-
PDF
- Split View
-
Views
-
Cite
Cite
Katrin Schilcher, Federica Andreoni, Satoshi Uchiyama, Taiji Ogawa, Reto A. Schuepbach, Annelies S. Zinkernagel, Increased Neutrophil Extracellular Trap–Mediated Staphylococcus aureus Clearance Through Inhibition of Nuclease Activity by Clindamycin and Immunoglobulin, The Journal of Infectious Diseases, Volume 210, Issue 3, 1 August 2014, Pages 473–482, https://doi.org/10.1093/infdis/jiu091
- Share Icon Share
Abstract
The Gram-positive human pathogen Staphylococcus aureus causes a variety of human diseases such as skin infections, pneumonia, and endocarditis. The micrococcal nuclease Nuc1 is one of the major S. aureus virulence factors and allows the bacterium to avoid neutrophil extracellular trap (NET)–mediated killing. We found that addition of the protein synthesis inhibitor clindamycin to S. aureus LAC cultures decreased nuc1 transcription and subsequently blunted nuclease activity in a molecular beacon–based fluorescence assay. We also observed reduced NET degradation through Nuc1 inhibition translating into increased NET-mediated clearance. Similarly, pooled human immunoglobulin specifically inhibited nuclease activity in a concentration-dependent manner. Inhibition of nuclease activity by clindamycin and immunoglobulin enhanced S. aureus clearance and should be considered in the treatment of S. aureus infections.
The Gram-positive bacterium Staphylococcus aureus is a major human pathogen causing a wide spectrum of diseases, including superficial skin infections as well as invasive infections, such as bacteremia, endocarditis, necrotizing fasciitis, and pneumonia [1–3]. Increasing antibiotic resistance of methicillin-resistant S. aureus (MRSA), as found in the well-characterized S. aureus USA 300 LAC strain, renders MRSA strains difficult to treat and may result in high mortality rates [2, 4]. Vancomycin is used for empiric treatment [5] but displays various problems, such as slow bactericidal activity, poor tissue penetration, and no activity against bacterial virulence factors [6–8]. Moreover, vancomycin-resistant S. aureus strains have developed [9–10]. Thus, additional treatment strategies are necessary to improve clinical response and to reduce further antibiotic resistance development. Ideally, these additive therapeutic strategies should target S. aureus virulence directly thereby disarming the bacteria and rendering them more susceptible to host innate immune defenses.
Efficient colonization and avoidance of host defense after invasion are facilitated by numerous staphylococcal virulence factors, including proteases, lipases, hyaluronidase, collagenase, as well as deoxyribonuclease (nuclease) [11–13]. Two active thermostable nucleases (Nuc1 and Nuc2), encoded by different open reading frames, are present in the S. aureus genome [14]. The nuc1 transcript levels are at their maximum during the postexponential growth phase, whereas those of nuc2 are high during the early exponential phase and decline afterward [15]. Nuclease activity measurements showed a strong correlation with the nuc1 transcription levels suggesting Nuc1 to be the major S. aureus nuclease [15]. Furthermore, a recent study showed that Nuc1 transcription and production is regulated in vitro and in vivo by the 2-component system SaeRS [16]. Production of Nuc1 is conserved across methicillin-susceptible S. aureus and MRSA strains and is therefore used as a unique marker to distinguish S. aureus from other staphylococcal species [17–18]. It has been shown that the expression and secretion of nucleases by various bacterial species allows bacteria to evade killing by the innate immune system through degradation of neutrophil extracellular traps (NETs) [19–21]. NETs consist of extracellular DNA fibers, histones, antimicrobial peptides and cell-specific proteases designed to entrap and kill various microbes [22]. In addition to NETs evasion streptococcal nucleases were shown to allow bacteria to circumvent Toll-like receptor 9–mediated recognition via degradation of unmethylated CpG-rich bacterial DNA [23].
Various nuclease activity detection methods have been developed, such as gel electrophoresis, enzyme-linked immunosorbent assays, or filter binding. However, they all have some disadvantages, such as being time consuming or discontinuous or requiring handling of radioactive material. To overcome such obstacles, fluorescence-based assays have been developed using DNA or RNA target molecules labeled with a fluorophore and a quencher. In the uncleaved state, the quencher is in spatial proximity to the fluorophore. On cleavage of the target molecule, the quencher is no longer in the vicinity of the fluorophore, and an increase in fluorescence can be measured [24–29].
