-
PDF
- Split View
-
Views
-
Cite
Cite
Cruz Garcerá, Yuling Ouyang, Sara J. Scott, Enrique Moltó, Elizabeth E. Grafton-Cardwell, Effects of Spirotetramat on Aonidiella aurantii (Homoptera: Diaspididae) and Its Parasitoid, Aphytis melinus (Hymenoptera: Aphelinidae), Journal of Economic Entomology, Volume 106, Issue 5, 1 October 2013, Pages 2126–2134, https://doi.org/10.1603/EC12510
- Share Icon Share
Abstract
Laboratory and field studies were conducted to measure the effects of spirotetramat on life stages of California red scale, Aonidiella aurantii (Maskell), and a primary parasitoid, Aphytis melinus DeBach. Organophosphate-resistant and -susceptible populations responded similarly to spirotetramat, suggesting there is no cross-resistance between these insecticide classes. First and second instar male and female A. aurantii were 10- and 32-fold more susceptible to spirotetramat (LC50 = 0.1–0.2 ppm) compared with early third (LC50 = 1.5 ppm) and late third instar females (LC50 = 5.3 ppm). The LC99 value indicated that late stage third instar females would not be fully controlled by a field rate of spirotetramat; however, spirotetramat would reduce their fecundity by 89%. Field applications of spirotetramat in two water volumes and using two adjuvants (oil and a nonionic spray adjuvant) showed similar reduction in A. aurantii numbers, even though the higher water volume demonstrated more complete coverage. These data suggest that this foliarly applied systemic insecticide can be applied in as little as 2,340 liters/ha of water volume, minimizing application costs, and that the two adjuvants acted similarly. The endoparasitoid, A. melinus, was unaffected by the field rate of spirotetramat when it was applied to the host when the parasitoid was in the egg or larval stage. Adult A. melinus showed 2 wk of moderate reductions in survival when exposed to leaves with field-weathered residues. Spirotetramat is an integrated pest management compatible insecticide, effective in reducing A. aurantii stages and allowing survival of its primary parasitoid A. melinus.
California red scale, Aonidiella aurantii (Maskell), is a worldwide pest of citrus, and it has been a key pest of California citrus for many years, especially in the San Joaquin Valley (Ebeling 1959, Flint et al. 1991, Grafton–Cardwell et al. 2008, Grafton–Cardwell 2010). California red scale is found on leaves, twigs, and fruit, on all varieties of citrus. It causes primarily cosmetic damage to the fruit, resulting in the downgrading of the fruit in the packing house. However, in severe infestations, leaf yellowing and dieback of branches occur, reducing the productivity and health of the tree and occasionally causing tree death (Jeppson 1989, Flint et al. 1991). Biological control has not always been sufficient to keep armored scales below an economic threshold in the San Joaquin Valley, because of extreme winter and summer temperatures. Cold winter temperatures eliminate younger instars, synchronizing the first and second generations of scale in the spring. This synchrony minimizes overlap of scale age classes, and consequently, there are periods during the early season when second and third instar scale are not available, and these stages are preferred by parasitoids for oviposition (Walde et al. 1989, Forster et al. 1995). This causes a delay in the development of parasitoid populations until the third generation of scale. In addition, extremely high temperatures in summer are detrimental to parasitoid development because of reduced host size (Yu and Luck 1988, Pekas et al. 2010). A delicate biological control system, coupled with an emphasis on cosmetically perfect fresh market fruit, results in a pest management challenge that often requires insecticides.
California red scale has demonstrated its ability to develop resistance to many of the insecticides used to control it. In the late 1800s, control of these scales began with the use of hydrogen cyanide (HCN), but then, as early as 1912, California red scale resistance to HCN was detected (Quayle 1938). Petroleum oil sprays then replaced HCN as control agents. With the development of parathion in the 1940s, citrus growers next relied on organophosphate and later carbamate insecticides for scale insect control (Carman 1977). Resistance to organophosphate and carbamate insecticides in California red scale populations developed in the 1970s in South Africa (Georgala 1975, Nel et al. 1979) and Australia (Abdelrahman 1973) and in the 1990s in California (Grafton–Cardwell and Vehrs 1995, Grafton–Cardwell et al. 2001).
