-
PDF
- Split View
-
Views
-
Cite
Cite
Leslie A. Kalish, Kenneth McIntosh, Jennifer S. Read, Clemente Diaz, Sheldon H. Landesman, Jane Pitt, Kenneth C. Rich, William T. Shearer, Katherine Davenny, Judy F. Lew, for the Women and Infants Transmission Study, Evaluation of Human Immunodeficiency Virus (HIV) Type 1 Load, CD4 T Cell Level, and Clinical Class as Time-Fixed and Time-Varying Markers of Disease Progression in HIV-1—Infected Children, The Journal of Infectious Diseases, Volume 180, Issue 5, November 1999, Pages 1514–1520, https://doi.org/10.1086/315064
- Share Icon Share
Abstract
Human immunodeficiency virus (HIV) type 1 RNA load, CD4 T cell level, and Centers for Disease Control and Prevention (CDC) clinical class history were measured as potential correlates of a CDC class C diagnosis or death in 165 HIV-1—infected children followed from birth. These covariates were assessed at fixed “landmark” ages from 6 to 24 months and were also assessed as time-varying values. Virus load was associated with progression in all analyses, even after adjusting for immunologic and clinical status. This confirms its importance for monitoring pediatric disease progression. CD4 T cell level was associated with disease progression in time-varying but not in adjusted landmark analysis, suggesting that CD4 cells reflects immediate risk more than long-term risk. The distinction between clinical class B and lower classes is prognostic during the first 18 months of life; class C versus classes N/A/B becomes more important as the patient ages. Virologic, immunologic, and clinical status all provide information regarding disease progression risk.
Several prospective analyses of human immunodeficiency virus (HIV) type 1—infected adults have established the importance of plasma HIV-1 RNA load and CD4 T cell level as independent predictors of HIV-1 disease progression or death. Most studies using prospective time-to-event statistical methods to relate both “baseline” HIV-1 RNA load and CD4 T cell levels to subsequent disease progression risk were initially done in adult populations [1–8]. Use of these methods in pediatric HIV-1 disease is usually more recent and based on smaller sample sizes with shorter follow-up [9–13]. Some of these studies [12–18] have utilized cohorts prospectively followed from birth to focus on characteristics defined very early in life, including maternal characteristics, as potential prognostic factors.
This study evaluated conventional measures of virologic, immunologic, and clinical status as potential prognostic factors for pediatric HIV-1 disease progression. We assessed the utility of measuring patient characteristics only once, at a fixed baseline age, and the utility of considering the covariates as time-varying markers, by updating patient characteristics at each visit. We also assessed whether the associations between patient characteristics and the risk of progression are similar during the first 18 months of life and after 18 months of age.
We used data from a pediatric cohort followed prospectively from birth, the Women and Infants Transmission Study (WITS) cohort. Previous WITS publications have described the natural history of clinical and immunologic disease progression [18], the history of plasma RNA HIV-1 load [14], the prognostic value of infant virus load [14, 15], and several other infant and maternal characteristics [15] measured in the first few months of life for predicting rapid disease progression.
In the time-fixed covariate or “landmark” approach, the risk of HIV-1 disease progression is related to patient characteristics that are measured at the landmark or “baseline” age. In this analysis, covariate values were determined as of the baseline date, which was considered “time zero” for measuring time to the end point (disease progression or death). Many studies of seroprevalent cohorts of adults and children have used this approach, with baseline arbitrarily defined as the date the subject entered the study or the date a virus load measure was first obtained [1, 3–11].
The other analysis approach uses time-varying covariates, which, rather than being fixed at baseline levels, are subject to change as the patient ages. This approach more closely reflects the natural history of the disease and clinical practice in which patient characteristics (virologic, immunologic, and clinical status) are monitored at each patient visit. The time-varying covariate analysis relates risk of disease progression to the most recently measured patient characteristics rather than to characteristics measured at baseline.
