-
PDF
- Split View
-
Views
-
Cite
Cite
Elena Gimmi, Christoph Vorburger, High specificity of symbiont-conferred resistance in an aphid-parasitoid field community, Journal of Evolutionary Biology, Volume 37, Issue 2, February 2024, Pages 162–170, https://doi.org/10.1093/jeb/voad013
- Share Icon Share
Abstract
Host–parasite coevolution is mediated by genetic interactions between the antagonists and may lead to reciprocal adaptation. In the black bean aphid, Aphis fabae fabae, resistance to parasitoids can be conferred by the heritable bacterial endosymbiont Hamiltonella defensa. H. defensa has been shown to be variably protective against different parasitoid species, and different genotypes of the black bean aphid’s main parasitoid Lysiphlebus fabarum. However, these results were obtained using haphazard combinations of laboratory-reared insect lines with different origins, making it unclear how representative they are of natural, locally (co)adapted communities. We therefore comprehensively sampled the parasitoids of a natural A. f. fabae population and measured the ability of the five most abundant species to parasitize aphids carrying the locally prevalent H. defensa haplotypes. H. defensa provided resistance only against the dominant parasitoid L. fabarum (70% of all parasitoids), but not against less abundant parasitoids, and resistance to L. fabarum acted in a genotype-specific manner (G × G interactions between H. defensa and L. fabarum). These results confirm that strong species- and genotype-specificity of symbiont-conferred resistance is indeed a hallmark of wild A. f. fabae populations, and they are consistent with symbiont-mediated adaptation of aphids to the parasitoids posing the highest risk.
Introduction
Host–parasite relationships are characterized by continuous adaptation and counteradaptation between the interacting species, a process referred to as antagonistic coevolution (Inouye, 2012). It requires heritable genetic variation in host resistance and parasite infectivity, which can be maintained by negative frequency-dependent selection when genotype-by-genotype interactions between hosts and parasites determine infection success (Agrawal & Lively, 2002; Hamilton, 1980), or when host resistance and parasite virulence are costly (Agrawal & Lively, 2002; Nuismer, 2006). Host–parasite coevolution may result in local adaptation, that is, adaptation of hosts to the local parasites, or of parasites to the local hosts. On average, the direction of the effect will depend on whether the host or the parasite has the upper hand in the coevolutionary arms race. Local adaptation may be promoted in the antagonist that shows comparatively high migration or mutation rates, as this increases the genetic variability upon which selection can act (Gandon & Michalakis, 2002; Gandon et al., 1996). Short generation times and high reproductive rates may also provide an advantage by allowing fast adaptation (Gandon et al., 1996; Kaltz & Shykoff, 1998). The latter is typical for many parasites, and indeed, local adaptation of parasites to their hosts is frequently observed (e.g., Ebert, 1994; Lively et al., 2004). However, also local adaptation of hosts to parasites, sometimes equated with parasite local maladaptation, can occur in natural systems (e.g., Kaltz et al., 1999; Lemoine et al., 2012; Oppliger et al., 1999). In either case, observed levels of resistance in a host population could originate from past and present selection imposed by the local environment, and in particular the local parasite community (Decaestecker et al., 2007; Kerfoot & Weider, 2004; Sadd & Schmid-Hempel, 2009; Schmid-Hempel & Ebert, 2003).