The insect growth regulators (IGRs) buprofezin and pyriproxyfen were registered for California red scale control in California in the late 1990s. Pyriproxyfen accounted for 90% of the IGR applications in the San Joaquin Valley during 2005–2010 because of lower cost and greater efficacy against California red scale (California Department of Pesticide Regulation [CDPR] 2000–2010, Grafton–Cardwell et al. 2006). Pyriproxyfen and buprofezin have little toxic effect on the primary parasitoid of California red scale, Aphytis melinus DeBach (Rill et al. 2008), allowing them to assist with control of scale. Although pyriproxyfen has been the primary California red scale treatment for more than a decade, resistance monitoring has not revealed significant levels of resistance to pyriproxyfen (Ouyang and Grafton–Cardwell 2010). However, given the documented ability of California red scale to develop resistance, it is important to introduce insecticides with alternative modes of action into the treatment regime.
Spirotetramat, a foliarly systemic tetramic acid insecticide, providing a novel mode of action with activity against sucking insect pests such as whiteflies, psyllids, and aphids was registered for California citrus in 2008 (Brück et al. 2009, Frank and Lebude 2011, Jamieson et al. 2010, Page–Weir et al. 2011). The unique two-way systemic action of spirotetramat, moving throughout the plant via the phloem and xylem (Brück et al. 2009), potentially allows it to be applied at lower water volumes compared with other foliar applications that require a minimum of 7,015 liters/ha, and so could greatly reduce application costs for growers. This product requires application with an adjuvant to enhance its wettability and increase its penetration, improving its efficacy (Brück et al. 2009). The objectives of our research were to determine the response of various stages of California red scale to spirotetramat, measure its impact on eggs, larvae, and adult stages of the parasitoid A. melinus, and determine the effect of two water volumes (2,340 and 4,680 liters/ha) and two surfactants (oil and a nonionic spray adjuvant) on both insecticide distribution and field efficacy. The results will provide a measure of the efficacy and integrated pest management (IPM) compatibility of spirotetramat and a baseline for future resistance monitoring.
Materials and Methods
Two laboratory colonies of California red scale, one organophosphate-resistant and one susceptible, were used in these experiments. The susceptible colony of California red scale originated from an insecticide-free backyard citrus tree on 15 September 1991 in Porterville, CA. The organophosphate-resistant colony originated from a navel orchard, Citrus sinensis (L.), in Orosi, CA, collected during 1998. Both colonies have been maintained continuously without pesticide selection on green lemons, Citrus limon (L.) at the University of California, Kearney Agricultural Center (Parlier, CA), at 25.5 ± 2°C, 45 ± 10% relative humidity (RH), and a photoperiod of 12:12 (L:D) h (Grafton–Cardwell et al. 1998). The organophosphate insecticide resistance and susceptibility of the two colonies was confirmed by measuring the esterase activity of 20 individuals from each colony at the time of the spirotetramat bioassays (Grafton–Cardwell et al. 2004). The esterase activity of individuals of the susceptible lab colony ranged from 0.0 to 0.1 nm α-naphthyl esterase/min/μg protein while the organophosphate-resistant colony individuals ranged from 0.6 to1.7 nm α-naphthyl esterase/min/μg protein. Green lemons used for rearing were dipped in liquid paraffin wax so that 75% of their surface was coated, to conserve moisture and prevent shrinkage, and the remaining 25% was left wax-free to allow scale to settle. Waxed green lemons were infested by placing them on top of infested colony lemons with crawler-producing scales.
Response of California Red Scale Stages to Spirotetramat.