Owing to its excellent bioavailability, the protein synthesis inhibitor clindamycin is commonly used in clinics, in particular for skin and soft-tissue infections. However, owing to its bacteriostatic action, clindamycin is not the treatment of choice for S. aureus bacteremia. Clindamycin at low concentrations was shown to inhibit toxic-shock syndrome exotoxin production [30]. Transcription of most S. aureus exoproteins is consistently inhibited. Levels of messenger RNA (mRNA) are affected by protein inhibitors, most likely via inhibition of global regulators [31]. For example, α-hemolysin expression is impaired by clindamycin [32], paralleled by decreased mRNA expression [33]. In addition to antibiotics, immunoglobulin may be used in severe staphylococcal infections as an adjunct treatment. Immunoglobulin contains antibodies capable of neutralizing and/or binding exoproteins of S. aureus [34–35].
We hypothesized that clindamycin as well as immunoglobulin could be used to treat S. aureus infections, aiming to attenuate staphylococcal virulence factors and thus rendering the bacteria more susceptible to host clearance. We present a simple-to-perform assay to quantify nuclease activity and show that both clindamycin and immunoglobulin efficiently blocked staphylococcal Nuc1. This translated into more efficient NET-mediated clearance of S. aureus. Thus, specific inhibition of S. aureus nuclease activity should be considered a complementary treatment strategy for severe staphylococcal infections.
MATERIAL AND METHODS
Bacterial Strains and Growth Conditions
The following bacterial strains were used: S. aureus LAC wild-type (wt) (CA-MRSA; pulsed-field type USA300), nuclease-deficient derivative LAC Δnuc1 [19, 27], and 3 clinical MRSA isolates (MRSA 1–3) exhibiting clindamycin resistance with 2 distinct pulse field types. All bacterial strains were grown in Lennox broth (LB) liquid medium (BD Difco) or on blood agar plates (Trypcase soy agar plus 5% sheep blood; bioMérieux) and incubated with shaking (220 rpm) at 37°C, unless otherwise indicated. For antibiotic assays, bacterial cultures were diluted to an optical density at 600 nm (OD600) of 0.1 in fresh LB and then grown to midlogarithmic phase (OD600, 0.4–0.5). The cultures were diluted to 3–5 ×105 colony-forming units/mL, clindamycin hydrochloride (0.03 or 0.6 µg/mL; AppliChem) or the dilution medium (control) was added, and the bacteria were grown to postexponential phase (OD600, 2.5 ± 0.1). Bacterial pellets were then frozen in liquid nitrogen, and bacterial supernatants were harvested and filter sterilized (0.22 µm; Millipore Express PES membrane) from bacterial cultures adjusted to an OD600 of 1. Pooled human immunoglobulin (Privigen; CSL Behring) was preincubated with bacterial supernatants for 30 minutes at 37°C. Immunoglobulin concentrations of 25, 10, and 2.5 mg/mL were used, unless otherwise indicated.
Determination of Minimum Inhibitory Concentration
The minimum inhibitory concentration (MIC) of clindamycin was determined by using the broth microdilution method, as described elsewhere [36]. The assay was performed from midlogarithmic phase cultures in LB with a standard inoculum of approximately 3–5 ×105 cells. The MIC of clindamycin for LAC wt and LAC Δnuc1 was 0.25 mg/L. The clindamycin resistance of the clinical isolates (MRSA 1–3) was tested with the broth microdilution method as well as a standard E test (Clinical and Laboratory Standards Institute guidelines). The MIC of MRSA 1–3 was >256 mg/L. All MIC tests with broth microdilution were performed in duplicate and repeated 3 times.
DNA Degradation Assay
To assess nuclease activity, recombinant micrococcal nuclease (MNase; New England Biolabs) or bacterial supernatants were incubated with calf thymus DNA (1 mg/mL; Sigma-Aldrich Chemie) in reaction buffer (5 mmol/L calcium chloride and 50 mmol/L Tris-HCl; pH 7.9). After 20 minutes at 37°C, the nuclease reaction was stopped by adding 80 mmol/L ethylenediaminetetraacetic acid (pH 8.0; Merck). Next, 10 µL of each sample was separated on a 0.8% agarose gel (0.5 µg/mL ethidium bromide; Sigma-Aldrich Chemie) and visualized with Gel DOC1000 (Bio-Rad Laboratories) and the IC Capture 2.2 image software (The Imaging Source Europe GmbH).