Selection for resistance acts not only on the host genome but may also affect resistance conferred by host-associated symbiotic organisms. So-called defensive symbionts bear the potential for rapid evolution of host resistance (e.g., Hedges et al., 2008; Jaenike et al., 2010; Teixeira et al., 2008) and may enable symbiont-driven host–parasite coevolution (Vorburger & Perlman, 2018). Many examples of defensive symbioses concern insects, which can harbour various endosymbiotic bacteria known to confer ecological benefits, including defence against pathogens, parasites, and predators (Florez et al., 2015; Oliver & Moran, 2009; Oliver et al., 2014). One of the most extensively studied insect defensive symbioses is that between aphids (Aphidoidea) and the gammaproteobacterium Hamiltonella defensa (Moran et al., 2005), a heritable endosymbiont known to provide several aphid species with resistance against parasitoid wasps (Asplen et al., 2014; Oliver et al., 2003; Vorburger et al., 2009). H. defensa is a facultative endosymbiont in that it is not necessary for aphid survival under benign conditions. Its presence even entails fitness costs for the aphid host in the absence of parasitoids (Dykstra et al., 2014; Oliver et al., 2008; Vorburger & Gouskov, 2011), which likely contributes to the fact that H. defensa is rarely fixed in natural aphid populations (e.g., Brady et al., 2014; Clarke et al., 2018; Sepúlveda et al., 2017). Individual aphids do usually not carry more than one H. defensa strain (Russell et al., 2013), but multiple strains can occur within a single aphid species (e.g., Henry et al., 2022; Leclair et al., 2016; Wu et al., 2022). Different H. defensa strains carry different variants of a bacteriophage residing in the bacterial genome (Degnan & Moran, 2008; Rouïl et al., 2020). These phage variants may encode distinct toxins that are likely involved in parasitoid resistance by inhibiting the development of the parasitoid egg or larva within the aphid (Brandt et al., 2017; Lynn-Bell et al., 2019; Oliver & Higashi, 2019; Oliver et al., 2009). H. defensa-conferred resistance against parasitoid wasps is therefore a variable and heritable trait that can be subject to selection by a local parasitoid community. Parasitoids, for their part, possess genetic variation for overcoming H. defensa-conferred resistance and can evolve counteradaptations to different H. defensa strains (Dennis et al., 2017; Dion et al., 2011; Rouchet & Vorburger, 2014).
In accordance with this, multiple studies have demonstrated strong variation and high specificity of H. defensa-conferred resistance to parasitism. A given strain of H. defensa can confer resistance against some parasitoid species but not against others (e.g., Asplen et al., 2014; Hopper et al., 2018; Łukasik et al., 2013; Martinez et al., 2016; McLean & Godfray, 2015), and it can also provide variable resistance against different genotypes of the same parasitoid species (e.g., Cayetano & Vorburger, 2013, 2015; Schmid et al., 2012). This suggests that local parasitoid communities can influence patterns and types of symbiont-conferred resistance in their hosts. However, much of the available evidence is based on experiments in which H. defensa strains and parasitoids were combined haphazardly, using lines collected from different sites or time points (e.g., Cayetano & Vorburger, 2013; Hopper et al., 2018; Schmid et al., 2012), or parasitoids obtained from commercial breeders of biocontrol agents (e.g., Asplen et al., 2014; Cayetano & Vorburger, 2015; Łukasik et al., 2013). The antagonists confronted in these experiments thus did not have a common evolutionary history, such that the experimentally observed specificities and apparent trade-offs for resistance are not necessarily representative of resistance patterns that occur in natural, locally (co)adapted insect communities.
In an attempt to improve on this, Wu et al. (2022) shuffled H. defensa strains among three aphid species found in the U.K. and then exposed each aphid species to its dominant parasitoid. They found that in the majority of cases, the aphids’ native H. defensa strains were most protective against the species’ dominant parasitoids, consistent with the hypothesis of symbiont-mediated adaptation to the host-specific parasitoid community. However, the focus on a single parasitoid species captures only part of the risk experienced by each host species, and we therefore took a different approach for the present study. We took advantage of a 2-year field study on the black bean aphid, Aphis fabae fabae, where we had collected very detailed information on (a) the relative abundances of different parasitoid species exploiting the local aphid populations near Zurich, Switzerland and (b) the prevalence of different H. defensa haplotypes in the same aphid populations (Gimmi et al., 2023). These data were used to design a full-factorial experiment in which we tested the ability of the five most frequent parasitoid species of A. f. fabae—using locally collected insects—to parasitize aphids carrying the two most frequent H. defensa haplotypes present in the local aphid populations. The results provide a comprehensive picture of the strength and specificity of symbiont-conferred resistance in a locally assembled, natural insect community.