It has been suggested that perinatally infected children have 2 possible patterns of disease progression: rapid progression, typically manifesting as progression to AIDS within ∼18 months, and slow progression, with a much lower progression rate that is more constant over time [19–23]. Accordingly, we focused primarily on 2 landmark ages, 6 and 24 months, to explore whether prognostic information is similar for patient characteristics measured 6 months into each of these 2 time periods. (Covariate values at the “true” baseline, birth, have little prognostic utility in perinatally infected children followed from birth. For example, at birth all infants are in the earliest clinical stage, most are in the earliest immunologic stage, and most have undetectable HIV-1 RNA.) Similarly, we assessed whether the magnitude of associations between time-varying covariates and outcome is uniform over 2 age periods, <18 and ⩾18 months.
Methods
The WITS is a multicenter, longitudinal study of the natural history of HIV-1 infection in pregnant women and their infants [24]. Participants are enrolled at study centers in Massachusetts (Boston and Worcester), Illinois (Chicago), Texas (Houston), New York (2 centers in New York City), and Puerto Rico (San Juan). Enrollment began in December 1989. This analysis includes all children who were found to be HIV-1 infected based on study visits through 31 May 1997.
Whole blood was collected by venipuncture in Vacutainer tubes (Becton Dickinson, Franklin Lakes, NJ) containing heparin (>90% of samples) or EDTA. Peripheral blood specimens were routinely collected, and HIV-1 cultures were done [25] at or shortly after birth and at ages 1, 2, 4, 6, 9, and 12 months and every 6 months thereafter. In addition, the protocol called for blood collection at age 15 months until December 1993. A child was considered to be infected if peripheral blood mononuclear cell cultures for HIV-1 were positive at ⩾2 visits [26].
Plasma HIV-1 RNA was measured in 3 laboratories by the Amplicor HIV-1 monitor test (Roche Diagnostic Systems, Branchburg, NJ) according to the manufacturer's instructions and the AIDS Clinical Trials Group (ACTG) quality assurance recommendations, as described elsewhere [14]. CD4 T cell levels were quantitated by standard flow cytometry techniques at local laboratories certified by the ACTG. Children were categorized according to the Centers for Disease Control and Prevention (CDC) 1994 revised classification system for HIV infection in children [27] as having no, moderate, or severe immunologic suppression (immunologic stages 1, 2, and 3, respectively) and as having no, mild, moderate, or severe clinical symptoms (clinical classes N, A, B, and C, respectively). Immunologic staging is based on both absolute CD4 T cell count and percentage. Clinical staging was conducted retrospectively until 1 April 1995 and prospectively thereafter, as described elsewhere [18]. Data from follow-up visits initiated through 31 May 1997 were included in the analysis.
The end point for all analyses was a CDC clinical class C diagnosis or HIV-related death. In the landmark analyses, only children who reached the landmark age were eligible for the corresponding analysis. The disease progression end point was defined as the first clinical class C diagnosis or death occurring after the landmark age. In the time-varying covariate analysis, the end point was a first clinical class C diagnosis or HIV-related death occurring after birth.
Covariates in the landmark analysis were defined from patient characteristics measured most recently prior to the landmark age. Similarly, in the time-varying analysis, covariates at any age were defined from patient characteristics measured most recently prior to that age. Plasma HIV-1 load was analyzed on a logarithmic (base 10) scale, with values below the limit of quantification (400 copies/mL) imputed as 400 and measurements from the birth visit excluded. CD4 T cell count was analyzed both as a categorical covariate, with categories based on both CD4 T cell counts and percentages as defined by the CDC immunologic staging system [27], and as a continuous covariate based on CD4 T cell percentage. Clinical class was categorized according to the CDC clinical staging system [27]. In the landmark analysis, we included children in clinical class C at the landmark age in the analysis and therefore could assess the prognostic value of a prior class C condition for the risk of progression to a new class C diagnosis. Because the time-varying analysis had first class C diagnosis after birth as an end point, we were unable to include class C as a comparison group in that analysis. With both approaches, however, we could compare clinical class B versus class N or A for their risks of further progression to class C.