Quantification of DNA Cleavage Using a Molecular Beacon
A 21–base pair imperfect palindrome DNA molecular beacon (5′CGA ATT CCT TTT TGG AAT TCG 3′) bearing a HEX fluorophore at the 5′ and a Black Hole Quencher 1 at the 3′ end was purchased from Microsynth. All reactions were carried out using the molecular beacon at a concentration of 0.2 µmol/L in the reaction buffer. Quantitative analysis was started by addition of nuclease(s) at 37°C and fluorescence (excitation, 530 nm; emission, 575 nm) was recorded every 15 seconds for 25 minutes. The background signal was determined and subtracted from the sample values. The initial digestion rate for the standard was measured from the slope of the linear portion of the time curve.
Isolation of Human Blood-Derived Neutrophils
Primary human neutrophils were isolated from venous blood drawn from healthy donors (BD Vacutainer Systems, K2E, REF 367525) by means of density gradient centrifugation using the Polymorphprep system according to the manufacturer's protocol (Axis-Shield), as reported elsewhere [37].
Blood was taken from healthy donors in strict accordance with the institutional guidelines, according to Declaration of Helsinki principles. The protocol 2010-0126/0 was approved by the Institutional Review Board of the University of Zurich. Written informed consent was received from all participants.
Neutrophil Killing Assay
Neutrophils were seeded in flat 96-well tissue culture plates at a density of 5 × 105 cells per well in Roswell Park Memorial Institute 1640 medium (PAA Laboratories), containing subinhibitory concentrations of clindamycin (subclindamycin; 0.03 µg/mL) or dilution medium as control. For extracellular neutrophil killing assays, neutrophils were prestimulated with 25 nmol/L phorbol 12-myristate 13-acetate (Sigma-Aldrich Chemie) and the phagocytosis inhibitor cytochalasin D (10 µg/mL; MP Biomedicals) for 20 minutes at 37°C with 5% carbon dioxide (CO2). Bacteria grown overnight in the absence or presence of subclindamycin were washed with phosphate-buffered saline and adjusted to an OD600 of 0.4. Bacteria were preincubated for 30 minutes at 37°C with dilution medium, subclindamycin, or 25 mg/mL immunoglobulin and then added directly to the neutrophils at a multiplicity of infection of 0.3. After centrifugation, cells were incubated for 90 minutes at 37°C with 5% CO2. For enumeration of surviving bacteria (in colony-forming units per milliliter), serial dilutions in sterile water were plated on blood agar plates. Bacterial growth in the presence of neutrophils was normalized to bacterial growth under the same conditions in the absence of neutrophils to make sure that the effect is not due to growth differences of the strains in the presence or absence of clindamycin.
NET Degradation Assay
To induce extracellular trap formation, neutrophils were seeded on glass slides (8-mm diameter; Electron Microscopy Sciences) coated with poly-D-lysine (BD Biosciences) and were then stimulated with 25 nmol/L phorbol 12-myristate 13-acetate for 4 hours at 37°C in a CO2 incubator. Supernatants from bacteria grown with or without subclindamycin or supernatants preincubated with 25 mg/mL immunoglobulin were diluted (1:20) and added directly to the NETs. The plates were centrifuged at 538 g for 10 minutes and further incubated for 20 minutes, followed by fixation with 4% paraformaldehyde (Kantonsapotheke Zurich). After washing and blocking with 2% (wt/vol) bovine serum albumin (Sigma-Aldrich Chemie) plus 0.2% Triton X-100 (vol/vol) (Fluka Biochemika) in phosphate-buffered saline (45 minutes at room temperature), NETs were visualized using a primary antibody against the histone H2A-H2B-DNA complex (mouse monoclonal anti-H2A-H2B-DNA; PL2-6; stock 0.9436 mg/mL, diluted 1:1500) [38] or a mouse immunoglobulin G2b (1.3 mg/mL, diluted 1:2100; Thermo Scientific) as respective isotype control antibody. After overnight incubation at 4°C the slides were washed and incubated for 45 minutes at room temperature with the secondary antibody DyLight 488–conjugated goat anti-mouse immunoglobulin G (1:1000; Thermo Scientific). Extracellular DNA and nuclei were counterstained with 4', 6-Diamidin-2-phenylindol (DAPI) dihydrochloride (1:5000; Sigma-Aldrich Chemie). Microscopic images were obtained at room temperature with a confocal inverted microscope (CLSM Leica SP5) and a ×20 (multi-immersion NA 0.7) objective at calibrated magnifications. Images were obtained and processed identically using ImageJ software (version 1.40g). All image acquisition parameters were equal. Experiments were performed in duplicate with 3 biological independent replicates, and ≥3 images captured per slide.