Methods
Organisms
An overview of the background and setup of our experiment is presented in Figure 1. In the field study preceding the present experiment (Gimmi et al., 2023), we collected black bean aphids (A. f. fabae) and estimated their risk of infection by parasitoids on a monthly basis for two full growing seasons (2019 and 2020) at three rural sites in the close proximity of Zurich, Switzerland. Parasitoids were collected using sentinel hosts, i.e., susceptible, H. defensa-free black bean aphids that were brought to the field and exposed to the local parasitoid community. Parasitized aphids were returned to the laboratory to count and identify the hatching parasitoids. In total, we identified 5,029 individuals belonging to 11 parasitoid species (Gimmi et al., 2023). For the present experiment, we considered the five most frequent parasitoid species, which together made up 97% of the collected samples: Lysiphlebus fabarum (70% of the collected samples), Aphelinus chaonia (13%), Binodoxys angelicae (8%), Praon volucre (4%), and Ephedrus plagiator (2%). A. chaonia belongs to the family Aphelinidae, the other four species are braconid wasps (Braconidae) from the subfamily Aphidiinae. We established laboratory populations of these species with individuals collected during the second year of the field study. A. chaonia, B. angelicae, P. volucre, and E. plagiator are sexual species (arrhenotokous reproduction) and were bred as large cage populations on an H. defensa-free clone of A. f. fabae that was different from the clone used in the experiment (see below). L. fabarum is predominantly asexual (thelytokous reproduction), hence we initiated 11 asexual lines from single females and reared them on the same aphid clone as the other parasitoid species. We genotyped these lines at 10 microsatellite loci (Sandrock et al., 2007, 2011b), showing that they belonged to six different genotypes. For the experiment, we used four genetically different lines, including the two genotypes that had been collected multiple times (L.fab 5, collected five times and L.fab 1, collected twice), likely representing abundant genotypes of this species in Zurich, as well as two of the genotypes collected only once (L.fab 2 and 3).
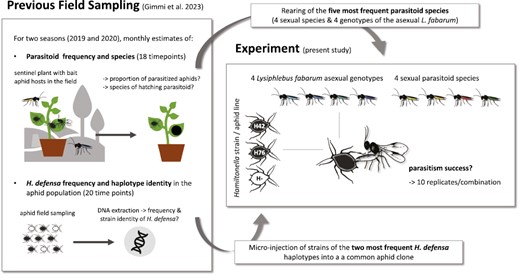
Graphic overview of the background and setup of our experiment. The field sampling took place in three rural areas in close proximity (<15 km) to Zurich, Switzerland.
In total, 35% of the 3,449 aphids collected in the field study carried H. defensa. Sequence typing of the symbiont in a subset of samples (n = 175) determined that 98% of the infected aphids carried the same known H. defensa haplotype (haplotype 2, Cayetano et al., 2015), while another 1% each carried the known haplotype 1 (Cayetano et al., 2015) and a previously unknown haplotype (Gimmi et al., 2023). For the present experiment, we assessed the resistance conferred by H. defensa haplotypes 1 and 2 in a common aphid genetic background, that is, we worked with three different lines of a single clone of A. f. fabae from our laboratory collection (clone ID: 407): one line carried no facultative endosymbiont (407), one line carried a H. defensa strain with haplotype 1 (strain 76, aphid line 407-H76), and one line carried a H. defensa strain with haplotype 2 (strain 42, aphid line 407-H42). The H. defensa-infected aphid lines had been created by microinjection of hemolymph from aphids carrying the desired H. defensa strains into the H. defensa-free 407 clone (Cayetano & Vorburger, 2015; Henry & Vorburger, unpublished). The common genetic background allowed us to exclude variation in resistance due to the aphid genotype, even though the endogenous resistance of A. f. fabae is likely low compared to H. defensa-conferred resistance (Vorburger et al., 2009). All aphid lines have been maintained parthenogenetically in the lab for multiple generations prior to the experiment. Aphid and parasitoid rearing and the experiment took place in a climate chamber at constant 19 °C and with a 16/8 hr light/dark cycle.