Kaplan-Meier estimates, log-rank tests, and proportional hazards models with fixed covariates were used in the landmark analysis [28, 29]. Proportional hazards models with time-varying covariates were used in the time-varying covariate analysis [29]. To enable a time-varying covariate, Z(t), to have different associations before and after 18 months of age, it was replaced with 2 time-varying covariates: Z1(t) = {Z(t)[I(t <18)]} and Z2(t) = {Z(t)[I(t ≥ 18)], where I(x) is the indicator function. I(x) = 1 if x is true and 0 if x is false. Equality of the 2 corresponding parameters was tested with a Wald test. All P values were 2-sided and considered significant at <.05.
Results
As of 31 May 1997, 165 infants were known to be infected with HIV-1. Median clinical follow-up was 36 months (maximum, 7 years). Sixty-one children had reached a disease progression end point (⩾ 1 clinical class C diagnosis or HIV-related death); for 5 of the 61, the end point was HIV-related death. The Kaplan-Meier estimate of disease progression by 18 months was 30%. Of the 165 children, 146 had ⩾1 HIV-1 RNA load measurements (total, 1095 measurements; average, 7.5/child).
Excluding antiretroviral therapy received during the first 2 months of life, which could have represented prophylaxis against perinatal transmission, 66% received antiretroviral therapy during the first 12 months of life, 79% during the first 24 months, and 88% during the first 36 months. Because the first protease inhibitors to be approved by the Food and Drug Administration for use in children (nelfinavir and ritonavir) were not approved until March 1997, within the last 3 months of follow-up for this analysis, little protease inhibitor use is represented in this data set.
Landmark analysis
The numbers of children still being followed and the numbers with a subsequent disease progression end point after landmark ages 0, 6, 12, 18, and 24 months, respectively, are 165 (63 with subsequent end point), 148 (56), 127 (37), 110 (33), and 99 (24). Table 1 and figure 1 show univariate analyses from the landmark analysis with 6- and 24- month landmarks. The same analyses were also done using 12- and 18-month landmarks (data not shown). There were too few end points for meaningful analysis for landmarks after 24 months. Since Kaplan-Meier plots can be constructed only with categorical covariates, HIV-1 RNA load was dichotomized, using the median value among those at risk at age 6 months to define categories.
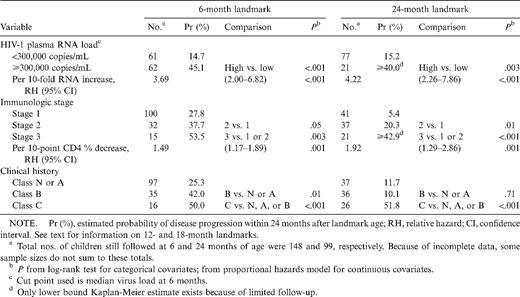
Univariate landmark analysis with 6- and 24-month landmarks in human immunodeficiency virus (HIV)—1—infected children.
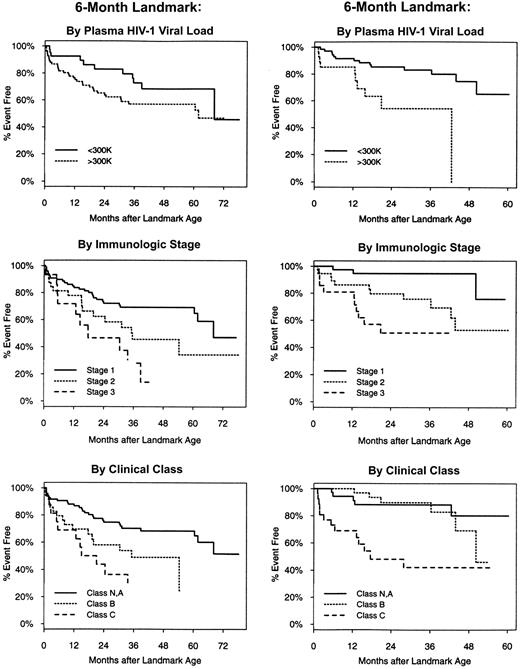
Time to next clinical class C diagnosis or death measured from 6- and 24-month landmarks, stratified by plasma human immunodeficiency virus (HIV)—1 RNA load, immunologic stage, and clinical class.
