-
PDF
- Split View
-
Views
-
Cite
Cite
John M. Atack, Ines Ibranovic, Cheryl-Lynn Y. Ong, Karrera Y. Djoko, Nathan H. Chen, Rachel vanden Hoven, Michael P. Jennings, Jennifer L. Edwards, Alastair G. McEwan, A Role for Lactate Dehydrogenases in the Survival of Neisseria gonorrhoeae in Human Polymorphonuclear Leukocytes and Cervical Epithelial Cells, The Journal of Infectious Diseases, Volume 210, Issue 8, 15 October 2014, Pages 1311–1318, https://doi.org/10.1093/infdis/jiu230
- Share Icon Share
Abstract
Lactate is an abundant metabolite, produced by host tissues and commensal organisms, and it represents an important potential carbon source for bacterial pathogens. In the case of Neisseria spp., the importance of the lactate permease in colonization of the host has been demonstrated, but there have been few studies of lactate metabolism in pathogenic Neisseria in the postgenomic era. We describe herein the characterization of genome-annotated, respiratory, and substrate-level lactate dehydrogenases (LDHs) from the obligate human pathogen Neisseria gonorrhoeae. Biochemical assays using N. gonorrhoeae 1291 wild type and isogenic mutant strains showed that cytoplasmic LdhA (NAD+-dependent D-lactate dehydrogenase) and the membrane-bound respiratory enzymes, LdhD (D-lactate dehydrogenase) and LldD (L-lactate dehydrogenase) are correctly annotated. Mutants lacking LdhA and LdhD showed greatly reduced survival in neutrophils compared with wild type cells, highlighting the importance of D-lactate metabolism in gonococcal survival. Furthermore, an assay of host colonization using the well-established human primary cervical epithelial cell model revealed that the two respiratory enzymes make a significant contribution to colonization of and survival within the microaerobic environment of the host. Taken together, these data suggest that host-derived lactate is critical for the growth and survival of N. gonorrhoeae in human cells.
Lactate represents a potential carbon and energy source for bacterial pathogens. For example, in human serum, lactate concentrations are approximately 1 mmol/L, versus approximately 4 mmol/L for glucose [1]. Lactate is also abundant in the female genital tract where the metabolic activity of lactic acid bacteria produces over 6 mmol/L lactate [1]. In Neisseria gonorrhoeae, loss of the lactate permease (LctP) decreases colonization of the mouse genital tract and results in reduced density and duration of carriage [2]. Although caution should be exerted in applying observations made in mice to humans, these data strongly suggest that L-lactate metabolism is important for gonococcal growth and survival.
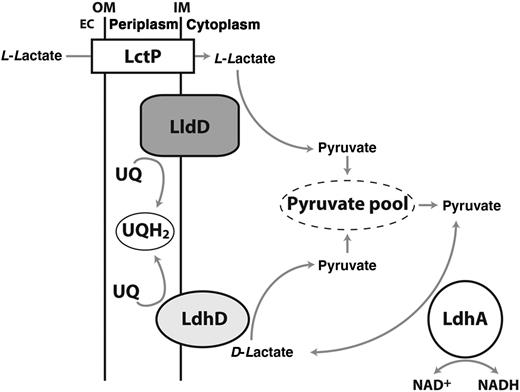
Proposed model for organization and roles of lactate dehydrogenases (LDHs) in Neisseria gonorrhoeae. Abbreviations: EC, extracellular space; IM, inner membrane; LctP, L-lactate permease; LdhA, NAD+-linked D-LDH; LdhD, respiratory D-LDH; LldD, respiratory L-LDH; NAD+, nicotinamide adenine dinucleotide, oxidized; NADH, nicotinamide adenine dinucleotide, reduced; OM, outer membrane; UQ, ubiquinone; UQH2, ubiquinol.
In the current study, we sought to confirm the properties of these annotated LDHs and define the role of these enzymes in host colonization and gonococcal survival in human polymorphonuclear leukocyte (PMN) and primary cervical epithelial (pex) cells.