Experimental setup
We tested the parasitism success of eight parasitoid types (four sexual species, four different isofemale lines of the asexual L. fabarum) on three different aphid lines (407, 407-H42, and 407-H76) using a full-factorial design with 10 replicates per aphid line-parasitoid type combination (240 experimental units). The experiment was performed in 10 randomized complete blocks containing each one replicate of the 24 treatment combinations. Five blocks were processed on each of 2 consecutive days. An experimental unit consisted of a seedling of Vicia faba—the plant on which we routinely rear A. f. fabae—in a pot of ca. 5 cm diameter, covered by a ventilated plastic cage of ca. 15 cm height. The aphid lines were split up into 240 separate plants and reared for two generations prior to the start of the experiment, to avoid carrying over (grand)maternal environmental effects from the stock populations (Kindlmann & Dixon, 1989). On day 1 of the experiment, we transferred four adult aphids per experimental unit onto fresh V. faba seedlings. On day 2, the adult aphids were removed from the plants, leaving behind a cohort of aphid nymphs. We counted the number of nymphs on the plants on day 3 (average n = 18 ± 6 SD). On day 4, we added two female wasps to each of the plants and allowed them to parasitize the aphids for 8 hr. Fourteen days later (day 18), the successfully parasitized aphids were clearly recognizable as so-called mummies (parasitoid pupae within the emptied aphid husk). We counted the mummies and calculated the parasitism rate per experimental unit as the number of mummies divided by the number of nymphs that were initially exposed to the parasitoids.
Analysis
Data analysis was carried out with R version 4.2.3 (R Core Team, 2019) in Rstudio 2022.02.3 (RStudio Team, 2020) and we used ggplot2 3.3.5 (Wickham, 2016) for plotting. To test for overall patterns of H. defensa resistance, we first considered two models analyzing either the parasitism rates of the sexual parasitoid species (A. chaonia, B. angelicae, P. volucre, and E. plagiator) or those of the four asexual L. fabarum lines. For both data subsets, we applied generalized linear models (GLMs) to the proportion of aphids that got parasitized, using logit links and a quasibinomial error distribution to account for overdispersion. Anova from the R library car 3.0.7 (Fox & Weisberg, 2019) was used for analysis of deviance with F-tests as recommended for quasi-likelihood fits (Crawley, 2014). For the four sexual parasitoid species, we tested for the effects of the experimental block, aphid line, parasitoid species, and the aphid line × parasitoid species interaction. For the four L. fabarum lines, we tested for the effects of block, aphid line, L. fabarum line, and the aphid line × L. fabarum line interaction. Furthermore, we specifically tested for the protective effect of both H. defensa strains against each parasitoid species and each L. fabarum line. Because three combinations of lines resulted in zero mummies for all replicates, leading to problems with model convergence, we did not use GLMs for this but rather applied Kruskal–Wallis tests for overall differences among aphid lines for each parasitoid species or line separately. We then tested for a difference in parasitism rate between the H. defensa-free aphid line and either of the two H. defensa-infected aphid lines using two pairwise Mann–Whitney U tests with Bonferroni correction.
Results
Parasitoid species was the only significant effect in the analysis of parasitism rates achieved by the four sexual species A. chaonia, B. angelicae, P. volucre, and E. plagiator (Table 1a, Figure 2A). The effect is mainly driven by the low parasitism rates of B. angelicae (around 10% on all three aphid lines, Figure 2A) in contrast to the other species. The aphid line × parasitoid species interaction was marginally non-significant in this model (F6, 99 = 2.09, p = .0611, Table 1a), the relatively low p-value resulting mostly from the slight difference in parasitism by E. plagiator between the H. defensa-infected aphid lines 407-H42 and 407-H76 (Figure 2A). The main effect of the aphid line was not significant for the parasitism rates of the sexual species (Table 1a). This is in accordance with the fact that neither of the two H. defensa-infected aphid lines showed significantly changed parasitism rates compared to the H. defensa-free line when exposed to any of these parasitoid species (Figure 2A, Supplementary Table S1). The two tested H. defensa strains are therefore not protective against these parasitoid species in the studied field community.