A fruit dip bioassay method (Grafton–Cardwell and Vehrs 1995) was used to determine the response of first instar California red scale to spirotetramat for both susceptible and organophosphate-resistant colonies. Green lemons were infested with scales for 24 h at 25 ± 2°C and 50–60% RH. Ten to 25 healthy first instar scales (white cap stage) on each lemon were circled with a permanent marker. Six lemons were used for each insecticide concentration. Scale-infested lemons were dipped for 10 s in concentrations of 0, 0.01, 0.0316, 0.1, 0.316, and 1.0 ppm spirotetramat (Movento 240 SC, 240 g active ingredient per liter, Bayer CropScience LP, Research Triangle Park, NC) mixed with 0.016% Triton B-1956 (Sigma–Aldrich Corp., St. Louis, MO). Fourteen days after treatment, when scales had matured to the second instar, the scale covers were removed and mortality was assessed.
The responses of male and female second instar scales from the susceptible colony were tested with spirotetramat using the fruit dip method described above. Green lemons were infested with California red scale for 24 h and the scales allowed to develop at 25 ± 2°C into second instars, at a point just before the sex can be identified by the presence of eye color in males (≈16 d). Twenty-five to fifty second instar scales on each lemon were circled with a permanent marker, and five lemons were used for each concentration of insecticide. Scale-infested lemons were treated with 0, 0.01, 0.0316, 0.1, 0.316, 1, and 3.16 ppm spirotetramat mixed with 0.016% Triton B-1956. The numbers of live and dead males and females were recorded 2 wk after the treatment when scales in the control treatment had matured to adult stages. Scales were considered dead if they did not mature to the next stage.
The responses of third instar female scales of two ages from the susceptible colony were tested with spirotetramat using the fruit dip method described above. Green lemons were infested with California red scale for 24 h 4 d apart and then allowed to develop into early third instar females (26 d after infestation) and late third instar females (30 d after infestation). The two age-groups were treated at the same time. The concentrations of spirotetramat used for testing were 0, 0.1, 0.316, 1, 3.16, 10, 31.6, and 100 ppm spirotetramat mixed with 0.016% Triton B-1956. Five lemon replicates were used for each concentration. The number of live adult females and dead females were recorded 20 d after treatment. All scales were inverted, and if crawlers were found under the scale body, the scale was counted as alive.
Concentration–mortality response data (response slope, LC10, LC50, LC90, and LC99) were obtained for both organophosphate-resistant and -susceptible first instar scale, second instar male and female, and early and late-stage third instar female scale by performing probit analysis (LeOra Software 2005).
Effects of Spirotetramat on the Fecundity of California Red Scale.
Twelve green lemons were infested with first instar scales from the susceptible scale colony as described above. The scales were allowed to mature until they had mated and were close to producing crawlers, ≈35–40 d after infestation at 25 ± 2°C. Ten gravid female scales on each fruit were circled, and the remaining scales were removed. Six fruits were dipped for 10 s into 75 ppm spirotetramat (the field rate) mixed with 0.016% Triton B-1956. Six fruits were dipped into water mixed with 0.016% Triton B-1956 to act as the control. The crawlers began to emerge 9 d after the treatment. The number of first instar crawlers and white caps were recorded and then removed from the green lemon three times per week for 25 d. The total number of crawlers per fruit for treated and untreated lemons was transformed Log10(x + 1) before analysis, and the means were compared with a t-test (P < 0.05) (Statgraphics Centurion XV 2007).
Influence of Water Volume and Surfactant Type on Field Efficacy and Coverage.
A field trial was conducted during June–October 2009 on 41-yr-old navel orange trees (C. sinensis L.) variety ‘Atwood Washington,’ in a commercial orchard in Woodlake, CA (36° 25′12.7″ N/119° 9′10.16″ W). The trees were planted in a 6.8- by 6-m row and tree spacing. Tree height averaged 4.55 m, and the tree diameter averaged 5.1 m, resulting in an average tree volume of 118.3 m3, assuming the crown has a cube shape.
