, only 1 was used at any time to determine the genotype.
Primer Sequences Used to Genotype Mouse Strains With Altered Junctional Adhesion Molecule-A Expressiona
Mouse Strain . | Region Amplified . | Forward Primer . | Reverse Primer . |
---|---|---|---|
JAM-A f/f (WT) | JAM-A Exon 1 | TCT TTT CAC CAA TCG GAA CG | AAA AAC TCT AGG AAC TCA CCC AGG A |
JAM-AKO (KO) | JAM-A Exon 1 | TCT TCT TCA GAC GCC GAA CCT | CCT CTC TTT TCA CCA ATC GGA |
Tek-Cre (EKD) | Tek P to Cre | CCC TGT GCT CAG ACA GAA ATG AGA | CGC ATA ACC AGT GAA ACA GCA TTG C |
Vav-Cre (HKO) | Vav P to Cre | GAA GGA ACG AGG GTG CAC | TGC CTG TCC CTG AAC ATG TC |
Vav-Cre (HKO) | Cre to Vav E | ATG CAG GCT GGT GGC TGG | GGC TCG CGA GGT TTT ACT TGC |
Vav-JAM (HJ) | Vav P to JAM-A | GAA GGA ACG AGG GTG CAC | GTG CAG GTC AAT TTG ATG GAC TCG |
Vav-JAM (HJ) | JAM-A to Vav E | CAG CTG TCC TGG TAA CAC TGA TTC | GGC TCG CGA GGT TTT ACT TGC |
Mouse Strain . | Region Amplified . | Forward Primer . | Reverse Primer . |
---|---|---|---|
JAM-A f/f (WT) | JAM-A Exon 1 | TCT TTT CAC CAA TCG GAA CG | AAA AAC TCT AGG AAC TCA CCC AGG A |
JAM-AKO (KO) | JAM-A Exon 1 | TCT TCT TCA GAC GCC GAA CCT | CCT CTC TTT TCA CCA ATC GGA |
Tek-Cre (EKD) | Tek P to Cre | CCC TGT GCT CAG ACA GAA ATG AGA | CGC ATA ACC AGT GAA ACA GCA TTG C |
Vav-Cre (HKO) | Vav P to Cre | GAA GGA ACG AGG GTG CAC | TGC CTG TCC CTG AAC ATG TC |
Vav-Cre (HKO) | Cre to Vav E | ATG CAG GCT GGT GGC TGG | GGC TCG CGA GGT TTT ACT TGC |
Vav-JAM (HJ) | Vav P to JAM-A | GAA GGA ACG AGG GTG CAC | GTG CAG GTC AAT TTG ATG GAC TCG |
Vav-JAM (HJ) | JAM-A to Vav E | CAG CTG TCC TGG TAA CAC TGA TTC | GGC TCG CGA GGT TTT ACT TGC |
In cases in which 2 sets of primers for a particular mouse strain are shown, only 1 was used at any time to determine the genotype.

