-
PDF
- Split View
-
Views
-
Cite
Cite
Tatiana L. Hernández‐Palma, Luis Alberto Rueda‐Solano, Janne K. Valkonen, Bibiana Rojas, Predator response to the coloured eyespots and defensive posture of Colombian four‐eyed frogs, Journal of Evolutionary Biology, Volume 36, Issue 7, 1 July 2023, Pages 1040–1049, https://doi.org/10.1111/jeb.14193
- Share Icon Share
Abstract
Deimatic displays, where sudden changes in prey appearance elicit aversive predator reactions, have been suggested to occur in many taxa. These (often only putative) displays frequently involve different components that may also serve antipredator functions via other mechanisms (e.g., mimicry, warning signalling, body inflation). The Colombian four‐eyed frog, Pleurodema brachyops, has been suggested to gain protection against predation through putative deimatic displays where they inflate and elevate the posterior part of their body revealing eye‐like colour markings. We exposed stationary artificial frogs to wild predators to test whether the two components (eyespot/colour markings, defensive posture) of their putative deimatic display, and their combination, provide protection from predation without the sudden change in appearance. We did not detect any obvious additive effect of defensive posture and eyespots/colour markings on predation risk, but found a marginally significant trend for model frogs in the resting posture to be less attacked when displaying eyespots/colour markings than when they were not, suggesting that the presence of colour markings/eyespots may provide some protection on its own. Additionally, we found that models in a resting posture were overall more frequently attacked on the head than models in a defensive posture, indicating that a defensive posture alone could help redirect predator attacks to non‐vital parts of the body. The trends found in our study suggest that the different components of P. brachyops' coloration may serve different functions during a deimatic display, but further research is needed to elucidate the role of each component when accompanied by sudden prey movement.
Abstract
INTRODUCTION
Predation is acknowledged as a strong selective force influencing the evolution of morphological, chemical and behavioural defence mechanisms in several organisms (Endler, 1986, 1991; Ruxton et al., 2018). One such defence strategy is aposematism, in which conspicuous colouration is coupled with a secondary defence that predators learn to avoid (Poulton, 1890). While warning coloration is usually studied as a static (i.e., always “on”) signal, it can also be associated with dynamic elements such as movement (Rößler et al., 2019). Such is the case of deimatic or ‘startle’ displays, in which the conspicuous coloration is not permanently visible, but only revealed under threat, often accompanied by distinctive behaviours (Umbers & Mappes 2015; Umbers et al., 2015; 2017).
Many species in several anuran families (Bufonidae: Caorsi et al., 2014; Odontophrynidae: Borgues‐Nojosa et al., 2016; Leptodactylidae: Sazima & Caramaschi, 1986; Cei, 1962, 1980; Cintra et al., 2014; Kolenc et al., 2009; Lenzi‐ Mattos et al., 2005; Toledo et al., 2011), are thought to use deimatic displays to deter predators. Such putative antipredator displays may involve arching the body to expose conspicuous coloration in the belly or vocal sac (i.e., the so‐called “Unken reflex”; Bajger, 1980), lifting the hind legs and lowering the anterior part of the body to reveal contrasting eyespots or noxious glands (Hödl & Amézquita, 2001; Toledo et al., 2011), or inflating the body.
The Colombian four‐eyed frog, Pleurodema brachyops, is one of the species thought to use a deimatic display as a defence mechanism against predators (Martins, 1989). Their putative display consists of multiple components, the most prominent of which are a defensive posture achieved by the inflation of their body and elevation of their hind parts, and the exposure of bright colour markings and so‐called ‘eyespots’ (Martins, 1989). These, in combination with noxious substances presumably secreted by the frogs' inguinal glands, are thought to stimulate different sensory modalities in would‐be predators upon attack (i.e., it could be considered a multimodal display; Partan & Marler, 2005; Rojas et al., 2019). While these components may altogether have synergistic effects and a deimatic function when accompanied by a sudden movement, the eyespots and defensive posture of this frog could also have protective effects on their own, even in the absence of a display, as shown in multiple study systems (see below). However, there is no experimental evidence available to date to confirm the predator‐deterrent nature of this display or its different components.