Quantitative Reverse-Transcription Polymerase Chain Reaction Analysis of nuc1 mRNA
Nuc1 transcripts from LAC wt grown with or without clindamycin to postexponential phase (OD600, 2.5 ± 0.1) were assayed by means of quantitative reverse-transcription polymerase chain reaction (qRT-PCR). RNA was isolated with the Ambion TRI Reagent solution (Invitrogen) according to the manufacturer's protocol. Pellets were lysed with lysing matrix tubes (0.1 mm silica spheres lysing matrix B; MP Biomedicals) and the Bead Beater FastPrep Fp120 (Bio 101, Savant) at 6000 rpm for 40 seconds. RNA samples were treated with Ambion Turbo DNA-free Kit (Invitrogen), according to the manufacturer's protocol. Complementary DNA was synthesized from 750 ng of RNA by using an RT enzyme mix (Invitrogen) and 250 ng of random primers (3 µg/mL; Invitrogen). The qRT-PCR was carried out in 96-well plates (iCycler iQ PC plates; Bio-Rad) using the SYBR Green ER qPCR Super Mix and a real-time PCR detection system (iQ5; Bio-Rad Laboratories). Reaction mixtures were prepared using 250 nmol/L primers (listed in Supplementary Data) and 1:30 diluted complementary DNA. GyrB and rpoD were used as housekeeping genes, and the efficiency of each primer pair was tested in triplicate. Differences in mRNA levels were calculated as follows: ΔCt values of gyrB and nuc1 were calculated by normalization of the mean cycle threshold from each biological replicate to the median of the biological replicates without clindamycin. For each sample, the fold change was calculated according to the Pfaffl analysis method [39]. PCR experiments were performed using ≥3 biological replicates, each tested in technical triplicates.
Statistics
For data analyses nonparametric Mann–Whitney U tests, Wilcoxon signed rank tests, or unpaired 2-tailed Student t tests were used. P values were calculated with GraphPad Prism software (Prism 5; 2007). Differences were considered statistically significant at P < .05.
RESULTS
Quantification of Nuclease Activity
To quantify nuclease activity of S. aureus LAC over time, we designed a molecular beacon–based reporter assay. Cleavage of the molecular beacon by a nuclease resulted in an increase in fluorescence (Supplementary DataA). To test the reliability of our reporter system and assess its use for quantification, the molecular beacon was incubated with various concentrations of the commercially available MNase (New England Biolabs). As depicted in Supplementary DataB, the increase in the fluorescence signal was proportional to the MNase concentration with a correlation coefficient of 0.9701.
Inhibition of Extracellular Nuclease (Nuc1) by Clindamycin
S. aureus incubated with subclindamycin has been shown to alter the expression of the extracellular virulence factors protein A, α-hemolysin, and serine protease [31]. To investigate its effects on nuclease activity, LAC wt and LAC Δnuc1 were grown in the presence or absence of subclindamycin to the postexponential phase (for viable cell counts, see Supplementary Data), and extracellular nuclease activity was assessed using our molecular beacon–based reporter assay. Nuclease activity was significantly reduced in LAC wt supernatants from cultures grown in the presence of subclindamycin, compared with the non–clindamycin-exposed control (P < .01; Student t test). The strain LAC Δnuc1 showed no detectable nuclease activity regardless of subclindamycin exposure (Figure 1A). Nuclease activity was also assessed by visualizing degradation of calf thymus DNA on an agarose gel. Untreated LAC wt supernatants degraded calf thymus DNA, whereas supernatants of LAC wt cultures grown with subclindamycin showed only partial degradation. The LAC Δnuc1 supernatant did not show DNA degradation and was not influenced by the presence or absence of subclindamycin (Figure 1B).