When considered in unadjusted analysis, virologic, immunologic, and clinical status were each associated with disease progression subsequent to all landmark ages considered. HIV-1 RNA load and CD4 T cell level were significant when treated as either categorical or continuous covariates. The distinction between being in class B and being in class N or A at age 18 or 24 months had no prognostic value (P = .18 and P = .71, respectively), was marginally significant at 12 months (P = .07), but was significant at age 6 months (P = .01). At all landmark ages, a history of a class C condition elevated the risk of subsequent progression.
Results from multivariate analysis using 6- and 24-month landmarks are shown in table 2. Even after adjusting for immunologic and clinical status, HIV-1 RNA load at either 6 or 24 months was significantly associated with subsequent progression. However, immunologic status was not associated in adjusted analysis when modeled as either a categorical or continuous covariate. Having been diagnosed with a clinical class C condition prior to age 18 or 24 months elevated the risk of another class C diagnosis or death. However, the adjusted relative hazard (RH) associated with a clinical class C history at 12 months was only marginally significant (adjusted RH, 2.13; P = .08); at the 6-month landmark, a class C diagnosis history was not associated with subsequent risk (adjusted RH, 1.59; P = .27). If an additional term was added to the multivariate models to compare class B versus lower classes after adjusting for virus load and immunologic stage, a class B history was associated with smaller risks and larger P values at older landmark ages (adjusted RH at 6 months, 2.28, P = .04; adjusted RH at 24 months, 1.28, P = .68).
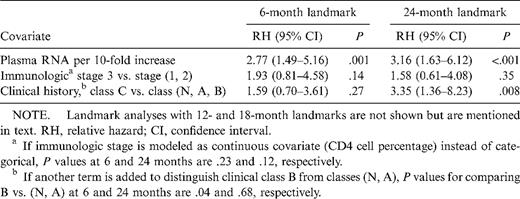
Multivariate landmark analysis with 6- and 24-month landmarks in human immunodeficiency virus (HIV)—infected children.
Time-varying covariate analysis
Results of univariate models with time-varying covariates are shown in table 3. Higher HIV-1 RNA load, immunologic stage 3, and clinical class B individually were associated with disease progression in univariate analysis. All 3 covariates remained significant in adjusted analysis as well (table 3). Adding terms to the multivariate model to compare immunologic stages 2 versus 1 or to compare clinical classes A versus N did not significantly improve the model (P = .13 for immunologic stage; P = .19 for clinical class).
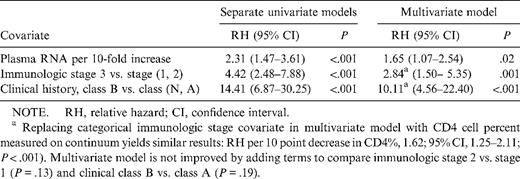
Time-varying covariate analysis with proportional hazards model in human immunodeficiency virus (HIV)—infected children.
The models summarized in table 3 assume that associations between each covariate and disease progression risk are the same at any age. In order to test the hypothesis that the adjusted associations seen in table 3 are similar before and after 18 age months, we expanded the model to allow for different RHs before and after this time point. The results (table 4) suggest that virologic and immunologic status have similar associations with progression at <18 and ⩾18 months of age. However, at or after 18 months, the distinction between clinical class B and class N or A becomes much less important as a marker of risk.
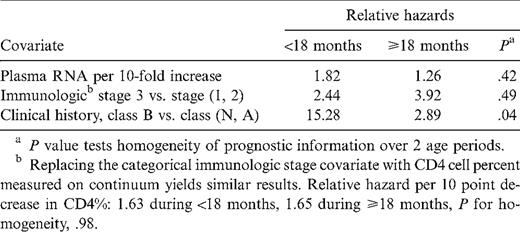
Multivariate time-varying covariate proportional hazards model, extended to allow relative hazards to differ during the first 18 months versus later in life.