MATERIALS AND METHODS
Bacterial Strains and Growth
N. gonorrhoeae strains were routinely grown (37°C, 5% carbon dioxide) on GC agar (Oxoid) supplemented with 1% (vol/vol) IsoVitalX (Becton Dickinson). Liquid cultures were grown in GW broth [7] with 36 mmol/L glucose (GW-g broth) or in chemically defined medium (CDM) [8] containing glucose (50 mmol/L). During growth curves using CDM, glucose, L-lactate, or D-lactate was provided at a final concentration of 0.5% (wt/vol). N. gonorrhoeae cells were grown aerobically by shaking at 200 rpm in flasks ≥5 times larger than culture volume. Microaerobic cultures used a volume-vessel size ratio of 0.9:1 with minimal shaking (25 rpm). All gonococci used in these studies were piliated and expressed Opa. E. coli was grown at 37°C on Luria-Bertani medium supplemented with 100 µg/mL ampicillin or kanamycin, when necessary.
DNA Manipulations and Genetic Techniques
Plasmid DNA was isolated using the QIAprep Spin Plasmid Miniprep kit; polymerase chain reaction (PCR) analysis was performed using KOD Hot Start Polymerase (Novagen/EMD Millipore) or Taq Polymerase (New England Biolabs). Restriction enzymes were from New England Biolabs. All of the above were used according to the manufacturer's specifications. DNA sequencing was performed by the Australian Equine Genome Research Facility.
A N. gonorrhoeae ldhA mutant was generated by amplifying the gene (NGO1336) plus flanking regions from N. gonorrhoeae 1291 wild type genomic DNA using primers LDHA-F and LDHA-R (Table 1). This product was ligated into the cloning site of pGEM-T-easy (Promega). An internal fragment of ldhA was removed by digestion with BsaBI and BmgBI, and the HincII-digested, pUC4kan-derived (Pharmacia) kanamycin resistance (KanR) cassette was inserted. This KanR cassette does not introduce polar effects on downstream gene expression in Neisseria [9]. Using this same approach a ldhD (NGO0890) mutant was made using primers DLD-F and DLD-R. In this case, an internal BsaBI- and EcoRV-derived fragment was replaced by the same KanR cassette described above. Similarly, an lldD mutant was generated using primers LLD-F and LLD-R to isolate and clone the lldD gene (NGO0639), with the KanR cassette replacing an internal EcoRV and BmgBI fragment. All plasmids were transformed into N. gonorrhoeae 1291 wild type by natural transformation, with the resulting kanamycin-resistant mutants designated as ΔldhA, ΔldhD, and ΔlldD respectively. Each mutant strain was confirmed by PCR using forward and reverse gene- and KanR cassette–specific primers (Table 1).
Primer . | Sequence 5′-3′ . |
---|---|
LDHA-F | CTC ACC GGT ATG CTT GCA GC |
LDHA-R | CCG GGA AAC ACG CCT ACC |
DLD-F | CAG CGA ACG CAT CCG CAC |
DLD-R | GAA CTG CCC TAC CGC GTG |
LLD-F | AAA ACC ATG GGG AAG GCT GAT CAG G |
LLD-R | AAA CTG CAG GGC AGT CAT GGT ATG G |
Kan-out-F | CAT TTG ATG CTC GAT GAG TTT TCT AA |
Kan-out-R | AGA CGT TTC CCG TTG AAT ATG GCT CAT |
ldhA comp-F | CCG CAC CTA CAA CTA TCC CC |