Analysis of deviance table for the proportion of aphids parasitized (parasitism rate). Two generalized linear models with logit link and quasibinomial fit are shown: (a) on the data set containing only the sexual wasp species (dispersion parameter Ф = 2.38) and (b) on the data of L. fabarum lines only (Ф = 4.49).
. | Df . | Sum sq . | F . | p . |
---|---|---|---|---|
(a) Sexual parasitoid species (A. chaonia, B. angelicae, E. plagiator, P. volucre) | ||||
Block | 9 | 29.1 | 1.36 | 0.2176 |
Aphid line | 2 | 7.3 | 1.54 | 0.2203 |
Parasitoid species | 3 | 391.7 | 54.94 | <0.0001 |
Aphid line × parasitoid species | 6 | 29.8 | 2.09 | 0.0611 |
Residuals | 99 | 235.3 | ||
(b) Lysiphlebus fabarum | ||||
Block | 9 | 51.62 | 1.28 | 0.2585 |
Aphid line | 2 | 186.62 | 20.79 | <0.0001 |
L. fabarum line | 3 | 10.58 | 0.79 | 0.5047 |
Aphid line × L. fabarum line | 6 | 320.03 | 11.88 | <0.0001 |
Residuals | 99 | 444.41 |
. | Df . | Sum sq . | F . | p . |
---|---|---|---|---|
(a) Sexual parasitoid species (A. chaonia, B. angelicae, E. plagiator, P. volucre) | ||||
Block | 9 | 29.1 | 1.36 | 0.2176 |
Aphid line | 2 | 7.3 | 1.54 | 0.2203 |
Parasitoid species | 3 | 391.7 | 54.94 | <0.0001 |
Aphid line × parasitoid species | 6 | 29.8 | 2.09 | 0.0611 |
Residuals | 99 | 235.3 | ||
(b) Lysiphlebus fabarum | ||||
Block | 9 | 51.62 | 1.28 | 0.2585 |
Aphid line | 2 | 186.62 | 20.79 | <0.0001 |
L. fabarum line | 3 | 10.58 | 0.79 | 0.5047 |
Aphid line × L. fabarum line | 6 | 320.03 | 11.88 | <0.0001 |
Residuals | 99 | 444.41 |
Analysis of deviance table for the proportion of aphids parasitized (parasitism rate). Two generalized linear models with logit link and quasibinomial fit are shown: (a) on the data set containing only the sexual wasp species (dispersion parameter Ф = 2.38) and (b) on the data of L. fabarum lines only (Ф = 4.49).
. | Df . | Sum sq . | F . | p . |
---|---|---|---|---|
(a) Sexual parasitoid species (A. chaonia, B. angelicae, E. plagiator, P. volucre) | ||||
Block | 9 | 29.1 | 1.36 | 0.2176 |
Aphid line | 2 | 7.3 | 1.54 | 0.2203 |
Parasitoid species | 3 | 391.7 | 54.94 | <0.0001 |
Aphid line × parasitoid species | 6 | 29.8 | 2.09 | 0.0611 |
Residuals | 99 | 235.3 | ||
(b) Lysiphlebus fabarum | ||||
Block | 9 | 51.62 | 1.28 | 0.2585 |
Aphid line | 2 | 186.62 | 20.79 | <0.0001 |
L. fabarum line | 3 | 10.58 | 0.79 | 0.5047 |
Aphid line × L. fabarum line | 6 | 320.03 | 11.88 | <0.0001 |
Residuals | 99 | 444.41 |
. | Df . | Sum sq . | F . | p . |
---|---|---|---|---|
(a) Sexual parasitoid species (A. chaonia, B. angelicae, E. plagiator, P. volucre) | ||||
Block | 9 | 29.1 | 1.36 | 0.2176 |
Aphid line | 2 | 7.3 | 1.54 | 0.2203 |
Parasitoid species | 3 | 391.7 | 54.94 | <0.0001 |
Aphid line × parasitoid species | 6 | 29.8 | 2.09 | 0.0611 |
Residuals | 99 | 235.3 | ||
(b) Lysiphlebus fabarum | ||||
Block | 9 | 51.62 | 1.28 | 0.2585 |
Aphid line | 2 | 186.62 | 20.79 | <0.0001 |
L. fabarum line | 3 | 10.58 | 0.79 | 0.5047 |
Aphid line × L. fabarum line | 6 | 320.03 | 11.88 | <0.0001 |
Residuals | 99 | 444.41 |