Generation and characterization of mouse strains. A, A schematic depicting the establishment of EndoJAM-AKD and HematoJAM-AKO mice used in infection experiments. Exon 1 of junctional adhesion molecule-A (JAM-A) is flanked by loxP sites in JAM-A f/f mice. Cre recombinase expression in tissue-specific Cre tg mice is driven by Tek (endothelial) or Vav1 promoter and enhancer sequences from an inserted transgene. Crosses between JAM-A f/f and tissue-specific Cre mice generate mice in which exon 1 of JAM-A is excised in tissues where Cre is expressed. Arrows indicate primer-binding sites for JAM-A primers (J1, J2), JAM-A f/f primers (F1, F2), Tek-Cre primers (TC1, TC2), and Vav-Cre primers (VC1, VC2). B, Mice that express JAM-A only within the hematopoietic cell compartment (HematoJAM-A mice) were obtained by establishing tg mice expressing a transgene in which JAM-A expression is driven by Vav1 promoter and enhancer sequences. JAM-A expression in other tissues was abolished by breeding the tg mice with JAM-AKO mice. Arrows indicate primer-binding sites for Vav-JAM primers (VJ1, VJ2). C and D, JAM-A expression was assessed in 6- to 8-week-old mice by genomic DNA polymerase chain reaction (PCR) and flow cytometry. C, Agarose gels displaying bands corresponding to regions of genomic DNA amplified in genotyping PCR reactions. Bands from the following reactions are shown for each mouse strain: JAM-A f/f, Tek-Cre, Vav-Cre, Vav-JAM, and JAM-AKO. D, Hematopoietic cells were collected from blood and spleens, and endothelial cells were cultured from lungs. JAM-A expression was quantified using flow cytometry. Flow cytometric profiles of endothelial and hematopoietic cells (peripheral blood granulocytes and macrophages) from each mouse strain. Abbreviations: CRE, Cre recombinase; E, enhancer; EKD, EndoJAM-AKD; HJ, HematoJAM-A; HKO, HematoJAM-AKO; JAM, JAM-A coding sequence; KO, JAM-AKO; PRO, promoter; US, unstained; Vav, Vav promoter; WT, wild-type (JAM-A f/f).
Mouse Infection Studies
Two- to 3-day-old mice were inoculated perorally or intravenously with purified reovirus strain T1L. Peroral inoculations were performed as described previously [2, 3]. Intravenous inoculations were performed following anesthesia by hypothermia [25, 26]. Anesthetized mice were positioned on a transilluminator (Phillips) to visualize the superficial temporal vein. A dose of 50 μL of virus inoculum was administered via a 33-gauge 0.25-inch needle (Cadence). At defined intervals post-inoculation, mice were euthanized and organs were excised, submerged into phosphate-buffered saline (PBS), subjected to 2 freeze–thaw cycles, and sonicated until homogenized. Viral titers in organ homogenates were determined by plaque assay [22]. Blood was collected into an equal volume of Alsever's solution (Sigma), subjected to 2 freeze–thaw cycles, sonicated, and processed for viral titer determination by plaque assay [22].
Animal husbandry and experimental procedures were performed in accordance with United States Public Health Service policy on Humane Care and Use of Laboratory Animals and approved by the Vanderbilt University School of Medicine Institutional Animal Care and Use Committee.
Assessment of Hematopoietic JAM-A Expression by Flow Cytometry
Hematopoietic cells were harvested from peripheral blood or spleens of 6- to 8-week-old mice. Erythrocytes were lysed using ammonium–chloride–potassium lysis buffer (Invitrogen) at room temperature for 5 minutes. Leukocytes were collected by centrifugation at 1000 × g for 5 minutes, and hematopoietic cell subsets were identified using antibodies specific for granulocytes (Gr-1), B cells (B220), T cells (TCRβ), macrophages (CD11b), and dendritic cells (CD11c). Expression of cell-surface JAM-A was assessed using antibody AF1077 (R&D Systems). Reovirus binding to or infectivity of hematopoietic cells was assessed using Alexa Fluor–conjugated reovirus-specific antiserum [27]. For infectivity studies, cells were fixed in 1% paraformaldehyde either prior to or after permeabilization using Cytofix/Cytoperm (BD Biosciences) prior to addition of Alexa Fluor–conjugated reovirus-specific antiserum as described elsewhere [28, 29]. The threshold level of detection for infected cells using this assay is ∼3% [19].
Assessment of Endothelial JAM-A Expression by Flow Cytometry
Primary lung endothelial cells [30] were cultured at 37°C for 5–7 days in EBM-2 medium supplemented to contain EGM-2 MV SingleQuots (human epidermal growth factor, hydrocortisone, gentamicin, amphotericin B, vascular endothelial growth factor, human fibroblast growth factor-B, insulin-like growth factor-1, ascorbic acid, and heparin; Lonza), washed twice with PBS on day 3, and supplemented with fresh EBM-2 medium. Expression of cell-surface JAM-A was assessed using flow cytometry following staining of cells with antibodies specific for hematopoietic cells (CD45), endothelial cells (CD31), and JAM-A. All cell staining was quantified using FlowJo software (Tree Star).