Eye‐like markings (i.e., eyespots), such as those present in the posterior dorsal region of the Colombian four‐eyed frog (Figure 1), have been shown to have protective effects against predator attacks via different mechanisms. For example, eyespots can act as deflective markings redirecting predator attacks towards less vulnerable body parts (Olofsson et al., 2010; Prudic et al., 2015). In the squinting bush brown butterfly, Bicyclus anynana, larger and brighter eyespots grant increased protection from predators compared to smaller, duller spots. In addition, while larger spots increase prey detection, they also make escape more likely, as attacks are deflected to non‐vital areas of the body such as the wing edges. Likewise, using artificial prey and three‐spined sticklebacks (Gasterosteus aculeatus) as predators, Kjernsmo and Merilaita (2017) found that spots smaller than the fish's own eyes attract attacks towards them, supporting the deflection hypothesis. Eyespots may also repel predators by in turn resembling their own predators' eyes (i.e., eye mimicry hypothesis), causing intimidation and confusion (De Bona et al., 2015; Kjernsmo & Merilaita, 2017); or simply by displaying conspicuous, high‐contrast colouration (Stevens, 2005; Stevens et al., 2008), which could be related to the evolution of an innate aversion by predators to stimuli which may appear dangerous (Skelhorn et al., 2016a).
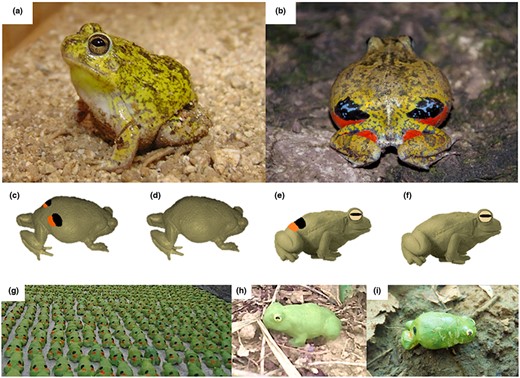
The Colombian four‐eyed frog, Pleurodema brachyops, in (a) resting posture and (b) defensive posture (note the eye‐like markings accompanied by conspicuous orange‐red spots, the body elevation, and the slight elevation of the posterior region of the body); and illustration of the four types of wax models deployed in the field: (c) defensive posture with visible eyespots/colour markings; (d) defensive posture without eyespots/colour markings; (e) resting posture with visible eyespots/colour markings; (f) resting posture without eyespots/colour markings; (g) Models ready to be deployed; (h) Non‐attacked model with resting posture and no eyespots/colour markings; (i) Model with defensive posture and eyespots/colour markings after a bird attack. Photos: (a), (c–i) Tatiana Hernández, (b) Giovanni Chaves.
Body elevation and inflation may also provide protection from predators on their own, or combined in a defensive posture. These phenomena may cause confusion and make prey appear larger than they are, thus discouraging attacks, particularly from small predators (Ferreira et al., 2019; Rojas & Burdfield‐Steel, 2017). Pufferfishes, for example, inflate their body when threatened or captured (Wainwright & Turingan, 1997), while some amphibians simultaneously elevate the pelvic region and inflate their body (Borgues‐Nojosa et al., 2016; Kolenc et al., 2009), presumably as part of a deimatic display. Interestingly, a defensive posture may either reduce the likelihood of attack altogether, or divert attacks away from vital parts of the body, as shown in salamanders of the genus Ambystoma (Myette et al., 2019). Furthermore, some toads (Jared et al., 2009; Stawikowski & Lüddecke, 2019; Pedroso‐Santos & Costa‐Campo, 2021) and salamanders (Brodie et al.,1979; Brodie & Smatresk, 1990) have been reported to adopt a defensive posture accompanied by the simultaneous display of parts of their body containing toxins (such as the parotid glands in bufonids) towards the predators. This has been suggested to increase the probability of said toxins being released upon attack (Toledo & Jared, 1995 ). Therefore, it is possible that the inflated body and raised hind legs of P. brachyops, which has lumbar glands (Duellman & Veloso, 1977; Ferraro et al., 2011), could be associated with this strategy.
Finally, the presence of eyespots and a defensive posture, and other traits such as body size, may have additive effects against predators. However, evidence in this direction is mixed. Skelhorn et al. (2016b), for example, found that birds learn more quickly that caterpillars with either only eyespots or only a defensive posture are profitable compared to caterpillars with both eyespots and a defensive posture, suggesting an advantage of displaying both components simultaneously. In contrast, Hossie and Sherratt (2013) found that while the presence of either eyespots or a defensive posture increased the probability of survival of caterpillars, exhibiting both components did not provide greater survival advantages, suggesting no additive effects of both traits against predation.