ldhA comp-R | GAA GAT TTC CAA GCA CGC CG |
ldhD comp-F | CGG ACA ATA TCA ACG TCG GC |
ldhD comp-R | GGC GGG ATG GGT GTA GAT TT |
lldD comp-F | CAG TCA AGT ATG GGG GAG CTG |
lldD comp-R | CGT TTG ATC GGT TTG CCG TT |
proB-F | GAC AAA CGC CGC TAC CAA AA |
proB-R | GTT GTC GCC GAT TTT CAA TT |
Primer . | Sequence 5′-3′ . |
---|---|
LDHA-F | CTC ACC GGT ATG CTT GCA GC |
LDHA-R | CCG GGA AAC ACG CCT ACC |
DLD-F | CAG CGA ACG CAT CCG CAC |
DLD-R | GAA CTG CCC TAC CGC GTG |
LLD-F | AAA ACC ATG GGG AAG GCT GAT CAG G |
LLD-R | AAA CTG CAG GGC AGT CAT GGT ATG G |
Kan-out-F | CAT TTG ATG CTC GAT GAG TTT TCT AA |
Kan-out-R | AGA CGT TTC CCG TTG AAT ATG GCT CAT |
ldhA comp-F | CCG CAC CTA CAA CTA TCC CC |
ldhA comp-R | GAA GAT TTC CAA GCA CGC CG |
ldhD comp-F | CGG ACA ATA TCA ACG TCG GC |
ldhD comp-R | GGC GGG ATG GGT GTA GAT TT |
lldD comp-F | CAG TCA AGT ATG GGG GAG CTG |
lldD comp-R | CGT TTG ATC GGT TTG CCG TT |
proB-F | GAC AAA CGC CGC TAC CAA AA |
proB-R | GTT GTC GCC GAT TTT CAA TT |
Primer . | Sequence 5′-3′ . |
---|---|
LDHA-F | CTC ACC GGT ATG CTT GCA GC |
LDHA-R | CCG GGA AAC ACG CCT ACC |
DLD-F | CAG CGA ACG CAT CCG CAC |
DLD-R | GAA CTG CCC TAC CGC GTG |
LLD-F | AAA ACC ATG GGG AAG GCT GAT CAG G |
LLD-R | AAA CTG CAG GGC AGT CAT GGT ATG G |
Kan-out-F | CAT TTG ATG CTC GAT GAG TTT TCT AA |
Kan-out-R | AGA CGT TTC CCG TTG AAT ATG GCT CAT |
ldhA comp-F | CCG CAC CTA CAA CTA TCC CC |
ldhA comp-R | GAA GAT TTC CAA GCA CGC CG |
ldhD comp-F | CGG ACA ATA TCA ACG TCG GC |
ldhD comp-R | GGC GGG ATG GGT GTA GAT TT |
lldD comp-F | CAG TCA AGT ATG GGG GAG CTG |
lldD comp-R | CGT TTG ATC GGT TTG CCG TT |
proB-F | GAC AAA CGC CGC TAC CAA AA |
proB-R | GTT GTC GCC GAT TTT CAA TT |
Primer . | Sequence 5′-3′ . |
---|---|
LDHA-F | CTC ACC GGT ATG CTT GCA GC |
LDHA-R | CCG GGA AAC ACG CCT ACC |
DLD-F | CAG CGA ACG CAT CCG CAC |
DLD-R | GAA CTG CCC TAC CGC GTG |
LLD-F | AAA ACC ATG GGG AAG GCT GAT CAG G |
LLD-R | AAA CTG CAG GGC AGT CAT GGT ATG G |
Kan-out-F | CAT TTG ATG CTC GAT GAG TTT TCT AA |
Kan-out-R | AGA CGT TTC CCG TTG AAT ATG GCT CAT |
ldhA comp-F | CCG CAC CTA CAA CTA TCC CC |
ldhA comp-R | GAA GAT TTC CAA GCA CGC CG |
ldhD comp-F | CGG ACA ATA TCA ACG TCG GC |
ldhD comp-R | GGC GGG ATG GGT GTA GAT TT |
lldD comp-F | CAG TCA AGT ATG GGG GAG CTG |
lldD comp-R | CGT TTG ATC GGT TTG CCG TT |
proB-F | GAC AAA CGC CGC TAC CAA AA |
proB-R | GTT GTC GCC GAT TTT CAA TT |
Mutant gonococci were complemented as described elsewhere [10]. Briefly, ldhA, ldhD, and lldD, with their native promoters, were amplified using the primer pairs ldhA comp-F & -R, ldhD comp-F & -R, and lldD comp-F & -R (Table 1), and the product was inserted into the SmaI site of pCTS32 [10]. The resulting plasmids pCTS32::ldhA, pCTS32::ldhD, and pCTS32::lldD were transformed into their respective recipient mutant strain. Spectinomycin-resistant transformants were checked with PCR using the primer pair proB-F & -R (Table 1), and verified by means of DNA sequencing.