The efficacy of three treatments with 730 ml/ha of formulated spirotetramat (Movento 240 SC), as recommended on its label, mixed with one of two adjuvants and applied at two water volumes (2,340–4,680 liters/ha), was compared with an untreated control. Treatments were as follows: 1) 1% of Britz 415 Supreme Spray Oil (Britz Fertilizers, Inc., Five Points, CA) in 2,340 liters/ha; 2) 1% of Britz 415 Supreme Spray Oil in 4,680 liters/ha; 3) 2.34 liters/ha of Exit (Miller Chemical & Fertilizer Corporation, Hanover, PA) in 2,340 liters/ha; and 4) nontreated trees. Exit is a nonionic adjuvant activator–enhancer designed for water- and oil-soluble agrochemicals to increase their deposition capability and surface activity. Britz 415 Supreme Spray Oil is an nC21 agricultural mineral oil with 92% unsulfonated residue.
The treatments were applied with a conventional air blast sprayer (D2/40 Engine-powered sprayer, Air-O-Fan, Reedley, CA). They were performed at 1 MPa (pressure measured in the manifold) and a tractor speed of 2.85 km/h. The wind speed was measured at five points on each side of the sprayer at the level of the nozzles with an anemometer (Kestrel 3000, Nielsen–Kellerman Co., Boothwyn, PA). The average measured wind speed was 41.39 m/s, and the estimated airflow was 1,49,961 m3/h. The air blast sprayer was equipped with Tee-Jet ceramic disc-core nozzles (Spraying Systems Co., Wheaton, IL). To apply the water volume of 2,340 liters/ha, 14 discs number 4 and two discs number 5, each one with a core of two holes, were used. To apply the water volume of 4,680 liters/ha, eight discs number 6 with four-hole cores were added to this arrangement.
Treatments were applied on 22 June 2009 and were assigned to three replicated plots per treatment (five adjacent rows of 20 trees, 0.4 ha), based on pretreatment densities of California red scale. Population density was estimated by counting live first-, second-, third-instar, and adult female scales from five twigs and three leaves per tree. The samples were nonrandomly collected (twigs with evidence of California red scale were chosen) from the medium height of six trees in the center of each plot before treatment on 3 June 2009. The same scale sampling method was used to determine the efficacy of treatments on 22 July 2009. On 9 October 2009, samples were collected randomly from the medium height of the trees and on 20 October 2009, from the top of the crown of the trees.
Weather conditions during spray application (temperature, RH, and wind speed) were measured at 1-h intervals at a height of 2 m by using a portable weather station (Kestrel 3000, Nielsen–Kellerman Co.). The meteorological data collected during the treatment were temperature: 26.4 ± 1.07°C; RH: 36.49 ± 3.06%; wind speed: 0.77 ± 0.15 m/s. Spray coverage of six trees per treatment was measured as percentage spray coverage of Tee-Jet 26- by 76-mm water-sensitive paper (WSP) (Spraying Systems Co., Wheaton, IL). Spray cards were stapled to the top of one leaf at 18 locations in the tree. These locations included three heights (L: low, 1 m; M: medium, 2 m; H: high, 3.5 m), two depths (O: outside; I: inside), and three random leaves at each combination “height × depth”. Spray cards were stapled just before the application of the treatments, collected as soon as the treatment had dried, and kept in dry conditions in plastic bags until their analysis. Sprayed cards were photographed and images analyzed with specific software (Matrox Inspector, version 2.2, MatroxTM, Dorval, Canada) following the methodology described by Chueca et al. (2010). The images were taken with 20 pixels/mm resolution. Objects in the image that consisted of one single pixel were considered to be noise and removed. Therefore, impacts <50 μm diameter were not necessarily detected. In each image, the program detected all impacts (deposited droplets produced by the spray over the collector) >2.5 10−3/mm2, and then calculated percent coverage as the percentage of the total surface covered by the impacts.