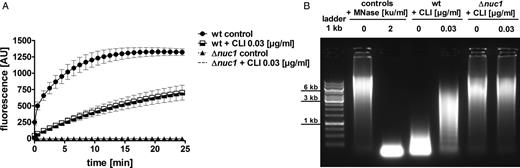
Quantification of Nuc1 activity in Staphylococcus aureus supernatants in the presence or absence of subclindamycin. A, S. aureus cultures grown to postexponential phase in the presence of subclindamycin resulted in a reduction of nuclease activity. DNA cleavage events were measured as increase in fluorescence (AU, arbitrary units). Reduced nuclease activity of LAC wild-type (wt) supernatants in the presence of subclindamycin is indicated by a lower fluorescence signal compared with control without subclindamycin (P < .01 at last measurement time point). Data were pooled from 3 independent experiments, each performed in triplicate (means ± standard errors of the mean) and statistically analyzed with Student t test. B, Representative agarose gel of bacterial culture supernatants of LAC wt and LAC Δnuc1 in the presence or absence of subclindamycin after incubation with calf thymus DNA. Nuclease activity resulted in a smear of degraded DNA; undegraded DNA appeared as a high molecular weight band. Addition of subclindamycin to the LAC wt cultures resulted in less degraded eukaryotic DNA. Abbreviations: ku, Kunitz units; MNase, micrococcal nuclease; CLI, clindamycin.
Dose-Dependent Inhibition of Nuc1 by Immunoglobulin
Supernatants of LAC wt and LAC Δnuc1 were incubated without or with various concentrations of immunoglobulin. The molecular beacon–based reporter assay showed that preincubation with immunoglobulin at a concentration of 25 mg/mL (P < .01; Student t test) or 10 mg/mL (P < .05; Student t test) resulted in dose-dependent inhibition of nuclease activity (Figure 2A). LAC Δnuc1 supernatants displayed no quantifiable nuclease activity regardless of the addition of immunoglobulin. Supernatants were also incubated with calf thymus DNA, and degradation was analyzed by gel electrophoresis. Immunoglobulin inhibited the nuclease activity in a dose-dependent manner in LAC wt supernatants (Figure 2B). No nuclease activity was detected in LAC Δnuc1 supernatants, with or without immunoglobulin.
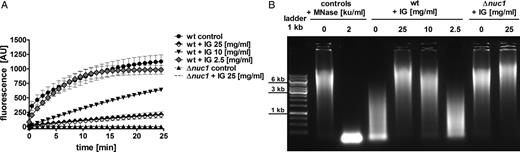
Dose-dependent inhibition of Staphylococcus aureus Nuc1 activity by immunoglobulin (IG). Supernatants of LAC wild-type (wt) and LAC Δnuc1 were preincubated with various amounts of immunoglobulin for 30 minutes at 37°C. A, Nuclease activity in the presence or absence of immunoglobulin was quantified with the molecular beacon–based reporter assay. Immunoglobulin dose-dependent inhibition of Nuc1 is shown from ≥3 independent experiments (means ± standard errors of the mean). Nuclease activity was significantly reduced after preincubation with 25 mg/mL (P < .01) and 10 mg/mL (P < .05) immunoglobulin, compared with control without immunoglobulin. No significant reduction in nuclease activity was observed after preincubation with 2.5 mg/mL immunoglobulin. Statistical analysis using Student t test was performed at the last measurement time point. B, Representative agarose gel of calf thymus DNA after incubation with bacterial culture supernatants of LAC wt and LAC Δnuc1 strains incubated with or without immunoglobulin. In the presence of high concentrations of immunoglobulin (25–10 mg/mL), less DNA degradation was observed, suggesting nuclease inhibition. Abbreviations: AU, arbitrary units; ku, Kunitz units; MNase, micrococcal nuclease.
Reduced NET Degradation Through Nuc1 Inhibition by Subclindamycin and Immunoglobulin
S. aureus Nuc1 degrades NETs, thereby evading innate immune defense [19]. We therefore investigated the inhibitory potential of subclindamycin and immunoglobulin on nuclease-mediated NET degradation. NETs were coincubated with bacterial supernatants from bacteria grown in the presence of subclindamycin, supernatants preincubated with 25 mg/mL immunoglobulin or supernatants without clindamycin or immunoglobulin as controls. NETs incubated with supernatants from subclindamycin showed reduced NET degradation compared with supernatants from bacteria grown without clindamycin. Reduced NET degradation was also detected when we added supernatants preincubated with immunoglobulin (Figure 3). Supernatants of LAC Δnuc1 did not show NET degradation.