N. gonorrhoeae Membrane Vesicle Preparation
N. gonorrhoeae were grown in CDM [8] containing 50 mmol/L glucose with vigorous shaking (200 rpm). After 5 hours (optical density at 600 nm [OD600], approximately 1.0), cells were harvested by means of centrifugation, washed twice with ice-cold 1× phosphate-buffered saline (PBS; 10 mmol/L magnesium chloride; pH 7.4), resuspended in ice-cold 1× PBS containing 25% (vol/vol) glycerol, and frozen at −80°C until further use. To obtain cell-free membrane vesicles, frozen cell suspensions were centrifuged (5000 g, 5 minutes) and resuspended in ice-cold Na-HEPES buffer (50 mmol/L; 10 mmol/L magnesium chloride; pH 7.4). Cells were disrupted by 3 passages through a French press (15 000 psi). Cell debris was removed by means of centrifugation (5000 g, 20 minutes). The supernatant was filtered through a 0.22-mm filter (MilliPore) and centrifuged (150 000 g, 2 hours, 4°C). The membrane-rich pellet was homogenized in 500 µL of ice-cold Na-HEPES buffer and stored on ice until further analyses (all performed on the same day). Protein concentrations were assayed using QuantiPro BCA Assay Kit (Sigma).
Oxygen Consumption Assays
Respiratory studies were based on the method of Markwell and Lascelles [11] using membrane vesicles isolated from N. gonorrhoeae 1291 wild type and ldhD and lldD mutants and complemented strains, using an Oxygraph oxygen electrode with its accompanying software (Hansatech). Reactions were performed at 35°C in a final volume of 2 mL Na-HEPES buffer. Membrane vesicles (50 µL;10 mg of total protein) were added to the reaction chamber at 10 seconds. Background respiration was recorded for 2 minutes. Reduction in oxygen was initiated by the addition of 50 mmol/L (final concentration) sodium L- or D-lactate. Reactions were monitored for another 2 minutes. The rates of oxygen consumption (in nanomoles of oxygen per minute) were plotted using the Oxygraph Plus program, and results were normalized to total protein content (in milligrams).
Enzyme Assays
Cell free extracts (CFEs) were prepared from 100 mL mid–log phase (OD600, approximately 0.5) GW-g broth cultures of N. gonorrhoeae. Cells were harvested by means of centrifugation (5000 g, 30 minutes, 4°C), and the cell pellet was lysed with BugBuster Protein Extraction Reagent (Novagen). Debris was removed by centrifugation (14 000 g, 30 minutes, 4°C) and the supernatant (ie, the CFE) was collected for use in enzyme assays. NAD+-dependent LDH activity was determined at 37°C in a reaction mixture containing 10 mmol/L Tris-hydrochloride (pH 7.5), 30 mmol/L sodium pyruvate or D-lactate, 0.4 mmol/L nicotinamide adenine dinucleotide, reduced (NADH) or NAD+, and an appropriate volume of CFE and distilled water to a 1-mL final volume. The reaction was started by adding sodium pyruvate or D-lactate, and the oxidation/reduction of NADH/NAD+ was followed at 340 nm. Specific activities were calculated using an extinction coefficient for NADH/NAD+ of 6.2 mmol/L−1 cm−1 at 340 nm.
Assays for Excreted Metabolites
Two-milliliter samples of gonococcal cultures (grown aerobically or microaerobically in GW-g broth) were collected by centrifugation after 4, 6, 8, and 24 hours of growth. These samples were pelleted by centrifugation (5000 g, 5 minutes, 22°C). The resulting supernatant was filter sterilized and used to assay metabolite concentrations using the D-lactate and acetate determination kits according to the manufacturer's instructions (Megazyme).
PMN Killing Assays
Killing assays were performed using human PMNs as described elsewhere; however, bacteria were not opsonized before PMN infection [12]. Bacterial suspensions were made from overnight plates in 1× PBS, and centrifuged (500 rpm, 5 minutes) to remove clumping cells. PMN viability was assessed by means of microscopy. Data are expressed as an index of survival ([survival at Tx/PMN association at T0] × 100). Human blood donation was in accordance with the National Statement on Ethical Conduct in Human Research, complying with the regulations governing experimentation on humans, and approved by the University of Queensland Medical Research Ethics Committee.
N. gonorrhoeae Association, Invasion, and Survival Assays
Pex cells were procured from surgical cervical tissue and maintained as described elsewhere [13]. Cervical tissue was obtained from premenopausal women undergoing hysterectomy at Ohio State University Medical Center for medically indicated reasons not related to our study and was provided by the Cooperative Human Tissue Network (The Research Institute at Nationwide Children's Hospital, Columbus, Ohio). In accordance with National Institutes of Health guidelines (article HHS45CFR46, exemption 4) and the Institutional Review Board, these tissues do not constitute human subjects. Quantitative association, invasion, and survival assays were performed as described elsewhere [14]. Microaerobic conditions (6% oxygen, 5%–8% carbon dioxide) were generated using Mitsubishi Pouch-MicroAero gas-generating pouches. Microaerobically cultured gonococci were used to challenge microaerobically incubated pex cells. After infection, pex cells were returned to a microaerobic environment. Separate gas pouches were used for each assay time point, and exposure to air was minimized throughout the assay; air was vacuum-evacuated from pouches before the 30 minutes incubation with gentamicin. For all assays, serial dilutions of the cell lysates were plated to enumerate viable colony-forming units. The percentage of association, invasion, or survival was determined as described elsewhere [14].