Brain Vascular Permeability Studies
Two- to 3-day-old mice were inoculated perorally with 5 × 106 plaque-forming units (PFU) of reovirus T1L or PBS as a negative control. Four or 8 days post-inoculation, mice were inoculated intraperitoneally with 100 μL of 10% sodium fluorescein (Sigma). Ten minutes later, mice were euthanized using isoflurane and perfused. Blood was collected, and brains were excised and flash frozen. To quantify sodium fluorescein in the tissue, brains were thawed and homogenized, and an aliquot of brain homogenate was mixed with 7.5% trichloroacetic acid (Sigma). Sodium fluorescein fluorescence in the sample was quantified using a Synergy HT multi-mode microplate reader (BioTek) with 485 nm excitation and 528 nm emission filters. Fluorescein concentration was determined from the fluorescein isothiocyanate fluorescence intensity using a standard curve generated with known concentrations of sodium fluorescein. Mice were inoculated with 1 mg/kg lipopolysaccharide (LPS; Sigma) 3 hours prior to euthanasia as a positive control.
Ex Vivo Infection of Lymphocytes
Splenocytes were isolated from spleens of 5-day-old mice, stained with antibodies specific for dendritic cells (CD11c), macrophages (CD11b), T cells (CD3), and B cells (B220), and separated using fluorescence-activated cell sorting (FACS).
Cells were adsorbed with rsT3D/T1LS1 at a multiplicity of infection of 100 PFU/cell on ice for 1 hour. Cells were washed once with FACS buffer, resuspended in lymphocyte medium (Roswell Park Memorial Institute 1640 medium supplemented to contain 10% fetal bovine serum, 1% penicillin/streptomycin, 1% HEPES (pH 7.4), 1% sodium pyruvate, 1% l-glutamine, and 0.1% β-mercaptoethanol) and incubated at 37°C. Cells were collected at 0 and 24 hours post-adsorption and subjected to 2 freeze–thaw cycles; viral titers in cell lysates were determined by plaque assay.
RESULTS
Characterization of Mice With Targeted Disruption of JAM-A Expression
To determine the role of endothelial JAM-A in reovirus infection, we established mice with diminished JAM-A expression in the endothelial cell compartment (Figure1). We used flow cytometry to assess expression of JAM-A on pulmonary vascular endothelial cells and hematopoietic cells collected from EndoJAM-AKD mice. We found that expression of cell-surface JAM-A was decreased on endothelial cells in these mice and absent on hematopoietic cells (Figure1D and Supplementary Data). Compared with that seen in wild-type mice, endothelial JAM-A expression in EndoJAM-AKD mice was diminished approximately 2-fold (Figure1D). It is possible that JAM-A expression on other types of endothelial cells is diminished to a greater degree.
To determine the role of hematopoietic JAM-A in reovirus infection, we established mice that either lack or express JAM-A solely in the hematopoietic compartment (Figure1A and 1B). We assessed endothelial and hematopoietic JAM-A expression in HematoJAM-AKO and HematoJAM-A mice using flow cytometry. As expected, endothelial JAM-A expression in HematoJAM-AKO mice mirrored that seen in wild-type endothelial cells (Figure1D), whereas endothelial JAM-A expression in HematoJAM-A mice was similar to that observed in JAM-AKO endothelial cells (Table1, Figure1D). JAM-A expression on hematopoietic cells collected from HematoJAM-AKO mice was undetectable, but hematopoietic JAM-A expression in HematoJAM-A mice was identical to that seen in wild-type mice (Figure1D and Supplementary Data). The JAM-A expression phenotypes of the mouse strains used in this study are summarized in Table2.