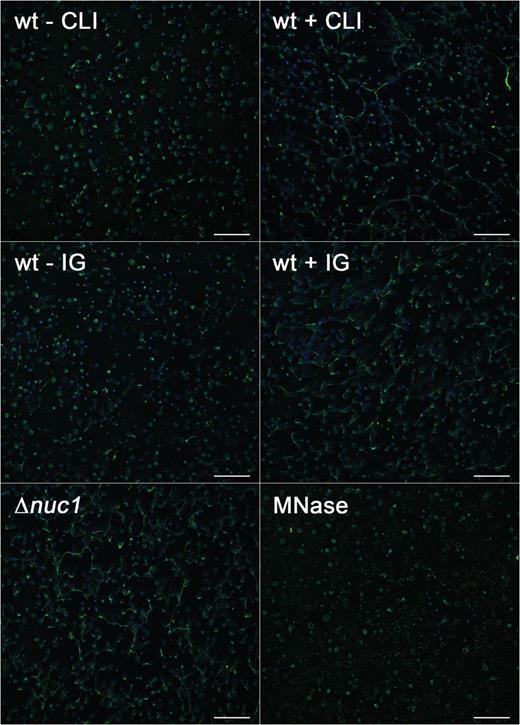
Inhibitory effect of subclindamycin and immunoglobulin (IG) on neutrophil extracellular trap (NET) degradation, which was used as an indicator of nuclease activity. Neutrophils were stimulated with phorbol 12-myristate 13-acetate for NET induction, followed by coincubation with bacterial supernatants. Reduced nuclease activity translated into reduced NET degradation in the presence of subclindamycin or preincubation with immunoglobulin (25 mg/mL). Supernatants of LAC Δnuc1 did not show NET degradation. Micrococcal nuclease (MNase; 4 Kunitz units/mL) was used as a positive control for efficient NET degradation. Representative images are shown. Nuclei were stained with DAPI (blue), and histone-DNA complexes were stained with AlexaFluor 488–labeled antibody (green). Scale bars represent 100 µm. Abbreviations: CLI, clindamycin; wt, wild type.
Enhanced Bacterial Clearance by Neutrophils Due to Reduced Nuclease Activity
Because our results showed that clindamycin and immunoglobulin reduced S. aureus extracellular nuclease activity and subsequent NET degradation, we tested whether addition of subclindamycin or immunoglobulin would also lead to enhanced bacterial clearance in total and in extracellular neutrophil killing assays. Survival of the LAC Δnuc1 strain was significantly reduced in extracellular neutrophil killing compared with LAC wt (Figure 4A). In contrast, no differences in survival were observed between the LAC wt and LAC Δnuc1 strain in total neutrophil killing (Figure 4B). The addition of subclindamycin and immunoglobulin significantly reduced LAC wt survival in total and extracellular neutrophil killing assays (Figure 4A and 4B).
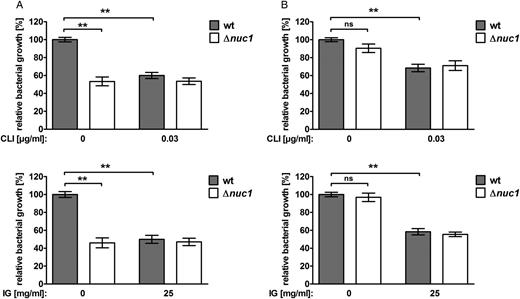
Reduced nuclease activity and enhanced bacterial clearance by neutrophils in the presence of subclindamycin and immunoglobulin. A, In extracellular neutrophil killing experiments, LAC wild-type (wt) showed significantly reduced growth when either treated with subclindamycin or preincubated with 25 mg/mL immunoglobulin, compared with bacteria grown without subclindamycin or immunoglobulin (control). LAC Δnuc1 was not affected by addition of subclindamycin or immunoglobulin. B, In total neutrophil killing experiments, LAC wt and LAC Δnuc1 grown with subclindamycin or preincubated with immunoglobulin showed significantly reduced growth compared with bacteria without subclindamycin or immunoglobulin (control). No significant difference in relative growth was observed comparing LAC wt and LAC Δnuc1. Data are presented as relative bacterial growth compared with LAC wt in the presence of neutrophils and absence of clindamycin. Pooled data from 2 independent blood donors (n = 12 in A upper graph; n = 9 in A lower graph and B upper and lower graph means ± standard errors of the mean), analyzed with the Wilcoxon signed rank test (**P < .01). Abbreviations: IG, immunoglobulin; CLI, clindamycin; NS, not significant.