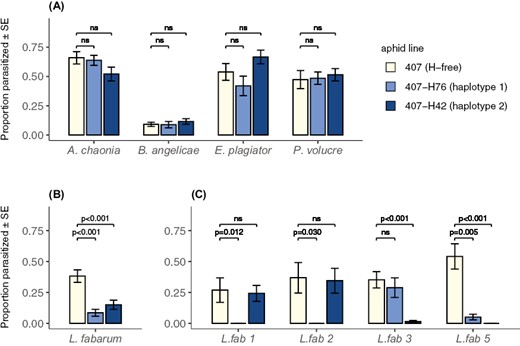
Mean parasitism rates calculated as number of mummies divided by number of exposed aphids. Panel (A) shows the four sexual parasitoid species on the x-axis, panel (B) shows the results for L. fabarum when averaging over the four different lines, and panel (C) shows each of the four different lines of L. fabarum separately. The p-values above the bars stem from pairwise Mann–Whitney U tests; values below 0.05 indicate a significant protective effect of the respective H. defensa strain against the wasp species or line after Bonferroni correction. Bar colours correspond to the three different aphid lines: 407 (light yellow, H. defensa-free), 407-H76 (light blue, H. defensa haplotype 1), and 407-H42 (dark blue, H. defensa haplotype 2).
In contrast, parasitism rates of L. fabarum were significantly dependent on the aphid line (Table 1b), with strongly reduced parasitism on the H. defensa-infected aphid lines 407-H42 and 407-H76 compared to the uninfected line 407 when averaging over all four L. fabarum lines (Figure 2B, Supplementary Table S1). There was also a highly significant interaction between aphid line and L. fabarum line (Table 1b), indicating genotype-specific protection by H. defensa: all four L. fabarum lines could parasitize the H. defensa-free aphids, the L. fabarum lines 1 and 2 could parasitize 407-H42 but not 407-H76, line 3 could parasitize 407-H76 but not 407-H42, and line 5 had very poor parasitism success on both H. defensa-infected aphid lines (Figure 2C, Supplementary Table S1). Both tested H. defensa strains thus protect against parasitism by L. fabarum, but the protection depends on the genotype of the attacking parasitoid.
Discussion
In a Swiss population of A. f. fabae, we tested the ability of two H. defensa haplotypes, the dominant haplotype (98%) and one of the rare haplotypes (1%), to protect aphids against the local parasitoid community. H. defensa conferred high levels of resistance only to the most abundant parasitoid species, L. fabarum, and this resistance acted in a genotype-specific manner (Figure 2, Table 1). That H. defensa-conferred resistance is differently effective against different parasitoid species has been demonstrated before (e.g., Asplen et al., 2014; Cayetano & Vorburger, 2015; Kraft et al., 2017; Martinez et al., 2016; McLean & Godfray, 2015), as have strong genotype-by-genotype interactions between L. fabarum and H. defensa (Cayetano & Vorburger, 2013; Gimmi & Vorburger, 2021; Schmid et al., 2012; Vorburger & Rouchet, 2016). However, previous experiments sometimes used commercially bred parasitoid stocks, or somewhat arbitrary combinations of host and parasitoid lines from different origins, making it unclear how representative the results are of interactions in natural populations. Our experiment shows that species- and genotype-level specificity of symbiont-conferred protection indeed prevails in a field population of black bean aphids and thus has the potential to affect the evolution of host resistance.