Here, we investigate the response of wild predators to artificial Colombian four‐eyed frogs with and without eyespots and colour markings (i.e., orange spots) in the inguinal region, either in a resting or a defensive posture, to determine whether these two components (defensive posture, eyespots/colour markings) of their putative deimatic display have additive effects (i.e., offer a higher protection when combined), or whether one component is more effective at preventing predator attacks than the other. We also test the alternative hypothesis that the defensive posture and eyespots/colour markings could provide protection against predation on their own, potentially via other mechanisms (e.g., warning signalling, eye mimicry). If the defensive posture and eyespots/colour markings of P. brachyops are enough to deter predators separately, we predict that models with the eyespot‐like and orange markings will deter predators irrespective of the frog's posture, and models with a defensive posture, will be attacked the least, irrespective of whether they display eyespot‐like and orange markings or not. If the defensive posture and eyespots/colour markings do not work in isolation but have additive effects, then we would expect models with eyespots/colour markings displaying a defensive posture to be attacked the least. Consequently, we would expect individuals in a resting position and no visible markings to be attacked the most. Alternatively, if the surprise element of the putative deimatic display is essential for predator deterrence, we predict that models with the eyespot‐like and orange markings displaying a defensive posture will be attacked the most, as they will be more easily detected, whereas individuals in a resting position and no visible markings will be attacked the least, presumably due to camouflage.
MATERIALS AND METHODS
Study species
The genus Pleurodema (Anura: Leptodactylidae) includes 15 species found in South America (Frost, 2018). Some of these species are known as “Four‐Eyed frogs” due to the presence of large lumbar glands (Ferraro et al., 2011) in the posterior part of their body. The Colombian four‐eyed frog, P. brachyops (Figure 1a), is a nocturnal and terrestrial species with an average size of 40 mm (Galvis et al., 2011) and variable greenish‐brown dorsal coloration (Hernández‐Palma et al., 2020) which contrasts with the large orange spots in the anterior part of the hind legs and the black lumbar glands, which are thought to look like a pair of eyes (Martins, 1989). It can be found in Tropical dry forest, pastures, roads and anthropogenic intervened areas located in the French Guiana, Panama, Venezuela, Colombia and Brazil, at elevations between 0 and 500 m (Blanco & Bonilla, 2010; Cochran & Goin, 1970; Cuentas et al., 2002; La Marca et al., 2010; Lynch & Romero, 2012). As is the case with six other species in the genus Pleurodema, P. brachyops has been reported to exhibit a deimatic display that presumably confers protection from visually oriented predators. This display includes body inflation, elevation of the hindparts, and the exhibition of its lumbar glands, orange spots and eyespots (Martins, 1989; Figure 1b). Because the eye‐like markings are accompanied by orange spots, it is likely that the putative deimatic display of these frogs is directed towards visually oriented predators, yet we are unaware of bird predation reports on P. brachyops. However, closely related species are hypothesised to share the same type(s) of predator(s) (Endler, 1986), and avian attacks have been documented in this and other species in the family Leptodactylidae (Andrade et al., 2013; Barroso et al., 2013; Fraga et al., 2014; Kokubum & Zacca, 2003; Vaz Ribeiro et al., 2019). Other predators of frogs of the genus Pleurodema, and closely related leptodactylids, include caimans (Thorbjarnarson, 1993), several snake species (Murcia et al., 2015), larger frogs (i.e., Ceratophrys calcarata; Pérez‐Anaya & Blanco‐Cervantes, 2015) and mammals such as ocelots (Bisbal, 1986) and Franged‐lipped bats (de Oliveira et al., 2020).
Study area
The study was done in four locations in the Magdalena department (Colombia): El Curval, Bonda (11°14′23.532″ N, 74°5′ 31.523″ W), Experimental farm at University of Magdalena (11°13′25.558″ N, 74°10′ 58.424″ W), La María, Ciénaga (10°58′51.265″ N, 74°13′47.59″ W) and Santa Rosalía, Zona Bananera (10°50′1.025″ N, 74°7′12.45″). The selected areas correspond to tropical dry forest patches and crop areas. We conducted the experiment between August and November 2018, during the breeding season of the species.
Model production
Prior to the elaboration of the models, we photographed 19 adult individuals of P. brachyops with a Sony DSC H‐300 compact camera set in manual mode with fixed image size, ISO 200, F3.0 lens aperture and shutter speed of 40 and next to a X‐Rite Colour Checker Passport, which provided a customized white standard, and a piece of graph paper for scale. We used the software Image J v. 1.52n (Abràmoff et al., 2004) to measure the snout‐vent length (SVL) and obtain RGB values of de dorsum and orange spots of every individual. We used the average RGB values of measurements in three sections of the dorsum and three of the orange spots to then match the paint colours to be used on the models.