Discussion
This study is unique in assessing plasma HIV-1 RNA load, CD4 T cell level, and clinical status simultaneously as potential risk factors for HIV-1 disease progression; in evaluating the factors both as baseline covariates and as time-varying covariates; and in applying these methods to a pediatric cohort followed prospectively from birth rather than a seroprevalent cohort. One limitation of the study was that follow-up was done before widespread use of highly active antiretroviral therapy (HAART) in pediatric HIV-1 disease. Although this makes results easier to interpret, since treatment effects are limited, further studies are required to determine whether conclusions would be altered with the widespread use of HAART. Although many children received antiretroviral therapy, adding time-varying indicator variables for antiretroviral history to multivariate models had only negligible effects on results and conclusions (data not shown).
Another limitation is the relatively small sample size and follow-up compared with those in some other studies. Statistical power in time-to-event analyses is more a function of the number of events than of total sample size. There were 61, 56, and 24 events, respectively, in the time-varying analysis and in the 6- and 24-month landmark analyses. This contrasts with 12 and 33 events in other pediatric cohorts followed from birth with HIV-1 RNA load and time-to-progression data [12, 13] and with 22, 92, and 135 events in the 3 seroprevalent pediatric cohort studies [9–11].
A major difference between the 3 pediatric studies of seroprevalent cohorts [9–11] and the present analysis is the age range studied. The mean or median ages at entry to the seroprevalent studies ranged from ∼3 to 5 years, with mean or median follow-up duration of 2–5 years. The WITS data and other cohorts followed from birth [12, 13] are much more informative about the first few years of life. All 3 cohorts have median follow-up to ∼3 years of age. Since this age is near the time of primary infection and since the natural history of virus load in perinatally infected children appears to stabilize much more slowly after primary infection than in adults [9, 10, 12–14], this could explain some differences in conclusions (discussed below).
The 2 types of analyses in this study are sensitive to different types of associations between covariates and outcome. It is helpful to understand some properties of the Cox proportional hazards model analysis in order to interpret these analyses. One formulation of the analysis is that it compares the covariates of each “case” (a patient who reaches an end point) with the average covariates of an appropriately chosen “control” group (all patients still at risk for the end point at the same age as the case patient's event time) [29]. The individual case/control comparisons are then combined. In the time-varying covariate analysis, the covariates for each case/control comparison are defined from characteristics measured most recently prior to the case patient's age at the time of the event, whereas in the time-fixed analysis, the covariates are defined at baseline (which could be years before the end point). Put another way, the time-varying covariate analysis relates covariates with events that occur soon after the covariates are measured, whereas in the time-fixed analysis, the outcomes may occur years after the covariates are measured. Thus, strong time-varying and time-fixed covariates are reflective of short-term and long-term associations with outcome, respectively. These concepts and methods can be useful in guiding other analyses of HIV-1 disease progression as well as in interpreting published studies.
HIV-1 RNA load has a strong association with progression in all analysis. This confirms its importance both as a short-term marker of current disease progression risk and as a long-term predictor. These associations remain significant even after adjusting for the patient's immunologic and clinical stage (although clinical stage is adjusted for in slightly different ways in the 2 types of analyses). The associations are uniform at ages younger and older than 18 months. Other pediatric cohorts also have found HIV-1 RNA load to be associated with disease progression [9–13].
Immunologic stage, as measured by CD4 T cell level, is a significant predictor in the multivariate time-varying covariate analysis, uniformly at <18 and ⩾18 months of age, and as a time-fixed covariate in univariate analysis. However, after adjusting for virologic and clinical status, immunologic stage does not contribute significantly in the time-fixed covariate landmark analysis, although the direction of the association is in the expected direction, with lower CD4 T cell level associated with higher risk. These results suggest that the CD4 T cell level is more a marker of short-term risk than a long-term predictor of progression over the subsequent few years. In contrast, the 3 seroprevalant cohort studies, with a median baseline age of 2–5 years, all reported significant CD4 effects at baseline even after adjusting for virus load [9–11]. Whether this discrepancy is due to differences of age or statistical power requires further study. Of the 2 cohorts followed from birth, one study did not assess CD4 cells as a prognostic factor [13], and the other yielded mixed results [12].