RESULTS
Mutational Analysis of LDHs in N. gonorrhoeae: Confirmation of Biochemical Properties
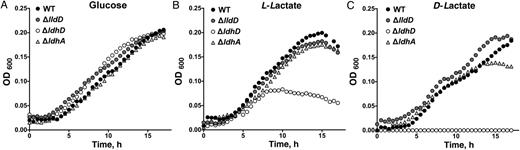
Growth curve analysis of Neisseria gonorrhoeae 1291 wild type (WT) and ΔlldD, ΔldhD, and ΔldhA mutants in chemically defined medium containing glucose (A), L-lactate (B), or D-lactate (C) . Carbon sources were added at a final concentration of 0.5% (wt/vol). Each time point is representative of 6 replicates. Abbreviation: OD600, optical density at 600 nm.
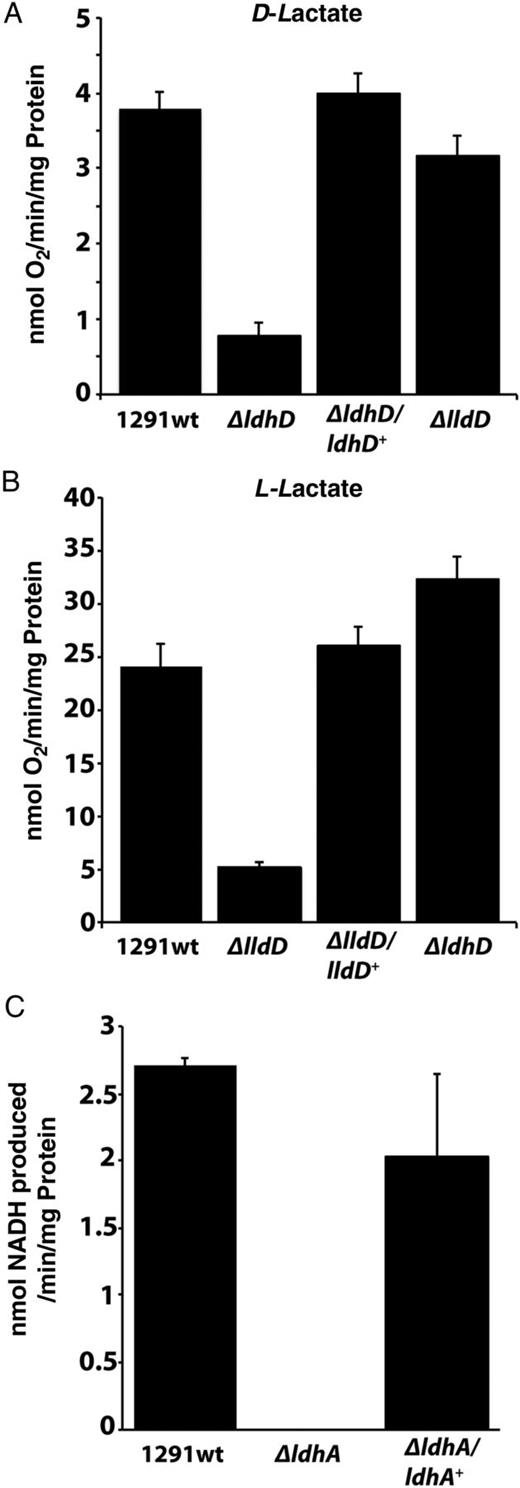
LDH assays using N. gonorrhoeae membranes and cell-free extracts. A, B, Oxygen consumption assays performed using D-lactate (A) and L-lactate (B) with membrane vesicles from 1291 wild type N. gonorrhoeae, ΔldhD, ΔlldD, and complemented mutant strains ΔldhD/ldhD+ and ΔlldD/lldD+. C, Coupled assays with D-lactate plus nicotinamide adenine dinucleotide, oxidized (NADH), using wild type N. gonorrhoeae, ΔldhA, and ΔldhA/ldhA+ cell-free extracts. Each assay was performed in triplicate, with error bars representing standard deviations. Abbreviation: NADH, nicotinamide adenine dinucleotide, reduced.