Junctional Adhesion Molecule-A Expression in Mouse Strains Used in Studies of Reovirus Bloodstream Spread
Mouse Strain . | Junctional Adhesion Molecule-A Expression . | |
---|---|---|
Endothelial Cells . | Hematopoietic Cells . | |
JAM-A f/f (WT) | ++a | ++ |
JAM-A KO (KO) | –b | – |
EndoJAM-A KD (EKD) | +c | – |
HematoJAM-A KO (HKO) | ++ | – |
Hemato-JAM-A (HJ) | – | ++ |
Mouse Strain . | Junctional Adhesion Molecule-A Expression . | |
---|---|---|
Endothelial Cells . | Hematopoietic Cells . | |
JAM-A f/f (WT) | ++a | ++ |
JAM-A KO (KO) | –b | – |
EndoJAM-A KD (EKD) | +c | – |
HematoJAM-A KO (HKO) | ++ | – |
Hemato-JAM-A (HJ) | – | ++ |
Wild-type expression.
Absent expression.
Expression decreased approximately 50%.
Junctional Adhesion Molecule-A Expression in Mouse Strains Used in Studies of Reovirus Bloodstream Spread
Mouse Strain . | Junctional Adhesion Molecule-A Expression . | |
---|---|---|
Endothelial Cells . | Hematopoietic Cells . | |
JAM-A f/f (WT) | ++a | ++ |
JAM-A KO (KO) | –b | – |
EndoJAM-A KD (EKD) | +c | – |
HematoJAM-A KO (HKO) | ++ | – |
Hemato-JAM-A (HJ) | – | ++ |
Mouse Strain . | Junctional Adhesion Molecule-A Expression . | |
---|---|---|
Endothelial Cells . | Hematopoietic Cells . | |
JAM-A f/f (WT) | ++a | ++ |
JAM-A KO (KO) | –b | – |
EndoJAM-A KD (EKD) | +c | – |
HematoJAM-A KO (HKO) | ++ | – |
Hemato-JAM-A (HJ) | – | ++ |
Wild-type expression.
Absent expression.
Expression decreased approximately 50%.
Endothelial JAM-A Promotes Bloodstream Dissemination of Reovirus
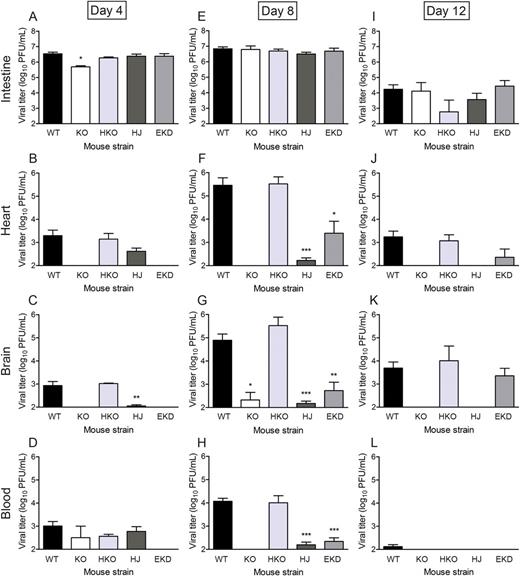
Endothelial junctional adhesion molecule-A is required for reovirus bloodstream spread. Newborn (2–3 days) mice were inoculated perorally with reovirus strain T1L at 1000 PFU per mouse. At 4, 8, and 12 days post-inoculation, intestine, heart, and brain were excised, and blood was collected into an equal volume of Alsever's solution for determination of viral titer by plaque assay. Results are presented as mean viral titer. Error bars indicate standard deviation. For each time point and mouse strain, 3 to 13 mice were used. Abbreviations: EKD, EndoJAM-AKD; HJ, HematoJAM-A; HKO, HematoJAM-AKO; KO, JAM-AKO; WT, wild-type (JAM-A f/f). *P < .05, **P < .005, ***P < .001 by Student t test.