As a time-varying marker of risk, CD4 T cell level was of greatest value in its lower range (i.e., higher immunologic stage) in this analysis. However, the distinction between immunologic stages 1 and 2 may become significant with greater sample size and follow-up.
Even after adjusting for plasma RNA HIV-1 load and CD4 T cell level, a patient's clinical stage provides additional information about progression risk. At <18 months of age, children in clinical class B are at much higher short-term risk of progressing to clinical class C or death than those in class N or A. This finding extends the results of Diaz et al. [18] by demonstrating that the association holds even after adjusting for viral and immunologic status. After age 18 months, the distinction between class B and class N or A becomes less informative. Having had a class C diagnosis is a prognostic indicator for further disease progression (another class C diagnosis), especially at or after 18 months of age. At age 6 months, a class C diagnosis history does not provide information regarding long-term risk in adjusted analysis. Further modeling (results not shown) showed that virus load was the primary confounder explaining this result. Therefore, a class C diagnosis very early in life in an infant with low virus load does not indicate a poor prognosis.
In conclusion, the virologic, immunologic, and clinical status over time each provide independent information regarding HIV-1 disease progression risk in children. This study confirms the important role of HIV-1 RNA load in monitoring pediatric patients. Even after adjusting for immunologic and clinical stage, virus load provided additional information regarding short- and long-term risk at all ages studied. The observation that immunologic stage is a stronger independent predictor of short-term risk than long-term risk suggests that CD4 T cell level reflects “current” disease stage more than it predicts future disease. Finally, reaching CDC clinical class B at an early age is an independent marker of further progression. The lower end of the classification system (classes N, A, B) does not appear to be as informative at older ages. In contrast, after the first several months of life, having an AIDS diagnosis is a strong independent predictor of risk.
WITS Investigators, Coordinators, Program Officers, and Funding Sources
Clemente Diaz and Edna Pacheco-Acosta (University of Puerto Rico, San Juan; AI-34858); Ruth Tuomala, Ellen Cooper, and Donna Mesthene (Boston/Worcester, MA; AI-34856); Jane Pitt and Alice Higgins (Columbia Presbyterian Hospital, New York; AI-34842); Sheldon Landesman, Hermann Mendez, and Gail Moroso (State University of New York, Brooklyn; HD-8-2913, HD-25714); Kenneth Rich and Delmyra Turpin (University of Illinois at Chicago; AI-34841); William Shearer, Celine Hanson, and Norma Cooper (Baylor College of Medicine, Houston; AI-34840); Mary Glenn Fowler (National Institute of Allergy and Infectious Disease, Bethesda, MD); Robert Nugent (National Institute of Child Health and Human Development, Bethesda); Vincent Smeriglio (National Institute on Drug Abuse, Rockville, MD); and Sonja McKinlay, Leslie Kalish, and Susan Ellis (New England Research Institutes, Watertown, MA; AI-35161).
Acknowledgments
We thank Beverly Dale for support and Roche Molecular Systems for providing the Amplicor HIV-1 monitor test kits.
References
Presented in part: 12th World AIDS Conference, Geneva, Switzerland, 28 June–3 July 1998 (abstract 13368).
Informed consent was obtained from the women and from the parents or guardians of the children in this study. Human experimentation guidelines of the US Department of Health and Human Services and of the authors' institutions were followed.
Grant support: National Institute of Allergy and Infectious Diseases (AI grants), National Institute of Child Health and Human Development (HD grants).
Author notes
Present affiliation: Department of Pediatrics, University of Florida, Gainesville.
Principal investigators, study coordinators, and program officers (with funding sources) are listed following the text.