To prepare the models we first created two polymer clay prototypes of P. brachyops, which were created taking a specimen from the Biological Collections Center of the University of Magdalena (CBUMAG: ANF: 00344) as reference. These prototypes were designed with two postures: a ‘resting’ posture, as frogs are commonly found in nature, and a defensive posture, which includes the elevation of the hind legs, body inflation, and slightly hidden head (Martins, 1989). From the polymer clay prototypes we made the silicon‐rubber moulds which were then filled with paraffin wax to obtain the frog models, following the procedures described in previous studies (Casas‐Cardona et al., 2018; Rojas et al., 2014). The standard size of the models was 3.5 cm ± 0.58, a measure obtained from the average of the SVL of the photographed frogs. Once the wax models were built, they were painted with odourless, non‐toxic paint (water‐based acrylic vinyl, Marfil Universal®) according to the colour treatment. Colour was standardized using an average of the RGB measurements obtained from the photographs mentioned above. RGB values do not take into account the UV light spectrum, which birds are able to see. However, we are unaware of UV reflection in the colour patterns of P. brachyops.
Predation experiment
We tested the effect of the defensive posture and eyespots/colour markings (Figure 1c–f) present in the putative deimatic display of P. brachyops by exposing 1000 frog models (Figure 1g), divided equally in four treatments, to natural predators in four different study areas. Two treatments included the eyespot/colour markings in the posterior dorsal region (Figure 1c and Figure 1e). These characteristic markings in P. brachyops consist of two rounded lumbar glands in a black colour accompanied by a pair of orange spots. These lumbar colour markings give the illusion of being a big pair of watching eyes (Ferreira et al., 2019; Martins, 1989). Four different types of models were thus used: defensive posture with visible eyespots/colour markings (Figure 1c); defensive posture without eyespots/colour markings (Figure 1d); resting posture with visible eyespots/colour markings (Figure 1e); and resting posture without eyespots/colour markings (Figure 1f,h). 250 models of each type were placed semi‐randomly (no two models of the same type were placed consecutively), distributed in equal numbers in each of the four locations. Model sequence was determined using an Excel‐based random numbers generator.
In each study site we distributed the models sequentially with an inter‐model distance of 5 m in all directions (see Figure S1). The models remained in the field for 3 days, with visits every 24 h to check for predation attempts on them. Models found with attack marks during the first (24 h) or second day (48 h) after deployment were removed and replaced with a new model (i.e., models that were found with attack marks during the last session [third day] were not replaced). Missing models were also replaced to keep the number of models within each category constant. Replacement models were treated as new replicates and their time to exposure to predation was counted from the moment at which they were deployed. All missing models were excluded from the data because we were unable to reliably attribute their absence to predation events. Models were exposed to predation for 24–72 h. Bird attacks were identifiable by V or U ‐shaped and stab‐like marks, whereas mammal attacks were identified by the bite marks, as reported in similar studies (Bordignon et al., 2018; Dreher et al., 2015; Noonan & Comeault, 2009; Rojas et al., 2014; Rönkä et al., 2020; Valkonen et al., 2011). Other attack marks, such as multiple small holes or scratches, were also recorded, but not included in our analyses. Here we focus primarily on bird attacks to emphasize the possible relevance of the visual component, and because other potentially important predators of P. brachyops, such as snakes, are very unlikely to “attack” clay models given their prominent use of chemoreception and thermoreception to detect prey (Rößler et al., 2018). We also recorded the area of the body where the model was attacked (head or rest of the body), as attacks in the head could be an additional indication that the predators do recognize them as prey. Alternatively, attacks in the ‘rest of the body’, particularly in models displaying the eyespot/colour markings, could be an indication that these markings might redirect predator attacks away from vital body parts.
Statistical analyses
Differences among the morphs in attack risk over time were analysed via a survival analysis (Cox Regression) using the probability of bird/mammal attack as a binary response variable, and the eyespots/colour markings (with/without), posture (resting/defensive), and their interaction as fixed factors. To account for the non‐independence of models within a study plot we included location as a random factor. We also took the subset of attacked models and tested the effect of eyespots/colour markings and posture (fixed factors) on the probability of attacks in the head using a Generalized Linear Mixed Model (GLMM) with binomial distribution and logit link function, and including location as a random effect. In all cases, we ran separate models for attacks by mammals and birds. All analyses were conducted in R (R Coreteam, 2013), using the RStudio (2015) platform, and the package coxme (Therneau, 2015).
