-
PDF
- Split View
-
Views
-
Cite
Cite
Jan Šafanda, Philipp Heidinger, Helmut Wilhelm, Vladimir Čermák, Fluid convection observed from temperature logs in the karst formation of the Yucatán Peninsula, Mexico, Journal of Geophysics and Engineering, Volume 2, Issue 4, December 2005, Pages 326–331, https://doi.org/10.1088/1742-2132/2/4/S05
- Share Icon Share
Abstract
Temperature–depth profiles, obtained during three campaigns of temperature logging in boreholes of the Yucatán Peninsula in the period 2002–2004, display the effects of thermal fluid convection. These effects are most pronounced in the uppermost part of the 1.5 km deep borehole Yaxcopoil-1 drilled within the Chicxulub impact crater. The convective zone is clearly discernible in all three profiles measured here in March 2002, May 2003 and February 2004. The loggings have revealed a gradual downward propagation of the convective features from the uppermost 145 m of the temperature profile to 230 m between the first and second loggings (with a propagation rate of 6 m/month) and to 265 m between the second and third loggings (4 m/month). A signature of the fluid convection is also evident in all other temperature logs in the area measured during the 2003 campaign, namely in four UNAM boreholes 2, 5, 7 and 8, three hydrogeological boreholes 1A, 1B and 1C, in a borehole at the meteorological observatory Mérida and in the cenote Ucil. The fresh/salt water interface is clearly visible in most of the logs as a zone of increased temperature gradient. The varying intensity of the convective features among the individual logs seems to be correlated with the borehole position relative to the impact structure.
1. Introduction
Extended geophysical and geological research on the 65 Ma old impact crater Chicxulub on the Yucatán Peninsula, Mexico (Hildebrand et al1991) started in the 1990s. The research on the impact structure with a diameter of 180–200 km and a centre at the port of Chicxulub (figure 1) involved drilling eight cored UNAM (Universidad Nacional Autónoma de México) boreholes inside the crater and in its immediate vicinity (Urrutia-Fucugauchi 1996) with a depth range between 63 m (UNAM 4) and 703 m (UNAM 7) and was promoted greatly by drilling the 1.5 km deep corehole Yaxcopoil-1 (Yax-1) of the Chicxulub Scientific Drilling Project (Urrutia-Fucugauchi et al2004), a part of the International Continental Deep Drilling Programme. Three of the UNAM boreholes (UNAM-5, 6 and 7) and Yax-1 sampled impact breccias below a several hundred metre thick cover of Tertiary carbonates (Urrutia-Fucugauchi et al2004, Rebolledo-Vieyra and Urrutia-Fucugauchi 2004). The cored boreholes provided an opportunity to carry out basic geothermal research on the northwest Yucatán. After the first preliminary results from the UNAM boreholes (Flores-Márquez et al1999) and from about thirty 50–100 m deep boreholes drilled for explosive seismic wave generation in 1996 (Matsui et al1998), the detailed study of the petrophysical properties of the Yax-1 drill-core was carried out together with its precise temperature logging repeated nine times in the period March 2002–February 2004 (Wilhelm et al2004, 2005, Popov et al2004). The heat flow determined for the Yax-1 site is surprisingly high, 70.5 ± 1.9 mW m-2, and constant throughout the investigated depth section 400–1400 m of the borehole (Wilhelm et al2005). During the second logging campaign in May 2003, the temperature was measured not only in Yax-1 but also in four then existing UNAM boreholes 2, 5, 7 and 8, in three hydrogeological boreholes 1A, 1B and 1C, in a borehole on the grounds of the meteorological observatory in Mérida and in the cenote Ucil (figure 1). It turned out that except for Yax-1 and UNAM 5, the boreholes are not suitable for heat flow determinations, because the temperature logs in their depth range of 35–344 m bear the signature of groundwater convection indicating the existence of a large non-conductive component of the heat transport. This paper focuses on the character and possible causes of the observed convective features.