Endothelial JAM-A is Required for Reovirus Egress From the Bloodstream
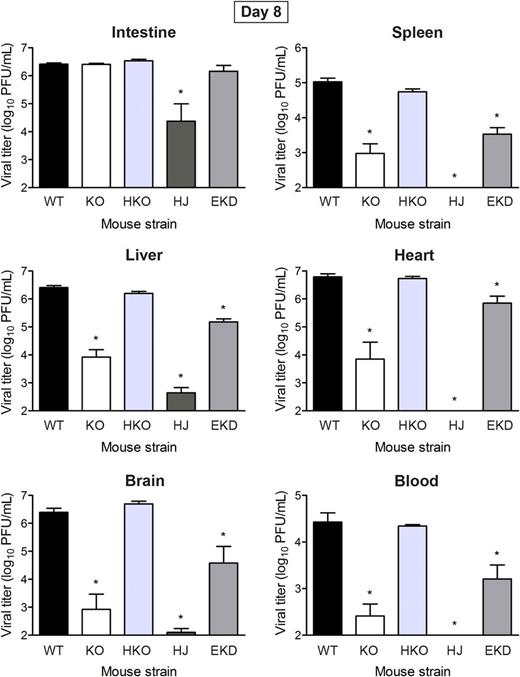
Endothelial junctional adhesion molecule-A is required for reovirus egress from the circulation. Newborn (2–3 days) mice were inoculated intravenously with reovirus strain T1L at 1000 PFU per mouse. At 8 days post-inoculation, intestine, spleen, liver, heart, and brain were excised, and blood was collected into an equal volume of Alsever's solution for determination of viral titer by plaque assay. Results are presented as mean viral titer. Error bars indicate standard deviation. For each time point and mouse strain, 5 to 18 mice were used. Abbreviations: EKD, EndoJAM-AKD; HJ, HematoJAM-A; HKO, HematoJAM-AKO; KO, JAM-AKO; WT, wild-type (JAM-A f/f). *P < .05 by Student t test.
To determine whether endothelial or hematopoietic JAM-A facilitates reovirus egress from the bloodstream, EndoJAM-AKD, HematoJAM-AKO, and HematoJAM-A mice were inoculated intravenously, and viral titers were determined in organ homogenates 8 days post-inoculation. Reovirus titers in HematoJAM-AKO mice were comparable to those in wild-type mice (Figure3), suggesting that reovirus egress from the bloodstream does not require hematopoietic JAM-A. Viral titers in the intestine, spleen, liver, heart, and brain of HematoJAM-A mice were similar to those in JAM-AKO mice (Figure3), suggesting that hematopoietic JAM-A is not sufficient for reovirus exit from the circulation and infection of those organs. Viral titers in EndoJAM-AKD mice were significantly lower in the spleen, liver, heart, brain, and blood compared with those in wild-type mice (Figure3). Taken together, these data suggest that reovirus egress from the bloodstream requires endothelial JAM-A but not hematopoietic JAM-A.
Reovirus Infection Does Not Increase Vascular Permeability in the Brain
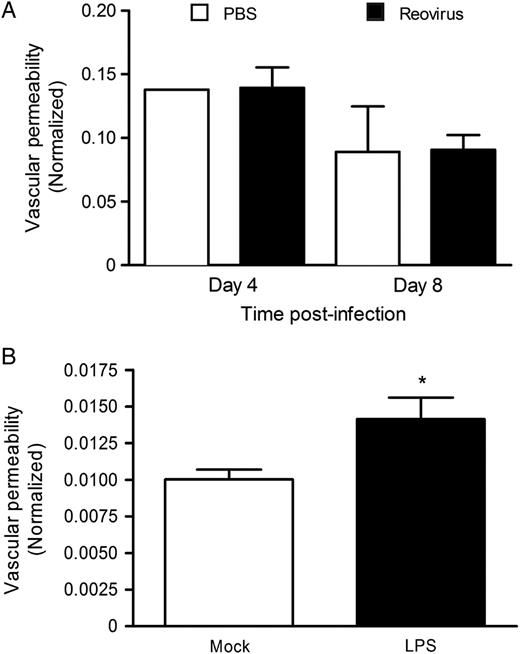
Reovirus infection does not increase vascular permeability in vivo. A, Newborn (2–3 days) wild-type mice were inoculated perorally with either phosphate-buffered saline (PBS) or reovirus strain T1L at 5 × 106 PFU per mouse. At 4 and 8 days post-inoculation, mice were injected intraperitoneally with 0.1 mL 10% sodium fluorescein. B, Mice (7–8 days) were inoculated with 1 mg/kg lipopolysaccharide (LPS) and 3 hours later injected intraperitoneally with 0.1 mL 10% sodium fluorescein. Ten minutes later, all mice were euthanized and perfused. Brains were homogenized, and fluorescein content was determined using a microplate reader with 485 nm excitation and 528 nm emission filters. Fluorescein content in blood was used to normalize the fluorescein content in the brain. In the PBS- and LPS-treated groups, 2 to 3 mice were used; in the reovirus-infected group, 5 mice were used at both time points. *P < .05 by Student t test.