RESULTS
Of the 1000 models initially deployed in our four study sites, 84 were replaced because they either went missing (N = 4) or were found with attack marks during the first or second day after deployment. The four missing frog models were excluded from further analysis because their disappearance could not be attributed to predation. This led to a final sample size of 284 with normal posture and absent eyespots/colour markings; 260 with normal posture and visible eyespots/colour markings; 264 with defensive posture and absent eyespots/colour markings; and 272 with defensive posture with visible eyespots and colour markings; thus, 1080 in total. 110 models (10.15%) were “attacked” and 47 (42.72%) of those attacks were attributable to birds, 47 (42.72%) to mammals, and 16 (14.5%) to either arthropods or unknown “attackers”. The latter were not taken into account in the analyses. The majority of bird attacks (36.1%) were found on models in resting posture without eyespots/colour markings (Table 1), whereas the lowest number (14. 8%) was found on models in resting posture with eyespots/colour markings (Table 1). Among the models attacked by mammals, most (31.9%) were found on models in resting posture without eyespots/colour markings (Table 1) and the lowest number of attacks (21.3%) was found on models with eyespots/colour markings in a defensive posture (Table 1).
Summary of attacks numbers by avian and mammalian predators according to treatment.
Distribution of attacks | Bird attacks | Mammal attacks | |||
Posture | Posture | ||||
Resting | Defensive | Resting | Defensive | ||
Eyespots/Colour markings | No | 17 | 10 | 15 | 11 |
Yes | 7 | 13 | 11 | 10 |
Distribution of attacks | Bird attacks | Mammal attacks | |||
Posture | Posture | ||||
Resting | Defensive | Resting | Defensive | ||
Eyespots/Colour markings | No | 17 | 10 | 15 | 11 |
Yes | 7 | 13 | 11 | 10 |
Summary of attacks numbers by avian and mammalian predators according to treatment.
Distribution of attacks | Bird attacks | Mammal attacks | |||
Posture | Posture | ||||
Resting | Defensive | Resting | Defensive | ||
Eyespots/Colour markings | No | 17 | 10 | 15 | 11 |
Yes | 7 | 13 | 11 | 10 |
Distribution of attacks | Bird attacks | Mammal attacks | |||
Posture | Posture | ||||
Resting | Defensive | Resting | Defensive | ||
Eyespots/Colour markings | No | 17 | 10 | 15 | 11 |
Yes | 7 | 13 | 11 | 10 |
When considering avian attacks, we found no support for our hypothesis that the eyespots/colour markings and defensive posture of P. brachyops have additive effects against predation, as models in a defensive posture displaying eyespots/colour markings were not attacked the least (Table 2; Figure 2a). Intriguingly, using a full model (pAttack~posture + eyespot/colourmarkings + posture × eyespot/colourmarkings + 1|location), we found a marginally significant interaction effect of posture and coloration on attack risk (Table 2; Figure 2a). Namely, there was a (non‐significant) trend for increased predation risk on models in a resting posture with no eyespots/colour markings and decreased attack risk on models in resting posture displaying eyespots/colour markings. This trend was not the same for models in a defensive posture. Neither eyespots/colour markings nor a defensive posture were found to provide a survival advantage on their own (Table 2; Figure 2a). An alternative model, which included only the intercept, had a slightly lower AIC, but, because ∂AIC of both models was <2, we opted to keep the full model as that was the most biologically relevant (see R script in Appendix S1). Among models attacked by birds, we found a significant effect of posture on the probability of the model to be attacked on the head. Thus, models in a defensive posture were less likely to be attacked on the head than models in the resting posture (estimate ± SE = −1.765 ± 0.831, z = −2.124, p = 0.034; Figure 2b), but there was no protective effect of colour alone (estimate ± SE = 0.288 ± 0.776, z = 0.371, p = 0.711).
Mixed effect Cox regression model estimating the hazard rates of artificial frogs, Pleurodema brachyops, to avian attacks.