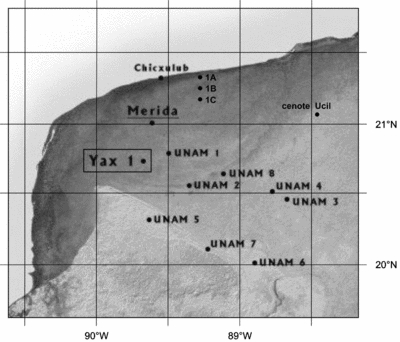
A map of the study area on the Yucatán peninsula (modified from JPL Planetary Photo Journal image #PIA03379) with the positions of the boreholes UNAM 1–8, Yaxcopoil-1, Mérida-observatory, 1A, 1B, 1C and the cenote Ucil.
2. Geological setting and hydrogeology of the area
The northwest part of the Yucatán Peninsula (figure 1) is a flat carbonate platform, underlain by several hundred metres thick sub-horizontal Tertiary and Quaternary formations of almost pure carbonate rocks and evaporates. They represent a mature karst formation with a well-developed system of interconnected caverns and conduits (Steinich and Marín 1997). The rocks display all types of dissolution voids ranging in size from the order of centimetres up to caverns with diameters of the order of tens of metres. The presence of the voids of considerable size implies that groundwater flow is predominantly determined by this secondary porosity. The unconfined aquifer is, in general terms, characterized by high permeabilities and a very low hydraulic gradient. Freshwater is present below the unsaturated zone as a thin lens with a thickness of 15 m near the coast, 45 m at Mérida, 50–80 m at Yaxcopoil and approximately 120 m at 90 km from the coast (Steinich and Marín 1996). It is underlain by the saltwater intrusion.
The area is typical for sinkholes, locally called ‘cenotes’, which are groundwater-filled dissolution and collapse shafts of Pleistocene age formed when the sea level was lower. Some of them are more than 100 m deep. A conspicuous geomorphologic phenomenon is the Ring of Cenotes, a band of high cenote density, approximately 5 km wide, with a radius of 70–80 km centred around the port of Chicxulub. The Ring represents a zone of high permeability (Escolero et al2000) and it is assumed (Steinich and Marín 1996) that it forms a subsurface river system with the water draining towards it and discharging into the sea at its intersection points with the coastline. Boreholes UNAM 2 and 8 are located within it (figure 1). Although the origin of the Ring remains uncertain, the link to the Chicxulub crater seems clear (Perry et al1995). One of the indications is its association with the largest of the concentric zones appearing on the map of the horizontal gravity gradients related to structural elements of the buried crater (Hildebrand et al1995).
3. Data
The high resolution temperature logs were recorded by the Geophysical Institute of the University of Karlsruhe, Germany, and by the Geophysical Institute of the Czech Academy of Sciences, Prague. The temperature in Yax-1 was measured with a sampling rate of 1 or 2 s and a logging speed between 1.53 and 2.95 m min-1 in March 2002 and February 2004, whereas in May 2003 Yax-1 and another boreholes discussed in this study were logged by a step-by-step measurement with a depth increment between 1 and 5 m. The logging tools have a resolution of 1–3 mK and an absolute accuracy of several hundredths °C (Wilhelm et al2004).
Borehole Yax-1 was drilled within 77 days from December 2001 through February 2002 about 60 km south of the port of Chicxulub and 10 km north of the Ring of Cenotes. (Urrutia-Fucugauchi et al2004). The impact (suevitic) breccias were encountered within the depth interval 795–895 m overlain by Tertiary cover rocks, mostly calcarenite and calcareous siltstones, and underlain by Cretaceous target rocks, mostly megablocks of limestone, dolomite, anhydrite and impact breccias (Dressler et al2004). The uppermost 400 m section of the hole discussed in this paper was drilled by rotary drilling operations without core recovery. Its diameter was 312 mm and it was cased with 245 mm steel tubes, and cemented to fix the tube to the borehole walls (Wohlgemuth et al2004).