Both efficacy and coverage of each combination of “height × depth” of the treatments were compared using a one-way analysis of variance (ANOVA) and means separated using the least significant difference (LSD) test (P < 0.05). The ANOVA assumption of normal distribution of data was assessed by the Shapiro–Wilk's test (Shapiro and Wilk 1965), and the assumption of homocedasticity by the Levene's test (Levene 1960) (Statgraphics Centurion XV 2007).
Effects of Spirotetramat on Immature Stages of A. melinus.
Adults of A. melinus were obtained from Mulholland Insectary (Orange Cove, CA) and allowed to host feed for 1 d on mixed California red scale stages before bioassays were conducted. Twelve green lemons infested with third instar California red scale (30 d after infestation) were exposed to 100 adults of A. melinus for 4 h. Twenty-four hours after exposure to the adult parasitoids, when the parasitized hosts contained eggs, six fruit were dipped for 10 s in 75 ppm spirotetramat and six fruit dipped in water, both mixed with 0.016% Triton B-1956. Fruit was kept in cylindrical paper containers (9 cm in diameter by 9 cm in height) with a 5-cm-diameter cloth circle at the top of the lid to allow air flow (25 ± 2°C). Ten days after exposure to A. melinus, half of the scales on the green lemons were examined and the number of unparasitized live and dead scales and the number of parasitized scales containing A. melinus pupae were recorded. The second half of the scales on each fruit was measured in a similar manner 15 d after exposure to A. melinus when the parasitoids in the untreated control treatment had emerged as adults.
To measure the impact of spirotetramat on A. melinus larvae, the same methodology was used as in the egg study, except that the fruit was dipped in the insecticide or water solution 5 d after exposure to A. melinus adults, when the parasitized hosts contained larvae. The total number of healthy and dead scales, and the number of live A. melinus at the pupal and adult stages were recorded 10 and 15 d after exposure to A. melinus adults (5 and 10 d after treatments were applied).
A t-test (P < 0.05) was used to compare the percentages of unparasitized live and dead scale and the percentage parasitized at the pupal and adult A. melinus stages between the treatments after arcsine (x)1/2 transformation of the proportions (Statgraphics Centurion XV 2007).
Effects of Spirotetramat on A. melinus Adults.
The response of A. melinus adults to various insecticides including spirotetramat was assessed using citrus twigs collected from 10-yr-old ‘Washington’ navel orange trees, C. sinensis (L.) Osbeck, in a 20 by 20 row and tree spacing, from a field trial conducted at the Lindcove Research and Extension Center (Exeter, CA). The trees were treated with spirotetramat (730 ml/ha Movento 240 SC), imidacloprid (1,025 ml/ha Admire Pro, 550 g a.i./liter, Bayer CropScience), chlorpyrifos (14 liters/ha Lorsban Advanced, 450 g a.i./liter, Dow AgroSciences LLC, Indianapolis IN), pyriproxyfen (1,170 ml/ha Esteem 0.86 EC, 103 g a.i./liter, Valent U.S.A. Corporation Agricultural Products, Walnut Creek, CA) or left untreated. Treatments were applied to eight individual tree replications on 11–12 July 2012 in 7,015 liters/ha for chlorpyrifos and pyriproxyfen and in 2,342 liters/ha for spirotetramat, by means of a handgun sprayer (John Bean Sprayers, LaGrange, GA) at 3.1 MPa. The imidacloprid was applied over a 2-h period through the micro-irrigation system (Fan Jet wrap-around trajectory heads, 30 psi) after prewetting for 1 h.
Individual twigs, ≈10 inches long that contained 5–10 leaves, from each of six trees per treatment were cut and placed in 3.75-liter glass jars. Adult A. melinus individuals were treated with CO2 for 90 s to immobilize and count them. Sixty A. melinus wasps were placed in each jar, which was covered with polyester fabric to allow air circulation. There were six replicates for each treatment. The test was conducted at a room temperature (25 ± 2°C). Twenty-four hours after exposure of the leaves and twigs to the adult A. melinus individuals, mortality was assessed. This process was repeated each week for 12 wk after the treatments were applied. The posttreatment percentage mortality was analyzed for each week using one-way ANOVA, and the means were separated by the LSD test (P < 0.05) after arcsine (x)1/2 transformation of the proportions (Statgraphics Centurion XV 2007).