Reovirus Does Not Bind to or Infect Hematopoietic Cells
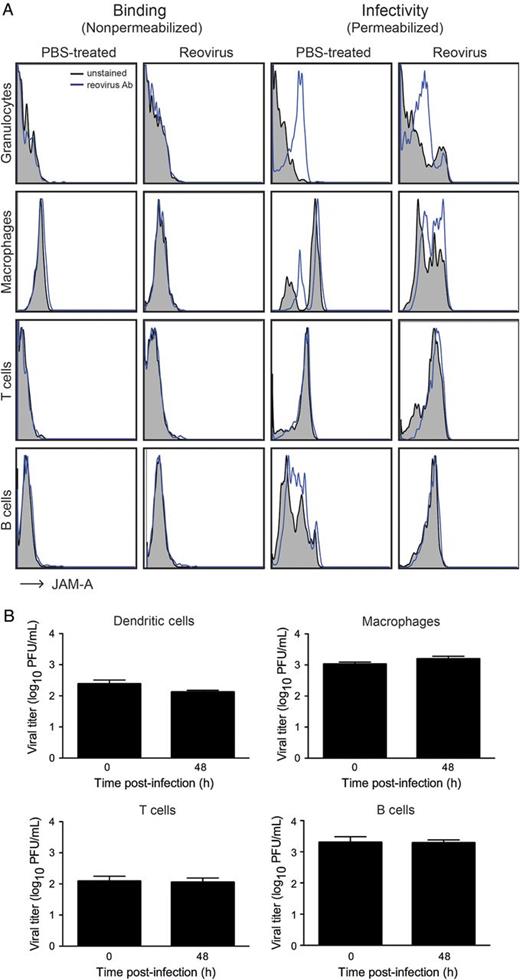
Reovirus does not infect hematopoietic cells in vivo or ex vivo. A, Newborn (2–3 days) wild-type mice were inoculated perorally with either phosphate-buffered saline or reovirus strain T1L at 5 × 106 PFU per mouse. At 8 days post-inoculation, hematopoietic cells from the blood were isolated and stained with antibodies specific for hematopoietic cell subsets and reovirus-specific polyclonal antiserum using either nonpermeabilizing (to detect binding) or permeabilizing (to detect infection) conditions. The percentage of infected cells within each cell population was determined using flow cytometry. B, Dendritic cells, macrophages, T cells, and B cells were isolated from the spleens of 5-day-old C57BL/6 mice by fluorescence-activated cell sorting using antibodies specific for CD11c, CD11b, CD3, and B220, respectively. Isolated cells were infected with reovirus strain rsT3D/T1LS1 at a multiplicity of infection of 100 PFU/cell, and viral titers were determined by plaque assay at 0 and 48 hours post-infection. Results are presented as mean viral titer at each time point. Error bars indicate standard deviation.
Next, we examined reovirus replication in distinct leukocyte populations ex vivo (Figure5B). Dendritic cells, macrophages, T cells, and B cells were isolated from the spleens of 5-day-old mice using FACS, and individual cell populations were infected with reovirus strain rsT3D/T1LS1, which disseminates hematogenously [3]. Viral titers in cell lysates were determined by plaque assay at 0 and 48 hours post-infection. Viral titers were roughly equivalent at both time points in each of these cell populations (Figure5B), indicating that reovirus does not replicate in explanted hematopoietic cells. Together, these data demonstrate that reovirus does not bind to or infect hematopoietic cells in vivo and suggest that reovirus disseminates following amplification in endothelial cells by a cell-free viremia.