The fact that H. defensa provides aphids with protection against the most frequent parasitoid species is similar to the finding of Wu et al. (2022), who described that other aphid species’ native H. defensa strains provided protection against those species’ dominant parasitoids. It is indicative of host adaptation in response to parasite-mediated selection as also seen, for example, in experimental populations of Daphnia water fleas (Capaul & Ebert, 2003; Haag & Ebert, 2004)—with the difference that in our model system, host adaptation is realized via defensive symbiosis (Hafer-Hahmann & Vorburger, 2020; Oliver et al., 2008; Rossbacher & Vorburger, 2020). In this context, it is interesting that both tested H. defensa strains protected strongly against L. fabarum line 5, which appeared to be an abundant genotype in the field populations of L. fabarum (see Methods).
Even though our experiment was comprehensive in using all parasitoid species representing a significant risk for the studied aphid population, it remains a snapshot in space and time. As suggested by Thompson (2005), environmental variability and corresponding changes in community composition could result in mosaic-like variation of selection forces. It is possible, therefore, that we would have observed different resistance patterns if the same experiment had been carried out with insects and symbionts from another geographic area or year (Kaltz & Shykoff, 1998). For instance, Lenhart and White (2017) found no protection of H. defensa against a local parasitoid community in Aphis craccivora. Nevertheless, there are conditions under which we expect local adaptation of hosts to parasites (rather than parasite local adaptation) to prevail. One such condition is when the host is more mobile than the parasite (Gandon & Michalakis, 2002; Gandon et al., 1996; Greischar & Koskella, 2007). Many aphids, including A. f. fabae, are highly migratory and show wind-assisted dispersal (Loxdale et al., 1993). The frequently reported weakness of genetic differentiation between aphid populations even from very distant sites is consistent with such large-scale dispersion abilities (e.g., Llewellyn et al., 2003; Rattanawannee et al., 2019; Sandrock et al., 2011a). In contrast, aphid parasitoids generally seem to be poor dispersers (e.g., Nyabuga et al., 2010; Rauch & Weisser, 2007). The comparatively high dispersal abilities of hosts may distinguish the aphid-parasitoid system from other host–parasite systems with higher mobility of parasites, where local adaptation of parasites is the predominantly observed pattern (Greischar & Koskella, 2007; Hoeksema & Forde, 2008; Lively et al., 2004). Furthermore, generation time might be another important factor. As long as genetic variability is not limiting, adaptation of parasites to hosts may predominate in those interactions where parasites have much shorter generation times than their hosts (Gandon & Michalakis, 2002; Kaltz & Shykoff, 1998; Nee, 1989; Price, 1980). This clearly does not apply to parasitoids, which have similar or slightly longer generation times than aphids, making host local adaptation a likely alternative outcome.
The strong genotype-specificity of symbiont-conferred resistance makes the host’s benefit of harbouring H. defensa contingent on the genotypic composition of its main parasitoid. This could set the stage for intense and dynamic coevolution (Kwiatkowski et al., 2012). Specificity promotes negative frequency-dependent selection, which can account for the maintenance of genetic variation in both hosts and parasites due to the selective advantage of rare over common genotypes (Clarke, 1976; Judson, 1995). Laboratory studies have demonstrated that parasitoid (genotypic) diversity indeed bears the potential to maintain strain diversity among protective endosymbionts (Hafer & Vorburger, 2019; Hafer-Hahmann & Vorburger, 2020). The high degree of specificity we observed here among lines of a natural community lends credibility to the relevance of these previous studies and supports the importance of H. defensa as a driver of coevolutionary dynamics between aphids and parasitoids (Kwiatkowski et al., 2012; Vorburger, 2014). It is therefore surprising that the field survey preceding our experiment (Gimmi et al., 2023) revealed that H. defensa haplotype 2 was totally dominant in the A. f. fabae population (98% of the infected aphids carried this haplotype). This does not really fit with the picture of highly dynamic turnover of symbiont strains, especially since the same haplotype was already found at high prevalence in collections of Central European A. f. fabae that preceded ours by more than a decade (Cayetano et al., 2015). We do not currently know why H. defensa haplotype 2 remains dominant in our study area. This is particularly puzzling since we have evidence that haplotype 2 (here represented by strain H42) is somewhat less protective than haplotype 1 (H76) when averaged over multiple L. fabarum genotypes, and also more costly to the aphid hosts in the absence of parasitoids (Cayetano et al., 2015; Vorburger & Rouchet, 2016). Possible explanations include differences in the vertical transmission reliabilities between haplotypes, other ecological benefits provided by H. defensa that may obscure parasitoid-mediated selection (e.g., Gimmi et al., 2023; Smith et al., 2015, 2021), or that haplotype 2 outcompetes other strains of H. defensa, but these hypotheses remain to be tested.