Location | 0.017 | |||
Random effects | Variance | |||
Fixed effects | Estimate | SE | Z | p‐Value |
Eyespots/Colour markings (yes) | −0.894 | 0.449 | −1.99 | 0.047 |
Defensive posture | −0.528 | 0.398 | −1.32 | 0.190 |
Eyespots/Colour markings (yes)*Defensive posture | 1.142 | 0.615 | 1.86 | 0.063 |
Location | 0.017 | |||
Random effects | Variance | |||
Fixed effects | Estimate | SE | Z | p‐Value |
Eyespots/Colour markings (yes) | −0.894 | 0.449 | −1.99 | 0.047 |
Defensive posture | −0.528 | 0.398 | −1.32 | 0.190 |
Eyespots/Colour markings (yes)*Defensive posture | 1.142 | 0.615 | 1.86 | 0.063 |
Mixed effect Cox regression model estimating the hazard rates of artificial frogs, Pleurodema brachyops, to avian attacks.
Location | 0.017 | |||
Random effects | Variance | |||
Fixed effects | Estimate | SE | Z | p‐Value |
Eyespots/Colour markings (yes) | −0.894 | 0.449 | −1.99 | 0.047 |
Defensive posture | −0.528 | 0.398 | −1.32 | 0.190 |
Eyespots/Colour markings (yes)*Defensive posture | 1.142 | 0.615 | 1.86 | 0.063 |
Location | 0.017 | |||
Random effects | Variance | |||
Fixed effects | Estimate | SE | Z | p‐Value |
Eyespots/Colour markings (yes) | −0.894 | 0.449 | −1.99 | 0.047 |
Defensive posture | −0.528 | 0.398 | −1.32 | 0.190 |
Eyespots/Colour markings (yes)*Defensive posture | 1.142 | 0.615 | 1.86 | 0.063 |
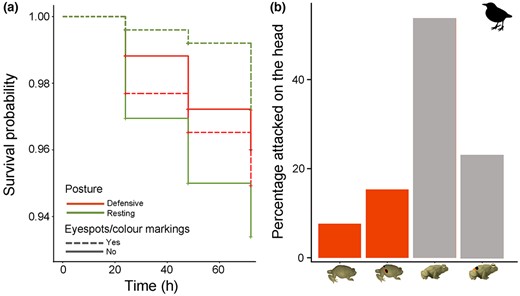
Avian predator attacks on the models deployed in the field. (a) Cumulative survival probability over time according to treatment. Models in resting posture with eyespots/colour markings had the highest survival, whereas models in resting posture and no eyespots/colour markings had the lowest. (b) Frequency of avian attacks on the head. Note that most attacks on the head were found on models in the resting posture (grey).
We found neither additive effects, nor effects of any variable in isolation, on predation risk (see Table 3; Figure S2a) or probability of head attack (eyespots/Colour markings: estimate ± SE = 0.566 ± 0.642, z = 0.882; posture: estimate ± SE = −0.736 ± 0.660, z = −1.11; p > 0.05 in both cases; Figure S2b) when considering only mammal attacks.
Mixed effect Cox regression model estimating the hazard rates of artificial frogs, Pleurodema brachyops, to mammal attacks. Full model used for comparison to attacks by birds.
Location | 8.15e‐5 | |||
Random effects | Variance | |||
Fixed effects | Estimate | SE | Z | p‐Value |
Eyespots/Colour markings (yes) | −0.314 | 0.397 | −0.79 | 0.43 |
Defensive posture | −0.299 | 0.397 | −0.75 | 0.45 |
Eyespots/Colour markings (yes)*Defensive posture | 0.197 | 0.590 | 0.33 | 0.74 |
Location | 8.15e‐5 | |||
Random effects | Variance | |||
Fixed effects | Estimate | SE | Z | p‐Value |
Eyespots/Colour markings (yes) | −0.314 | 0.397 | −0.79 | 0.43 |
Defensive posture | −0.299 | 0.397 | −0.75 | 0.45 |
Eyespots/Colour markings (yes)*Defensive posture | 0.197 | 0.590 | 0.33 | 0.74 |
Mixed effect Cox regression model estimating the hazard rates of artificial frogs, Pleurodema brachyops, to mammal attacks. Full model used for comparison to attacks by birds.