DISCUSSION
Reoviruses are useful experimental models to dissect mechanisms by which viruses gain access to systemic transport pathways in the host. In this study, we assessed hematogenous reovirus spread in mice with tissue-specific alterations in JAM-A expression. We hypothesized that systemic bloodstream spread of reovirus depends on JAM-A expression in the endothelium and on hematopoietic cells. The key finding in this study is that endothelial JAM-A but not hematopoietic JAM-A is required for reovirus bloodstream transmission.
To establish mice with altered JAM-A expression in the endothelial compartment, we used animals that express Cre recombinase driven by the Tek promoter. Tek is a tyrosine kinase expressed in endothelial cells, but this protein also is expressed in hematopoietic stem cells [32]. Tek-dependent Cre recombinase expression in hematopoietic stem cells likely accounts for the absence of JAM-A expression in all hematopoietic cell types in EndoJAM-AKD mice (Figure1D and Supplementary Data). To exclude the possibility that the absence of hematopoietic JAM-A might confound the results obtained using EndoJAM-AKD mice, we established HematoJAM-AKO and HematoJAM-A mice, which respectively lack or express JAM-A exclusively in hematopoietic cells.
Compared with wild-type mice, EndoJAM-AKD mice, which display diminished JAM-A expression on endothelial cells, have significantly lower levels of viremia after peroral or intravenous inoculation of reovirus (Figures2 and 3 and Supplementary Data). This finding suggests that endothelial JAM-A expression is required for reovirus entry into and exit from the bloodstream. Subsequent spread to sites of secondary replication (eg, brain and heart) after peroral inoculation is significantly diminished in EndoJAM-AKD mice (Figure2), suggesting that efficient spread to those sites requires a threshold level of viremia. Although viral titers in organ homogenates prepared from EndoJAM-AKD mice are significantly lower than those observed in wild-type mice, there is detectable virus at these sites. This finding may be attributable to residual JAM-A expression in the endothelium of EndoJAM-AKD mice or the presence of another host component that facilitates systemic trafficking of reovirus. Nonetheless, our findings provide strong evidence that endothelial JAM-A promotes bloodstream entry during systemic reovirus infection.
We envision 3 possible mechanisms by which reovirus could traverse an endothelial cell layer to invade the underlying tissue. First, reovirus may infect endothelial cells from the luminal surface and be released abluminally. Endothelial cells are readily infected by reovirus in culture [19], and reovirus antigen can be detected in endothelial cells within the brains of mice infected perorally with reovirus (data not shown). Second, reovirus infection of endothelial cells may induce barrier dysfunction and vascular leak, allowing reovirus access to tissue surrounding blood vessels. However, we found that reovirus infection does not induce vascular leak into the brain at 4 and 8 days post-inoculation, which coincide with times at which infectious virus can be detected at that site (Figure4). Third, reovirus may use hematopoietic cells to traffic hematogenously. Surprisingly, we found that viral titers in the blood and at sites of secondary replication of HematoJAM-AKO mice, which lack all hematopoietic JAM-A, are equivalent to those seen in wild-type mice after peroral or intravenous inoculation (Figures2 and 3 and Supplementary Data). Additionally, viral titers in HematoJAM-A mice, which express JAM-A only in hematopoietic cells, mirrored those seen in JAM-AKO mice (Figures2 and 3 and Supplementary Data). These data suggest that hematopoietic JAM-A is dispensable for reovirus bloodstream spread. However, it is possible that cell type-specific differences in posttranslational modification of JAM-A allows reovirus to infect and replicate in endothelial cells and not hematopoietic cells. Since reovirus associates with human dendritic cells in vivo [33], we thought that the virus might bind to or infect leukocytes using a receptor distinct from JAM-A. However, we found that reovirus does not attach to or productively infect murine hematopoietic cells in vivo (Figure5A and Supplementary Data) or ex vivo (Figure5B), suggesting that reovirus strains differ in the capacity to infect leukocytes in vivo or that such interactions are species specific.