Pharmacological Reduction of Nuclease Activity by Clindamycin and Reduced nuc1 Transcription
We next investigated whether the reduction of nuclease activity on exposure to subclindamycin was associated with lower nuc1 expression levels. We performed qRT-PCR on nuc1 of LAC wt grown in the presence or absence of subclindamycin to postexponential phase. The LAC wt strain grown in the presence of subclindamycin showed an approximately 7-fold decrease in nuc1 mRNA transcript compared with the LAC wt strain grown without subclindamycin (Figure 5).

Down-regulation of Nuc1 transcription in response to subclindamycin. Relative transcript levels of nuc1 derived from LAC wild-type (wt) cultures grown to postexponential phase with and without subclindamycin were determined by means of quantitative reverse-transcription polymerase chain reaction. Fold change ratios were calculated by normalizing complementary DNA levels of nuc1 against one of the reference genes (gyrB or rpoD). Data are presented as median fold change of nuc1 gene expression, and error bars indicate interquartile ranges for each data set. Experiments were performed with ≥4 independent samples, using the Mann–Whitney U test; **P < .01. Abbreviation: CLI, clindamycin.
No Inhibition of Nuclease Activity and nuc1 Transcription by Clindamycin in Clindamycin-Resistant Strains
Our results showed a regulatory effect of clindamycin on nuc1 expression in our laboratory strain LAC wt. Therefore, we wanted to know whether this is also the case for clindamycin-resistant clinical isolates of S. aureus (MRSA 1–3). Quantification of nuclease activity showed no significant change on incubation with 0.03 or 0.6 µg/mL clindamycin in all tested clinical isolates (Figure 6A). We also performed qRT-PCR on nuc1, and in line with our nuclease activity data, we did not observe alterations of nuc1 mRNA levels in our clindamycin-resistant clinical isolates on incubation with 0.03 or 0.6 µg/mL clindamycin (Figure 6B).
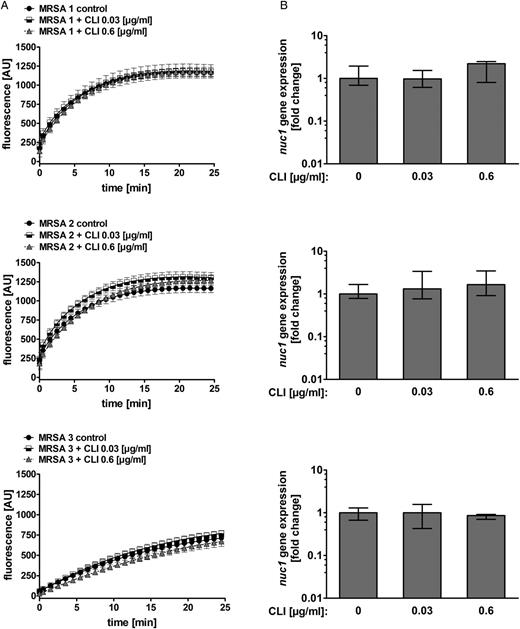
Effect of clindamycin on nuclease activity and nuc1 transcription in clindamycin-resistant methicillin-resistant Staphylococcus aureus (MRSA) isolates. A, Clindamycin-resistant S. aureus cultures (MRSA 1, 2, and 3) grown to postexponential phase with 0.03 and 0.6 µg/mL clindamycin did not result in significant alteration of nuclease activity. DNA cleavage events were measured as increase in fluorescence (AU, arbitrary units). Data were pooled from 3 independent experiments, each performed in triplicate (means ± standard errors of the mean). B, Relative transcript levels of nuc1 derived from MRSA 1, 2, and 3 grown without clindamycin and 0.03 and 0.6 µg/mL clindamycin were determined by means of quantitative reverse-transcription polymerase chain reaction. Fold change ratios were calculated by normalizing complementary DNA levels of nuc1 against one of the reference genes (gyrB or rpoD). Data are presented as median fold change of nuc1 gene expression, and error bars indicate interquartile range for each data set. Experiments were performed with ≥3 independent samples. Abbreviation: CLI, clindamycin.
DISCUSSION
Our work shows that addition of immunoglobulin and subclindamycin to clindamycin-susceptible MRSA directly blunted S. aureus nuclease activity, translating into enhanced extracellular clearance of S. aureus by neutrophils. Beneficial effects were also observed in total neutrophil killing assays, indicating that subclindamycin and immunoglobulin may affect not only extracellular nuclease but also other virulence factors.