Seven temperature logs were obtained to the depth of 858 m from 6 March to 19 March 2002, starting 10 days after the hole was shut in and mud circulation ceased. The successive logs revealed only small temperature variations in time and space, indicating a fast temperature recovery to almost undisturbed conditions prior to the first log. A mean temperature gradient of 37 mK m-1 was determined below the uppermost 150 m (Wilhelm et al2004). While the temperature increased linearly with depth in the section 150–380 m with a constant gradient of about 41 mK m-1 (figure 2), the temperature–depth profile above 150 m displayed strong variations, which were interpreted as a manifestation of groundwater convection within karstic rocks (Wilhelm et al2004). A prominent feature of the profile was a high gradient boundary zone (about 190 mK m-1) between heat convection dominated and heat conduction dominated sections of the hole, where temperature increased by 0.8 °C in the interval 140–145 m (figure 2).
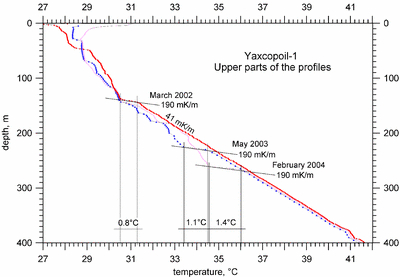
Upper 400 m sections of the three temperature logs of borehole Yaxcopoil-1 measured within 24 months after the hole shut-in. The high gradient zone is marked in each log by a line of slope 190 mK m-1 and by the value of its amplitude.
Consecutive logs were obtained 15 (May 2003) and 24 (February 2004) months after the hole shut-in to the depth of 895 m and 1398 m, respectively. The individual logs are very similar to each other with only insignificant differences below 265 m (Wilhelm et al2004), but they differ substantially above this depth. The high gradient boundary zone has been moving downwards as a distinct temperature wave (figure 2). The front of this cold wave had proceeded to the depth of 230 m by the time of the second logging in May 2003 (the apparent propagation rate was 6 m/month) and to 265 m by the time of the third logging in February 2004 (with a propagation rate of 4 m/month). The amplitude of the relative cooling within the 5–8 m thick high gradient boundary zone increased from 0.8 °C at the first logging through 1.1 °C at the second to 1.4 °C at the third logging, whereas the gradient value itself within the zone remained the same, i.e. ∼180–200 mK m-1. At the depths through which the cold wave had already passed, the temperature recovered, but stayed generally below the initial linear curve observed at the first log (figure 2).
Of eight UNAM boreholes drilled in the mid 1990s, four still existed and were logged in 2003, namely boreholes UNAM 2, 5, 7 and 8 (figure 3). UNAM 2 is located within the Ring of Cenotes, about 40 km to the SE from the Yax-1 site. It was drilled to a depth of 559 m and logged to 344 m. The temperature gradient is very small, less than 10 mK m-1 in the uppermost 200 m and close to zero below the depth of 200 m. There are even two zones of negative gradient in the depth intervals 215–255 m and 282–296 m. A much higher temperature gradient is observed in borehole UNAM-5, located 25 km south of the Ring of Cenotes and 45 km south of Yax-1. It sampled the impact breccias at the depth of 332 m. Although its original depth was 503 m, the temperature could only be measured to the depth of 334 m. The temperature gradient generally increases with depth and reaches a value of 41.7 mK m-1 in the lowermost 50 m (286–334 m). Borehole UNAM 7 is located about 50 km south of the Ring of Cenotes. Its original depth was 702 m and the impact breccias were encountered at the depth of 222 m. The borehole is blocked at 67 m. A very low temperature gradient of 1.5 mK m-1 observed throughout the measured depth interval increases to 150 mK m-1 in the lowermost 2 m. The last logged UNAM borehole is UNAM 8 situated in the Ring of Cenotes. It was open for the temperature probe to 99 m, within 2 m of the original depth of 101 m. The log displays a negative temperature gradient from the water level at 13.5 m down to 45 m. Below 60 m, the temperature increases with depth at the rate of 18 mK m-1.