Reovirus replication in endothelial cells appears to promote the development of viremia and facilitates systemic spread to sites of secondary replication. Viral titers in the blood of JAM-AKO mice are significantly lower than those observed in wild-type mice after peroral or intravenous inoculation (Figures2 and 3), suggesting that JAM-A promotes establishment of viremia. Interferon-β is induced in equal amounts in the intestines of wild-type and JAM-AKO mice 24 hours post-inoculation (data not shown), suggesting that differences in viral titer in animals varying in JAM-A expression are unlikely to be attributable to differences in reovirus-induced innate immune activation in the presence and absence of JAM-A. Infection of polarized endothelial cells with reovirus requires JAM-A [19] and may serve as a means to amplify reovirus within the bloodstream. Lower viral titers in the blood of EndoJAM-AKD mice in comparison to those in HematoJAM-AKO mice after peroral or intravenous inoculation (Figures2 and 3) suggest that endothelial JAM-A and not hematopoietic JAM-A is required for both development of viremia and viral egress from the bloodstream.
Reoviruses are superb candidates for oncolytic therapeutics due to their preferential replication in transformed cells and capacity to induce apoptosis [1]. Spread of reovirus by the bloodstream enables the virus to target even the smallest foci of tumor cells [33]. By understanding how reovirus interacts with JAM-A on the endothelium, it may be possible to design new reovirus vectors that reach target tumors more efficiently. Oncolytic serotype 3 reoviruses, which disseminate both neurally and hematogenously in newborn mice [26], likely require expression of JAM-A on endothelial cells to spread through the bloodstream in a manner similar to that observed in our study for serotype 1 reoviruses. Thus, understanding mechanisms that govern the spread of reovirus by the bloodstream sheds light on how pathogens disseminate systemically and may enhance vector targeting for therapeutic applications.
Notes
Acknowledgments. We thank members of the Dermody Laboratory for many useful discussions and Jennifer Konopka-Anstadt for critical review of the manuscript. We thank Ambra Pozzi for assistance in the isolation of primary murine endothelial cells. We acknowledge the Transgenic Mouse/Gene Targeting Core Facility at Emory University School of Medicine for producing floxed JAM-A mice from embryonic stem cells and the Transgenic Mouse/ES Cell Shared Resource at Vanderbilt University School of Medicine for assistance in generation of various transgenic mouse strains used in this study.
Financial support. This research was supported by United States Public Health Service awards T32 GM007347 (C. M. L.), F31 NS074596 (C. M. L.), T32 CA09385 (K. W. B.), F32 AI075776 (K. W. B.), R01 DK61379 (C. A. P), R01 DK72564 (C. A. P.), and R37 AI38296 (T. S. D.), all from the National Institutes of Health, and the Elizabeth B. Lamb Center for Pediatric Research. Additional support was provided by the Vanderbilt Diabetes Research and Training Center (DK020593), the Vanderbilt Flow Cytometry Shared Resource (DK058404), the Vanderbilt Cell Imaging Shared Resource (DK20593), and the Vanderbilt-Ingram Cancer Center (CA68485).
Authorship contribution. C. M. L., K. W. B., and A. J. P. designed and performed the experiments, analyzed the results, and wrote the manuscript. V. V. P. analyzed the results. C. A. P. and L. V. K. provided critical reagents and experimental advice. T. S. D. designed the experiments, analyzed the results, and wrote the manuscript.
Potential conflicts of interest. All authors: No potential conflicts of interest.
All authors have submitted the ICMJE Form for Disclosure of Potential Conflicts of Interest. Conflicts that the editors consider relevant to the content of the manuscript have been disclosed.
References
Author notes
Present address: Department of Microbiology and Immunology, University of Arkansas for Medical Sciences, Little Rock.