To treat MRSA infections, the bactericidal cell wall–active antibiotic vancomycin is typically given empirically. However, persistent bacteremia may be found despite therapeutic vancomycin concentrations in serum [6], illustrating the difficulties encountered in treating MRSA infections. In addition, antibiotic resistance is increasing [40].
Both clindamycin and immunoglobulin are being used empirically in clinics [41, 42] to treat bacterial infections. In vitro antagonism of clindamycin and vancomycin against S. aureus has been described elsewhere [43, 44], but the clinical relevance of this in vitro antagonism has not yet been prospectively analyzed. Protein synthesis inhibitors are frequently used owing to their excellent bioavailability and their ability to overcome the reduced antibacterial efficiency of penicillins on stationary bacteria, also known as the Eagle effect [45]. Production of the virulence factor Panton-Valentine leukocidin was shown to be down-regulated by the protein synthesis inhibitor linezolid in a rabbit model of necrotizing pneumonia [46]. In addition to translational inhibition, sub-MIC protein synthesis inhibitors were shown to indirectly regulate virulence factors on the transcriptional level [31, 47–49]. Sub-MIC antibiotic levels are common during infections, owing to reduced perfusion in necrotic tissue or in abscesses.
S. aureus Nuc1 is one of the most important staphylococcal virulence factors and plays a key role in evasion of the innate immune system by facilitating degradation of NETs, promoting survival and pathogenicity [19]. To study the effect of protein synthesis inhibitors or immunoglobulin on nuclease activity, we designed a sensitive and easy to use high throughput assay. This assay allowed direct quantification of nuclease activity using a basic real-time PCR machine. This enabled us to investigate the impact of subclindamycin on Nuc1 activity. We found that subclindamycin significantly lowered the transcription of the nuc1 gene and subsequently Nuc1 activity. For clinicians, this observation defuses concerns that subclindamycin could eventually result in paradoxically boosting virulence factor expression, as was shown for certain virulence factors of group A streptococci [50]. We also showed that in the clinical clindamycin-resistant S. aureus strains, subclindamycin did not inhibit Nuc1 expression or nuclease activity. Because clindamycin neither inhibits bacterial growth nor attenuates the bacterial virulence factor Nuc1, it will probably not influence infections due to clindamycin-resistant S. aureus.
We have now shown that immunoglobulin reduced S. aureus nuclease activity and that such inhibition directly translated into enhanced bacterial clearance. Based on these findings, addition of immunoglobulin should be considered as complementary treatment strategy for severe staphylococcal infections. Their addition to current state-of-the-art antibacterial treatment, usually consisting of cell wall–active antibiotics such as vancomycin for MRSA, should help control fulminant S. aureus infection more efficiently. In vivo, however, owing to their large molecular size, immunoglobulins might not efficiently diffuse toward sites of infection, and they might well be less efficient than our findings suggest. Further studies will be required to address the beneficial effects mediated by immunoglobulin in vivo.
Taken together, we provide rational evidence supporting and expanding current treatment concepts of treating S. aureus infections with a cell wall–active antibiotic combined with virulence factor–inhibiting agents, such as protein synthesis inhibitors or immunoglobulin. Our data also defuse concerns that subinhibitory concentrations of protein inhibitors might paradoxically boost virulence factor expression by showing that subclindamycin efficiently blunted Nuc1 in susceptible S. aureus strains, resulting in enhanced bacterial clearance by neutrophils.
Notes
Acknowledgments. Imaging was performed with equipment maintained by the Center for Microscopy and Image Analysis, University of Zurich.
Financial support. This work was supported by grants from the Swiss National Science Foundation (grants 310030_146295/1 to A. S. Z. and PZ00P3_136639 to R. A. S.), the Zentrum für Klinische Forschung, and the Stiftung für Medizinische Forschung, University of Zurich.
Potential conflict of interest. All authors: No reported conflicts.
All authors have submitted the ICMJE Form of Disclosure of Potential Conflicts of Interest. Conflicts that the editors consider relevant to the content of the manuscript have been disclosed.
References
Author notes
Presented in part: FEMS 2013, Fifth Congress of European Microbiologists, Leipzig Germany, 21–25 July 2013 (abstract 501); Joint Annual Meeting 2013 of the Swiss Society for Infectious Diseases, Hospital Hygiene, Tropical and Travel Medicine, Lugano, Switzerland, 30–31 May 2013.
Present affiliation: Department of Medical Microbiology Helmholtz Centre for Infection Research, Braunschweig, Germany.