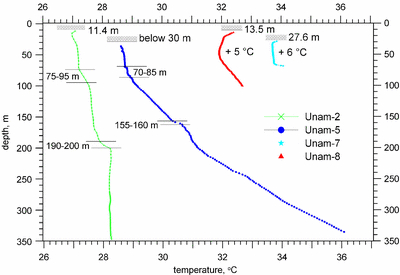
Temperature logs of boreholes UNAM 2, 5, 7 and 8 measured in May 2003. The logs UNAM 7 and 8 are shifted by +6 °C and +5 °C, respectively. The horizontal lines with hatching and depth labels indicate the groundwater levels in the individual boreholes. The horizontal lines with depth labels mark zones of increased gradient correlated with the fresh/saline groundwater interface in the UNAM 2 and 5 logs.
Temperature logs measured in three shallow hydrogeological observational boreholes 1A, 1B and 1C located along the profile Motul–Telchac Puerto perpendicular to the northern Yucatán coast (figure 1) are shown in figure 4. The distance of 1A, 1B and 1C from the coast is 4.4 km, 12.5 km and 19 km, respectively. Their depth ranges between 35 m and 45 m. They were drilled within the project of the groundwater management of the aquifer adjacent to the northern coast of Yucatán (Hita et al2003) before November 2002. Logs 1B and 1C display a 2 m thick zone of increased temperature gradient starting at the depths of 17 m and 22 m, respectively.
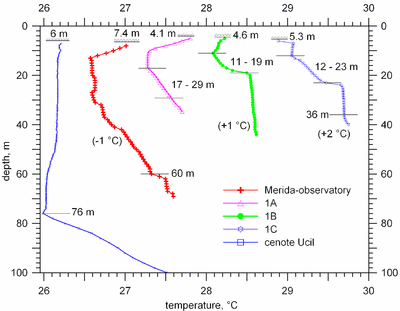
Temperature logs of the boreholes Mérida-observatory, 1A, 1B and 1C and of the cenote Ucil. The logs Mérida-observatory, 1B and 1C are shifted by -1 °C, +1 °C and +2 °C, respectively. The horizontal lines with hatching and depth labels indicate the groundwater levels in the individual boreholes. The horizontal lines with depth labels mark the fresh/saline groundwater transition zone based on the logs of the groundwater electric conductivity. For details see the text.
The last borehole measured in Yucatán during our 2003 logging campaign was a borehole on the grounds of the meteorological observatory in Mérida (figure 1). Its temperature log reaches the depth of 69 m (figure 4). It displays large variations of the temperature gradient with depth. The most conspicuous feature is a 2 m thick high gradient zone at the depth of 60 m.
In May 2003, the temperature was logged also in one of the deep cenotes, namely the cenote Ucil (figure 1), located some 25 km east of the Ring of Cenotes and 50 km inland from the coast. The observed temperature–depth curve (figure 4) has a distinct shape with a minimum at the depth of 76 m below the ground surface, assuming that the surface of the semi-open cenote lake was 6 m below the ground. The slightly negative temperature gradient between the lake surface and the depth of 76 m very sharply turns into a positive one of about 60 mK m-1 in the lowermost 24 m.
4. Discussion
In view of the existence of the karst environment extending to a depth of several hundreds of metres throughout the region, the common occurrence of convective features in most of the observed temperature logs is understandable. Quite surprising, however, are the temporal changes observed in the repeated logs in Yax-1. The downward propagation of the cold wave with a velocity of 4–6 m/month over the period of 24 months from 145 m to 265 m very probably is not a manifestation of a natural convective regime of the groundwater system. Because of its persistence and its relatively large scale, the observed downward migrating temperature anomaly can hardly be generated by a source confined to the borehole itself. We assume that its source must encompass the broader surroundings of the hole, at least tens of metres around the borehole, to be able to cool down the rock matrix.
Taking into account the geological setting, hydrogeology and the course of drilling, we see two possible driving forces of the convective features in the observed Yax-1 temperature profiles, both of them connected with the density imbalance, namely (i) groundwater circulation produced by disturbing the equilibrium fresh/saline water interface or (ii) fluid flow of denser-than-groundwater drilling mud, which accumulated in borehole surrounding voids, mostly during the drilling of the uppermost 100 m of the hole, when mud losses were huge.