Results
Response of California Red Scale Stages to Spirotetramat.
Organophosphate-resistant and -susceptible first instar scales responded similarly to spirotetramat based on overlap of the CIs for the LC10, LC50, LC90, and LC99 (Table 1). These data suggest that there is no cross-resistance between organophosphate insecticides and spirotetramat for California red scale. The average LC50 for the two colonies was 0.124 ppm for first instar scales.
Concentration–mortality response of organophosphate-resistant and -susceptible first instar California red scale to spirotetramat
![]() |
![]() |
Concentration–mortality response of organophosphate-resistant and -susceptible first instar California red scale to spirotetramat
![]() |
![]() |
When California red scale was treated with spirotetramat in the early second instar stage, before the males could be differentiated from the females, the resulting males and females showed no differences in response, based on overlap of the confidence intervals for the LC50, LC90, and LC99 (Table 2). The average LC50 for second instar males and females was 0.165 ppm, which was less than twofold higher than the LC50 for the first instar scales, indicating that second instar scales have only slightly less sensitivity to spirotetramat.
Concentration–mortality response of second instar male and female California red scale to spirotetramat
![]() |
![]() |
Concentration–mortality response of second instar male and female California red scale to spirotetramat
![]() |
![]() |
The early third instar females showed 3.5-fold greater sensitivity to spirotetramat than the 4 d older third instar females (Table 3). The LC50 was 1.48 ppm for early third instar female scales and 5.29 ppm for the late third instar female scales. Early and late third instar scales were ≈10-fold and 32-fold less sensitive to spirotetramat compared with first instar and second scales. Thus, scale sensitivity to spirotetramat declines significantly as the scales age from second instar to third instar and as the third instar female scales mature.
Concentration–mortality response of early and late-stage third instar female California red scale to spirotetramat
![]() |
![]() |
Concentration–mortality response of early and late-stage third instar female California red scale to spirotetramat
![]() |
![]() |
Treating late third instar female scales with spirotetramat effectively reduced scale fecundity. Over the 25-d measurement period, the mean number of crawlers produced by water-treated females was 49.35 ± 9.74, while the spirotetramat-treated females produced significantly fewer crawlers, with a mean of 5.48 ± 1.74 crawlers (t = 6.691; P < 0.0001).
Influence of Water Volume and Surfactant Type on Field Efficacy and Coverage.
The water-sensitive papers demonstrated that percentage oil coverage was greatest on the low to medium heights of the tree (Fig. 1), with coverage ranging from 66 to 88% of card area. Smallest differences in coverage were found on the outside of the tree at the medium height (F = 0.53; df = 2, 42; P = 0.5936), and the low height (F = 3.67; df = 3, 46; P = 0.0335). In contrast, the top of the tree outside coverage averaged only 30–34% of card surface area, and the 4,680 liters/ha treatment provided significantly greater coverage than either of the two 2,340 liters/ha treatments (F = 7.16; df = 2, 46; P = 0.002). Inside the tree, the 4,680 liters/ha treatment resulted in greater coverage than the 2,340 + oil treatment at the top of the tree (F = 11.19; df = 2, 53; P = 0.0001) and the 2,340 + Exit treatment at all heights (medium height F = 6.31, df = 2, 47, P = 0.0038; and low height F = 7.26, df = 2, 48, P = 0.0018).