All parasitoid species other than L. fabarum remained virtually unaffected by H. defensa in our experiment and mostly achieved parasitism rates as high or higher than L. fabarum. Low rates of parasitism were recorded only for B. angelicae (Figure 2A). This could suggest some endogenous resistance of A. f. fabae to these wasps, yet we observed that only a few aphids survived the exposure to B. angelicae during our experiment (E. Gimmi: pers. observation), suggesting that the low realized parasitism rates arose from increased aphid mortality after attack by B. angelicae, rather than from aphid resistance (see also Cayetano & Vorburger, 2015). There is no a priori reason why parasitoids of the genera Aphelinus, Binodoxys, Ephedrus, or Praon should not be susceptible to H. defensa-mediated defences: other studies have reported H. defensa-conferred protection against Aphelinus abdominalis in pea aphids (McLean & Godfray, 2015) or against two different species of Binodoxys in cowpea aphids (Asplen et al., 2014). To our knowledge, no protection by H. defensa against Praon or Ephedrus species has been reported yet, but the number of pertinent studies is low so far (Łukasik et al., 2013; Martinez et al., 2016). What shapes the effectiveness of (or susceptibility to) symbiont-conferred resistance in the context of an entire community of natural enemies is an interesting problem for further study. Since the only species susceptible to the defence provided by H. defensa was the predominantly asexual L. fabarum, we could speculate that the difference in reproductive mode among wasp species may impact the relative rates of adaptation. However, the genotypic diversity in asexual L. fabarum populations is remarkably high (Sandrock et al., 2011b), because there is the continuous potential for the formation of new asexual lines from coexisting (albeit rare) sexual lineages (Sandrock & Vorburger, 2011). We, therefore, expect that L. fabarum does not have a much diminished evolutionary potential compared to other parasitoid species.
Finally, it should be noted that by working with a single aphid clone, we completely ignored potential endogenous variation in aphid resistance to parasitoids for our experiment. Aphid populations exhibit genotypic variation in their susceptibility to parasitoids independently of carrying H. defensa (Martinez et al., 2014; Sandrock et al., 2010). This adds another layer of complexity to the coevolutionary interactions between aphids, endosymbionts, and parasitoids, though without denying the adaptive value of H. defensa-conferred resistance to the dominant parasitoid L. fabarum that we observe in black bean aphids.
Data availability
The data set generated in this study is available at Dryad Digital Repository: https://doi.org/10.5061/dryad.31zcrjdrp.
Author contributions
Elena Gimmi (Conceptualization [equal], Data curation [lead], Formal analysis [equal], Investigation [lead], Project administration [lead], Visualization [lead], Writing—original draft [lead], Writing—review & editing [equal]) and Christoph Vorburger (Conceptualization [equal], Formal analysis [equal], Funding acquisition [lead], Investigation [supporting], Resources [lead], Writing—original draft [supporting], Writing—review & editing [equal])
Funding
This research was funded by the Swiss National Science Foundation grant number 31003A_181969 to C.V.
Acknowledgments
We would like to thank Paula Rodriguez and Jesper Wallisch for their valuable help in insect maintenance and fieldwork.
Conflicts of interest
The authors have no conflict of interest to declare.