Soluble D-Lactate–Specific LDH in CFEs of N. gonorrhoeae
Previous studies have shown that E. coli LdhA is stereospecific for D-lactate [6]. The use of D-lactate as a substrate with CFE prepared from 1291 wild type bacteria resulted in an increase in absorbance (indicative of electron transfer) at 340 nm (Figure 3C). This activity was lost in the ΔldhA mutant, and was restored in the complemented strain ΔldhA/ldhA+ (Figure 3C). No such increase was observed when L-lactate was used as an electron donor with 1291 wild type CFE (Supplementary Data), indicating that N. gonorrhoeae LdhA is D-lactate specific. The directionality of LdhA oxidoreductase activity was then compared using 2 redox couples: (1) pyruvate and NADH and (2) D-lactate and NAD+. The D-lactate–NAD+ oxidoreductase activity in 1291 wild type CFE was increased approximately 3.5-fold versus the reverse NADH-pyruvate oxidoreductase activity (Supplementary Data). Thus, under conditions of saturating concentrations of both substrate and electron acceptor/donor, LdhA has a higher activity as a D-lactate–NAD+ oxidoreductase. However, in vivo, NADH-pyruvate oxidoreductase activity could play a more significant role, for example, in D-lactate production for use by LdhD or to increase flow through the pyruvate pool (Figure 1).
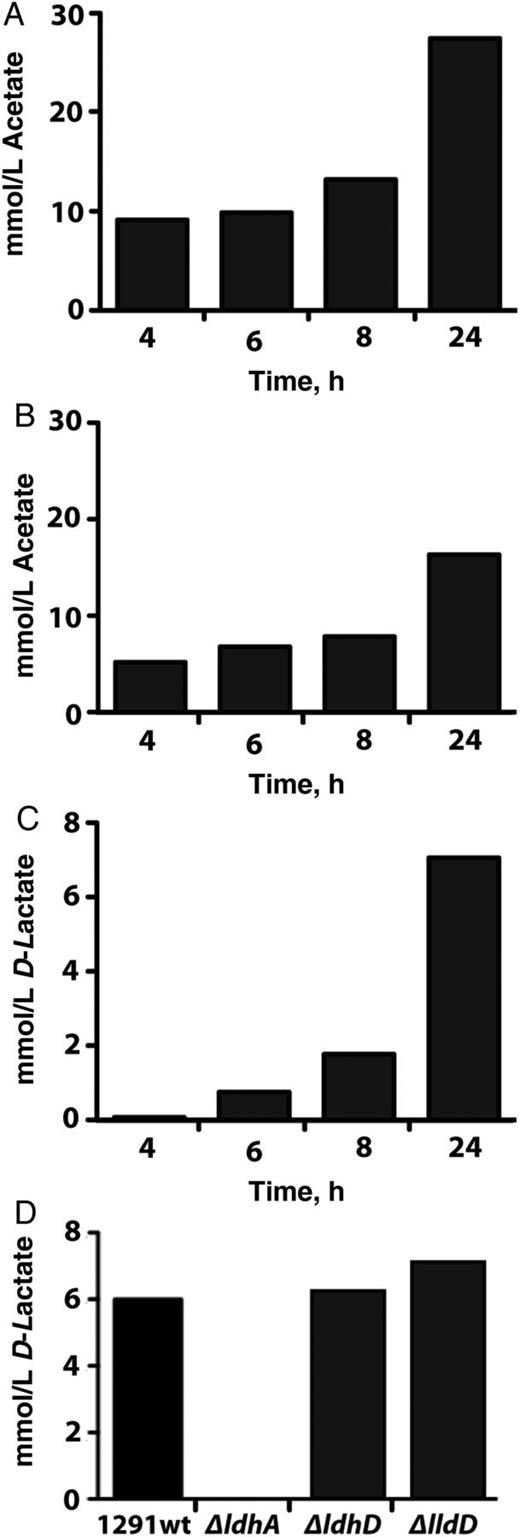
Determination of excreted metabolites when glucose is supplied as the sole carbon source. A, B, Acetate accumulation monitored over 24 hours using wild type Neisseria gonorrhoeae grown under aerobic (A) or microaerobic (B) conditions. C, D-lactate accumulation monitored over 24 hours using wild type N. gonorrhoeae grown under microaerobic conditions. (D) D-lactate concentration in growth media after 24 hours using wild type N. gonorrhoeae and the 3 isogenic mutant strains grown under microaerobic conditions. Cells were grown as detailed in Materials and Methods. Each experiment was performed in duplicate.