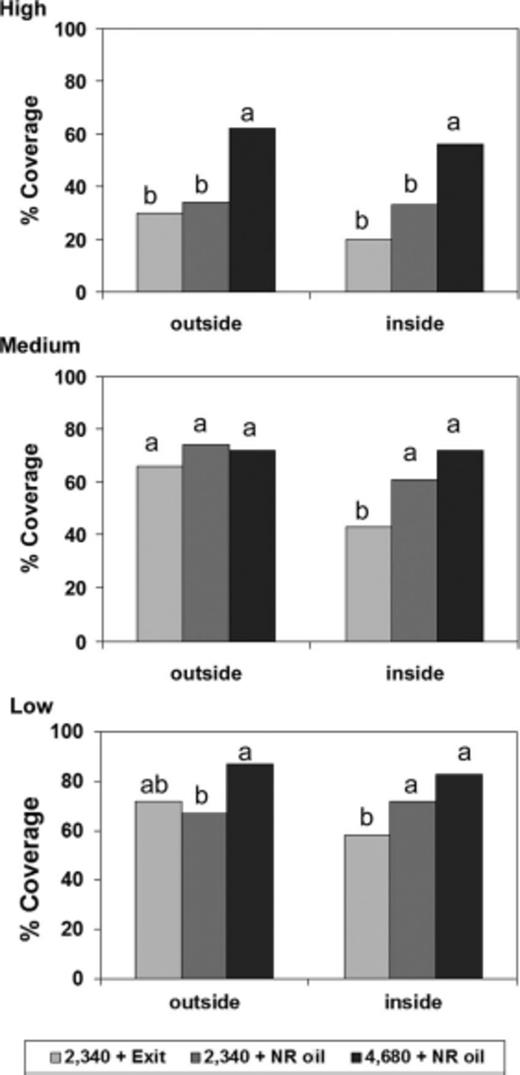
Water-sensitive paper coverage per treatment (mean ± SE) at two depths of the canopy (outside and inside) and at three heights High: 3.5 m, Medium: 2 m, and Low: 1 m. Mean values followed by a different letter for each position are significantly different (LSD test, P < 0.05).
Although greater coverage was achieved with the 4,680 liters/ha treatment, scale densities were not significantly different among water volumes or adjuvant treatments in July or in October in the middle of the tree and the top of the tree (Table 4). These data suggest that water volume could be reduced as low as 2,340 liters/ha when treating with spirotetramat and the two adjuvants were similar in their effectiveness.
Number of live scales per leaf and twig (mean ± SE) before and after treatments of 730 ml/ha of formulated spirotetramat applied in two volumes of water and using two different adjuvants
![]() |
![]() |
Number of live scales per leaf and twig (mean ± SE) before and after treatments of 730 ml/ha of formulated spirotetramat applied in two volumes of water and using two different adjuvants
![]() |
![]() |
Effects of Spirotetramat on A. melinus.
Spirotetramat applied at a rate of 75 ppm had no negative effect on the egg stage of A. melinus (Table 5). Nine days after treatment, when the scales were dissected, the spirotetramat treatment had caused 57% mortality of the nonparasitized third instar scales compared with 11% mortality of scales in the water treatment, and these differences were statistically significant (P < 0.001). However, there were no statistical differences (P = 0.682) in the percentage of scales with parasitoid pupae in the water-treated (45.6%) and spirotetramat-treated lemons (41.8%). The results were similar at 14 d after treatment, with the spirotetramat causing 62% mortality of unparasitized scales compared with 12% in the water treatment (P < 0.001), while the percentage of scales with emerged parasitoids was not significantly different (P = 0.120). Similarly, when scales were treated at the larval stage of A. melinus (Table 6), the percentage of unparasitized scales that were dead was significantly higher (P = 0.008) for the spirotetramat treatment (39%) compared with the water treated control (15%), but the percentages of parasitized scales were the same (47%) for the two treatments (P = 0.940). Similar results were observed 9 d after treatment when the A. melinus had emerged. Thus, 75 ppm spirotetramat, a concentration approaching the field rate, caused significant mortality of the scales, but had little effect on development and emergence rate of immature A. melinus.