Probably both these forces are effective in the studied borehole. However, the main feature of the observations, namely the migration of the cold wave from 145 m down to 265 m disqualifies the fresh/saline water imbalance as a driving force. Their equilibrium interface is quite shallow, 50–80 m below the surface and one can imagine either a downward migration of saline water between the surface and the interface or an upward migration of fresh water from depths below the interface. But an upward fluid flow would disturb the temperature profile in a different way than that observed. The persistent downward migration of the cold wave requires a steady downward flow of a fluid denser than saline groundwater through rocks adjacent to the borehole. The drilling mud is the only conceivable fluid that meets this requirement in the studied case.
A comparison of the temperature logs from boreholes UNAM 2 and UNAM 5 (figure 3) demonstrates the strong influence of the groundwater circulation within the highly permeable karst of the Ring of Cenotes on the subsurface temperatures. The UNAM-2 borehole is located within the Ring of Cenotes and its temperature profile bears the signature of an intensive downward groundwater flow with a strongly reduced temperature gradient down to the bottom of the log at the depth of 344 m. The two zones of negative gradient may indicate water convection in a cavity or a flow of groundwater of a different temperature in a conduit close to the borehole. Signs of the convective heat transfer are much less pronounced in the borehole UNAM-5, located 25 km south of the Ring in the Sierrita Ticul Mountains. The gradual increase of the temperature gradient with depth may indicate a groundwater circulation pattern typical for a recharge area enhanced by a 40–50 m terrain elevation over the flat platform to the north. In spite of that, the gradient of 41.7 mK m-1 observed in the lowermost 50 m, which are immediately underlain by the impact breccias, is slightly higher than the gradient observed in Yax-1 in the same stratigraphic position just above the depth of 800 m (Wilhelm et al2004). If the same stratigraphic position incurs similar values of the thermal conductivity of rocks, the conductive component of the heat flow should be expected to be even higher than in Yax-1, i.e. over 70 mW m-2.
The UNAM 2 and 5 logs might bear also a signature of the fresh/saline water interface (halocline), which is usually marked by a zone of increased temperature gradient (Stoessell et al2002, Stoessell 2003). This zone seems to be located at the depth of 70–90 m in both holes (figure 3), but the UNAM 2 and 5 logs reveal an even sharper temperature increase at the depths of 190–200 m and 155–160 m, respectively. The halocline depth of 120 m based on the electrical soundings was reported by Steinich and Marín (1996) for the borehole distance of 90 km from the coast, but the UNAM 2 and 5 are located outside the area of the soundings and it cannot be excluded that at their sites the halocline is deeper than 120 m. The situation is not also clear in Yax-1, where a sharp temperature increase zone observed between 40 and 50 m during the first logging in March 2002 turned into a near-zero gradient zone in the two consecutive logs and the increased gradient zone appeared in the depth range of 70–80 m. According to Steinich and Marín (1996) the halocline should be there between 50 and 80 m. As mentioned above, the natural groundwater conditions in Yax-1 were strongly disturbed during the drilling, which could explain the observed time changes.
It turned out that boreholes UNAM 7 and 8 are located within several tens of metres of the local water supply wells and the probability of a distortion of the subsurface temperature field within the borehole depth range through groundwater pumping is high.