The Role of LDHs in Survival of Gonococci in Human Neutrophils
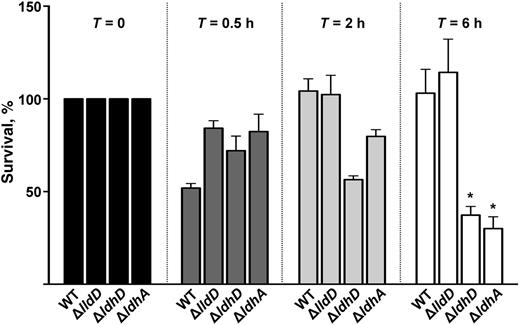
The percentage of survival of Neisseria gonorrhoeae wild type and isogenic mutants ΔlldD, ΔldhD, and ΔldhA after incubation with human polymorphonuclear leukocytes. These data demonstrate the intracellular survival and growth of bacteria at time points (T) 0, 0.5, 2, and 6 hours after infection. Intracellular counts were normalized to T = 0 (100%); subsequent time points were expressed as the percentage survival of T = X compared with T = 0. At T = 6 hours, only ΔldhD and ΔldhA had reduced intracellular survival compared with wild type (WT). *P < .01 (Student's t test).
Respiratory LDHs Required for Growth in Pex Cells Under Physiological Conditions
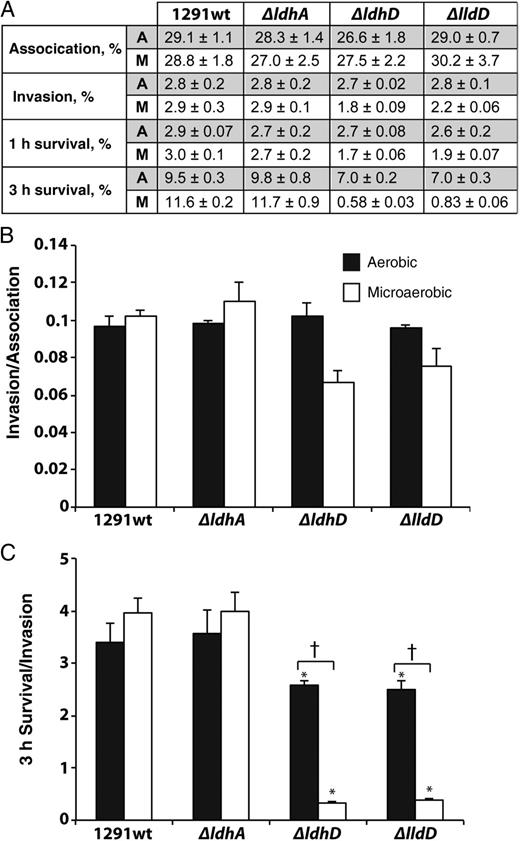
Wild type and mutant Neisseria gonorrhoeae cervical cell infection studies under aerobic and microaerobic conditions. A, Association (90 minutes), invasion (90 minutes of infection plus 30 minutes of gentamicin treatment), 1-hour survival (viable bacteria 1 hour after invasion), and 3-hour survival (viable bacteria 3 hours after invasion), calculated as a percentage of the initial inoculum. Each experiment was performed in triplicate on 3 occasions. All corresponding P values, determined with Kruskal–Wallis analysis of variance, are presented in Supplementary Data. B, Primary cervical epithelial cell invasion indices expressed as invasion/association. C, Three-hour survival indices expressed as 3-hour survival/invasion. *P < .0001 (vs wild type for growth condition; Student t test); †P <.0001 (aerobic vs microaerobic for strain; Student t test). Abbreviations: A, aerobic; M, microaerobic.