Location | 8.15e‐5 | |||
Random effects | Variance | |||
Fixed effects | Estimate | SE | Z | p‐Value |
Eyespots/Colour markings (yes) | −0.314 | 0.397 | −0.79 | 0.43 |
Defensive posture | −0.299 | 0.397 | −0.75 | 0.45 |
Eyespots/Colour markings (yes)*Defensive posture | 0.197 | 0.590 | 0.33 | 0.74 |
Location | 8.15e‐5 | |||
Random effects | Variance | |||
Fixed effects | Estimate | SE | Z | p‐Value |
Eyespots/Colour markings (yes) | −0.314 | 0.397 | −0.79 | 0.43 |
Defensive posture | −0.299 | 0.397 | −0.75 | 0.45 |
Eyespots/Colour markings (yes)*Defensive posture | 0.197 | 0.590 | 0.33 | 0.74 |
DISCUSSION
In this study, we used stationary frog models to test the antipredator effects of the defensive posture and eyespot/colour markings displayed by the Colombian four‐eyed frog, P. brachyops, in their putative deimatic display. We found no evidence that either the presence of eyespots/colour markings, or the defensive posture on their own influenced attack risk by mammalian or avian predators. However, we found an intriguing marginal interaction effect of posture and presence of eyespots/colour markings on the probability of bird attack: models in a resting posture, but not in a defensive posture, tended to have a lower attack risk when displaying eyespots/colour markings, but a higher attack risk in their absence. Among those models which received bird attacks, models in a resting posture were more likely to be attacked in the head than models in a defensive posture; this pattern was not found in models with mammalian attacks. We discuss the implications of our findings below.
The presence of eyespots/colour markings in combination with a defensive posture, consisting of inflating the body and lifting the hind limbs, have long been thought to work together as a display with which Colombian four‐eyed frogs, P. brachyops, deter predators. Our first working hypothesis was, thus, that these two traits (defensive posture, eyespots/colour markings) have additive antipredator effects (i.e., offer a higher protection when combined). However, we found no support for this hypothesis, as models with eyespots/colour markings in a defensive posture were not attacked the least. This result is in agreement with previous research. In a study on European vipers investigating whether their dorsal zig‐zag patterns and triangle‐shaped head have additive effects on protection from predators, Valkonen et al. (2011) found no added protection of a triangle‐shaped head in a snake model already exhibiting their characteristic zig‐zag pattern. Another study, investigating the protective value of eyespots and body inflation in caterpillars, reported no evidence of additive effects of these two traits against predation (Hossie & Sherratt, 2013). In contrast with our study, however, these two studies found that the presence of either component (the triangle‐shaped head or the zig‐zag pattern in the vipers, and the eyespots or defensive posture in the caterpillars) provided as much protection as the presence of both (Hossie & Sherratt, 2013; Valkonen et al., 2011). A potential explanation for the lack of support for an additive effect of eyespots/colour markings and defensive posture in our study could be its short duration. Skelhorn et al. (2016b) found that initial predator response to multicomponent deceptive signals was different from the response in the long‐term, such that multicomponent signals were retained for longer. This, as the authors suggest, may indicate that multicomponent signals are particularly effective when there are repeated encounters with the same predator (Skelhorn et al., 2016b). Because we only evaluated the initial, short‐term response of wild predators to our models, the possibility remains that our findings could have revealed an additive effect of eyespots/colour markings and defensive posture if the models had been deployed for longer, or collected and re‐deployed afterwards.
Our alternative hypothesis stated that the defensive posture and eyespots/colour markings could provide protection against predation on their own. While we found no evidence of a favourable effect of either the presence of colour markings/eyespots or defensive posture on the probability of attack by either predator type, we found an interesting marginally significant interaction showing a higher probability of survival for models in a resting posture with eyespots/colour markings than for models in the same posture but lacking colour markings/eyespots. This trend could indicate that visible eyespots/colour markings on a frog in the resting, normal posture may generate a neophobic reaction in potential visually oriented predators (Marples & Kelly, 2001; Stevens, 2005), which may not recognize them as prey. Alternatively, eyespots may act as an intimidating and distracting element, (Hossie & Sherratt, 2013; Kjernsmo et al., 2018; Kjernsmo & Merilaita, 2017), for example because they could mimic the eyes of a larger predator (De Bona et al., 2015). Whatever the mechanism, this suggests that eyespots/colour markings could offer some degree of protection per se, without being accompanied neither by a defensive posture nor by a sudden movement to startle the predator. Why we did not find any indication of a protective effect of eyespots/colour markings on models in a defensive posture is rather puzzling –if anything, those models were slightly more attacked by birds than models in a defensive posture without eyespots/colour markings. This could potentially mean that the combination of a static defensive posture with highly visible colour markings made the models much easier to detect and, in turn, to attack. At this point, however, any interpretation of this weak trend is highly speculative, and further research is needed to confirm or discard this hypothesis. Finally, the fact that models without any element of the putative deimatic display seem to have the highest probability of being attacked by bird predators, might be due to their similarity to real frogs. Admittedly, however, due to the low number of attacks despite the high number of models deployed, our study does not have enough power to provide conclusive evidence in support of these suggestive biological trends.