A more substantiated correlation between the position of the halocline and the shape of the temperature log could be established in the case of four shallow boreholes 1A, 1B, 1C and Mérida-observatory and of the cenote Ucil (figure 4). All five temperature–depth profiles can be compared with the corresponding groundwater electric conductivity–depth profiles (Hita et al2003, Stoessell 2003). According to these conductivity logs, a well-defined narrow halocline occurs in the boreholes 1A, 1B and 1C and in the cenote Ucil, where the electric conductivity increases within about the 10 m depth interval from a value below the limit for fresh water (0.22 S m-1) to values appreciably above this limit. The upper and lower boundaries of the halocline are at the depths of 17 m and 29 m for borehole 1A, at the depths of 11 m and 19 m for borehole 1B and at the depths of 12 m and 23 m for borehole 1C. Conductivity in the cenote Ucil starts to grow at the depth of 76 m and approaches 4.0 S m-1, typical of sea water, at the bottom. The boundaries are shown in figure 4. The upper halocline boundary coincides with a sharp increase in the temperature gradient. Above the halocline, the near-zero temperature gradient within the fresh water is probably sustained by convection in highly permeable karstic rocks, or in the water column in the case of the cenote Ucil, and might be changing slightly due to the annual run of the ground surface temperature. The lower halocline boundary is clearly reflected on the 1B and 1C temperature logs by a sharp decrease of the gradient indicating convection also in the saline groundwater, whereas no gradient change occurs on the 1A log. The only distinct feature of the 1A conductivity profile compared to those of 1B and 1C is a much higher conductivity value, and therefore a higher groundwater density, just below the lower halocline boundary. The electric conductivity in borehole 1C stabilizes below the halocline at a value of 0.4 S m-1 only and starts to grow again just above the bottom at the depth of 36 m. It is also reflected in the temperature log by the gradient increase.
The electric conductivity in the borehole Mérida-observatory increases with changing rate with depth from the groundwater level at 7 m and stabilizes at a value of 1.5 S m-1 below the only evident step at the depth of 61–63 m, which can be correlated with a zone of increased temperature gradient between 60 and 62 m. There are at least three other smaller temperature steps, which may be connected with less-pronounced salinity/density barriers.
5. Conclusions
An analysis of the available data shows that the subsurface temperature field in the karst of the north-western Yucatán is dominated to the depth of several hundred metres by convective heat transport. The temperature gradient just below the unsaturated layer is close to zero and increases sharply within the halocline layer, where the lighter fresh groundwater mixes with the underlying heavier saline groundwater. This density barrier stops the sinking fresh water as well as the ascending saline water and interrupts the convection. As the logs of the groundwater electric conductivity indicate, the salinity increases in some cases step-wise and there exist several layers of different density, which break up convection into several convective cells. It is evident that this basic mechanism is strongly influenced by the local system of interconnected caverns, conduits and all other forms of secondary porosity in the karstic rocks.
One of the most remarkable effects is the downward migrating temperature wave which cooled the section between 145 and 265 m of the borehole Yax-1 by about 1 °C within 23 months between the first and last temperature loggings and disturbed the originally linear log, which indicated purely conductive heat transfer. The observed phenomenon contradicts the common experience of fading out the temperature drilling disturbance after the shut-in of the hole. The most probable cause is the drilling mud, which accumulated in a large volume around the hole in the uppermost 100 m in the karstic dissolution voids, seeps slowly down and cools the rock matrix.
The lowermost 50 m section of the UNAM 5 temperature log suggests a heat flow value at least as high as that in the borehole Yax-1, i.e. 70 mW m-2 or more, on the rim of the Chicxulub crater, which contradicts the conclusions of Matsui et al (1998), who predicted a heat flow of 60 mW m-2 at the centre of the crater decreasing towards its rims to 20 mW m-2. It turns out that it is very difficult to determine the deep conductive heat flow at the base of the karst formation, which is representative for the crustal and lithospheric thermal studies, from 50 to 100 m deep boreholes in the study area. The zone where the convection dominance and its depth extent are extraordinary large, is the Ring of Cenotes. As demonstrated by the UNAM 2 temperature log, the temperature gradient in the Ring can effectively be zero as deep as 340 m.
Acknowledgments
The research was supported by the Grant Agency of the Czech Republic as project 205/03/0997 and the Ministry of Education project LE150 and by the Deutsche Forschungsgemeinschaft within the frame of the ICDP Priority Programme through contracts WI 687/17-1,2,3. We are grateful to Professor Jaime Urrutia-Fucugauchi, Dr Luis Marín and Dr Eduardo Graniel Castro for their valuable professional and logistic assistance provided during our logging campaigns in Mexico. We are indebted to two anonymous reviewers for comments which helped us to improve this communication.