DISCUSSION
There is a significant body of evidence indicating that lactate metabolism has an important role in neisserial virulence [1, 2, 5, 16–20], but before this work, our understanding of the organization of LDHs in these organisms was lacking. In our study, we have shown that N. gonorrhoeae has 2 respiratory LDHs, one specific for L-lactate (LldD) and the other specific for D-lactate (LdhD). A third, cytoplasmic, NAD+-dependent LDH (LdhA) generates D-lactate. The respiratory LDHs are membrane-bound flavoproteins that catalyze the reduction of ubiquinone. Although this electron transfer process is not directly linked to the generation of a proton electrochemical gradient, the remaining components of such a respiratory chain under conditions of abundant oxygen (cytochrome bc1 and cytochrome cbb3 oxidase) imply that 2 sites of energy conservation would exist.
In pex cells grown under aerobic conditions, we did not observe any difference in the immediate (ie, association, invasion, and 1-hour survival) survival of the LDH mutants when compared with wild type gonococci. However, the situation seems quite different in a microaerobic (physiological) environment. During microaerobiosis, mammalian cells mainly produce L-lactate, with small amounts of D-lactate also being produced, possibly resulting from the methylglyoxal pathway [21]. Thus, both D- and L-lactate would be available to gonococci as electron donors at the cervical epithelia, with L-lactate in abundance on intracellular invasion. Our observation that both ldhD and lldD are required for the extended survival of N. gonorrhoeae within pex cells under microaerobic conditions (as would occur in vivo) is consistent with this view.
Strain ΔldhD (lacking LdhD), but not strain ΔlldD (lacking LldD), exhibited a survival defect in PMN cells, suggesting that the ability to remove D-lactate is critical for N. gonorrhoeae growth. Consistent with this view, the ΔldhD mutant showed attenuated growth with L-lactate as a sole carbon source (Figure 2B). The ΔldhA mutant also showed reduced survival in PMNs and reduced growth in vitro in the presence of D-lactate (Figure 2C) but was not affected under the same conditions with L-lactate as a carbon source. This suggests that removal of D-lactate is critical for growth of the gonococcus when L-lactate is provided as a carbon source. The lack of impact of a ΔlldD mutation under these conditions can be rationalized if the L-lactate acquired by N. gonorrhoeae is oxidized to pyruvate via LdhD, which is known to have LldD activity [5]. This also explains the residual activity seen in vitro with strain ΔlldD when L-lactate is provided as a respiratory substrate (Figure 3B). Our results are consistent with the known ability of PMNs to produce considerable amounts of L-lactate [16, 22]. The situation in pex cells is probably different, and, if the concentration of L-lactate is low, the very high apparent Km of LdhD for L-lactate [5] may not be sufficient to support growth.
N. gonorrhoeae can survive at low oxygen levels because it has cytochrome cbb3 [23], an oxidase capable of operating at very low oxygen concentrations [24]. Thus, within the in vivo human mucosal environment, where free glucose, oxygen, and iron are limited, lactate generation/regeneration may be key for respiration for N. gonorrhoeae during infection and colonization. Lactate use also seems important in several other bacterial pathogens: in Haemophilus influenzae it is linked to increased human serum-resistance [25], with lactate uptake necessary for H. influenzae bacteremia [26]; lactate is an important carbon source for the human gastric pathogen, Campylobacter jejuni [27]; and lactate usage by Mycobacterium tuberculosis is likely to play a role in granuloma development [28].
In conclusion, we have expanded on previous work with N. gonorrhoeae suggesting that lactate metabolism is important in the interaction of this bacterium with the human host. In this regard, we have now provided evidence that use of respiratory lactate is critical to the intracellular survival of N. gonorrhoeae after its association with human cells and, in this way, may promote human colonization. The apparent conservation of a similar suite of LDHs in a number of bacteria, and the importance of lactate usage in several human pathogens, suggests that this type of metabolic interaction between host and pathogen may be widespread.
Notes
Financial support. This work was supported by the National Institute of Allergy and Infectious Diseases (grant 1R01A1076398 to J. L. E.) and the National Health and Medical Research Council (Program grant 565526 to M. P. J. and A. G. M.).
Potential conflicts of interest. All authors: No reported conflicts.
All authors have submitted the ICMJE Form for Disclosure of Potential Conflicts of Interest. Conflicts that the editors consider relevant to the content of the manuscript have been disclosed.
References
Author notes
J. M. A. and I. I. contributed equally to this work.