Residues of spirotetramat on leaves and twigs collected from a treated citrus orchard allowed 61 and 83% survival of A. melinus, during weeks 1 and 2 after treatment, and by the third week, survival was >95% (Table 7). This is a similar result to that observed with the IGR, pyriproxyfen, that resulted in 20 and 78% survival during weeks 1 and 2 and >95% survival in the subsequent weeks after treatment. This is also similar to the systemic neonicotinoid, imidacloprid, that allowed 30 and 45% survival in weeks 1 and 2 and >94% survival in the weeks following. In contrast, the broad-spectrum organophosphate insecticide, chlorpyrifos, allowed <95% survival for 11 wk. These data suggest that spirotetramat has only a short-term, moderately toxic effect on A. melinus adults.
Effect of residues of spirotetramat, imidacloprid, chlorpyrifos, and pyriproxyfen on adult A. melinus in a 24-h bioassay 1–12 wk after treatments were applied to field trees
![]() |
![]() |
Effect of residues of spirotetramat, imidacloprid, chlorpyrifos, and pyriproxyfen on adult A. melinus in a 24-h bioassay 1–12 wk after treatments were applied to field trees
![]() |
![]() |
Discussion
Younger, first and second instar scales were ≥10 fold more susceptible to spirotetramat compared with third instar female scales (Tables 1 and 2). The calculated field rate of spirotetramat, depending on the water volume used to apply it (4,680–2,340 liters/ha), ranges from 39 to 76 ppm, well above the LC99 for the younger instars but, based on our study, would allow a portion of the early- and late-stage females to survive (Table 3). The loss of sensitivity in response to spirotetramat as third instar scales aged may be because of a reduction in feeding (Forster et al. 1995), as spirotetramat must be ingested to have an effect on scales. Spirotetramat also reduced the fecundity of California red scale but did not eliminate it. Biche et al. (2011) demonstrated a high level of mortality of larvae and adults and reduced fecundity of the diaspidid scale, Lepidosaphes beckii (Newman), as well as greater impact with lower water volumes. Our field trial demonstrated that despite better coverage achieved with oil and 4,680 liters/ha water volume, all treatments provided similar levels of A. aurantii control. Thus, citrus growers can use either Exit or oil as an adjuvant and can apply as little as 2,340 liters/ha of water with spirotetramat, greatly reducing application costs.
Spirotetramat sprays had no significant negative effect on the ability of A. melinus eggs and larvae to survive to the pupal and adult stages (Tables 5 and 6). When A. melinus oviposits, it paralyzes its host, and this would prevent the scale from feeding and taking up the spirotetramat. Spirotetramat residues did cause a short-term (2 wk) low level of mortality (17–29%) of A. melinus adults (Table 7). Other parasitoids including Anagyrus sp. near pseudococci, Aphelinus certus Yasnosh, and Microplitis mediator (Haliday) have been shown to be relatively unaffected by spirotetramat (Frewin et al. 2011, Mansour et al. 2011, Moens et al. 2012), or in the case of Tamarixia radiata (Waterston), affected only by fresh residues (Hall and Nguyen 2010). Liu et al. (2012) found significant mortality when parasitoids fed on spirotetramat-treated honey-water. Differences in the effects of spirotetramat in the studies are likely because of the fact that spirotetramat has limited contact toxicity and the main effect is achieved through ingestion (Brück et al. 2009). Spirotetramat has been shown to be toxic to phytoseiid mites at field rates (Grafton–Cardwell and Scott 2008; Lefebvre et al. 2011, 2012). Phytoseiids that occasionally leaf feed or feed on prey that have ingested the toxicant would be more susceptible than parasitoids. Our data suggest that spirotetramat is compatible with A. melinus and is an important rotational insecticide with pyriproxyfen for A. aurantii control.
Acknowledgments
We thank Griffith Farms for providing a field site and Bayer CropScience, Dow AgroSciences, and Valent USA Corporation Agricultural Products for providing insecticides. This research was funded in part by the California Citrus Research Board.
References Cited