Interestingly, when we examined whether the models attacked by birds had marks on the head or on the rest of the body, we observed the highest number of attacks on the head in models in a resting position and no eyespots/colour markings. Altogether, models in a defensive posture were attacked in the head significantly less than those in a resting posture. A recent study using clay models of Ambystoma salamanders showed that a defensive posture alone is not effective at deterring predator attacks, but it can divert them from vital parts of the body (i.e., away from the head; Myette et al., 2019); something similar could be happening with the defensive posture of our models. This is not trivial, as avoiding an attack on the head may make the difference between dying and surviving a predation attempt.
In the putative deimatic display of the four‐eyed frog, the eyespot/colour markings and defence posture are supposed to be displayed suddenly and simultaneously (Martins, 1989), which is thought to confuse the predator upon attack (Umbers et al., 2017; Umbers & Mappes, 2016). Here, we show that, without the movement component of their putative deimatic displays, the eyespots/colour markings accompanied by a defensive posture do not have additive effects against avian predators, at least upon an initial encounter. This could mean that, either the surprise element (sudden movement) is essential for predator deterrence (even in the absence of accompanying conspicuous colour markings; Holmes et al., 2018), or that predators living in sympatry with the four‐eyed frog are already familiar with the appearance of the frogs when they adopt their defensive posture and expose their colour markings. Previous research has indeed shown that, while for deimatic displays to work predator learning is not required, predators may learn to expect the display and either avoid it or ignore it (Umbers et al., 2019). Hence, it would be interesting for future studies to test predator response to the sudden appearance of the eyespots/colour markings of P. brachyops accompanied by a defensive posture to better understand their role in this frog's putative deimatic displays.
We have shown that in static models deployed in the wild, the presence of eyespot/colour markings together with a defensive posture did not reduce the attacks by visually oriented predators, hinting at the sudden movement of prey during in deimatic displays as a key element for predator deterrence. Further research should thus focus on experimental approaches that allow testing predator response in a more interactive and realistic manner.
AUTHOR CONTRIBUTIONS
Tatiana L. Hernández‐Palma: Conceptualization (equal); data curation (equal); formal analysis (equal); funding acquisition (lead); investigation (lead); methodology (equal); writing – original draft (supporting); writing – review and editing (supporting). Luis Alberto Rueda‐Solano: Conceptualization (equal); data curation (equal); funding acquisition (lead); investigation (supporting); methodology (equal); project administration (equal); resources (equal); supervision (equal); writing – review and editing (equal). Janne K. Valkonen: Conceptualization (equal); formal analysis (equal); methodology (equal); writing – review and editing (supporting). Bibiana Rojas: Conceptualization (equal); data curation (equal); formal analysis (lead); funding acquisition (supporting); methodology (equal); supervision (equal); visualization (lead); writing – original draft (lead); writing – review and editing (lead).
ACKNOWLEDGEMENTS
We are grateful to L. Benavides, A. Barros and the Herpetological Research Group of the University of Magdalena for help during field activities and wax model elaboration. We thank S. Contreras for making the moulds. Fundación Atelopus, GIBEA and MIKU research groups helped with logistics and materials. Many thanks to Alice Exnerová, Tom Sherratt and an anonymous reviewer for their helpful comments and constructive feedback. BR is thankful to Johanna Mappes for insightful discussion. This study was funded by Asociación Colombiana de Herpetología (ACH) via a “Botas al Campo” research grant to TLH. BR and JKV were funded by the Academy of Finland (Academy Research Fellowship, Decision Nos. 318404 and 319949 to BR). We highly value equity, diversity, and inclusion in science (EDI) and thus took into account EDI best practice before and during our study. Our team includes researchers from both the so‐called Global North (Finland) and Global South (Colombia), and diverse backgrounds and career stages—from a Bachelor's student to an established PI—all of which significantly contributed to the quality of our study.
CONFLICT OF INTEREST STATEMENT
We declare no conflicts of interest.
PEER REVIEW
The peer review history for this article is available at https://www.webofscience.com/api/gateway/wos/peer‐review/10.1111/jeb.14193.
DATA AVAILABILITY STATEMENT
Data are openly available in the DRYAD repository http://doi.org/10.5061/dryad.n8pk0p31d.
REFERENCES
Supplementary data
Appendix S1
Figure S1
Figure S2