-
PDF
- Split View
-
Views
-
Cite
Cite
Casper J. van der Kooi, Karim Ghali, David Amptmeijer, Tanja Schwander, Niche differentiation among clones in asexual grass thrips, Journal of Evolutionary Biology, Volume 32, Issue 1, 1 January 2019, Pages 126–130, https://doi.org/10.1111/jeb.13393
- Share Icon Share
Abstract
Many asexual animal populations comprise a mixture of genetically different lineages, but to what degree this genetic diversity leads to ecological differences remains often unknown. Here, we test whether genetically different clonal lineages of Aptinothrips grass thrips differ in performance on a range of plants used as hosts in natural populations. We find a clear clone‐by‐plant species interactive effect on reproductive output, meaning that clonal lineages perform differently on different plant species and thus are characterized by disparate ecological niches. This implies that local clonal diversities can be driven and maintained by frequency‐dependent selection and that resource heterogeneity can generate diverse clone assemblies.
INTRODUCTION
The continuous generation of genetic variation for fitness is regarded as one of the main explanations for the predominance of sex in eukaryotes (Bell, 1982). Theoretical studies have shown that under some conditions, sex can be favoured over asexuality because it creates novel and/or rare genotypes (Bell, 1982; Otto, 2009). Such novel and rare genotypes are favoured under negative frequency‐dependent selection or in habitats with spatially heterogeneous or temporally fluctuating resources. For example, one component of the “parasite hypothesis for sex” implies negative frequency‐dependent selection, as it predicts that parasites evolve to track common host genotypes (Dagan, Liljeroos, Jokela, & Ben‐Ami, 2013; Decaestecker et al., 2007; Morran, Schmidt, Gelarden, Parrish, & Lively, 2011). Similar ideas are available for selection imposed by resources; that is rare genotypes are able to exploit resources where competition is low (reviewed by Scheu & Drossel, 2007).
Theories for the maintenance of sex generally assume genetically uniform populations of asexuals, whereas in nature, many asexual animal and plant populations comprise genetically distinct clones (Bell, 1982; Ellstrand & Roose, 1987; Jokela, Dybdahl, & Lively, 1999; Parker, 1979). Indeed, high genetic diversity is the case in many asexual populations (Fontcuberta García‐Cuenca, Dumas, & Schwander, 2016; Kearney et al., 2009; Moritz, Donnellan, Adams, & Baverstock, 1989; Neiman, Jokela, & Lively, 2005; Pannebakker, Zwaan, Beukeboom, & Van Alphen, 2004; Tully & Ferrière, 2008; Vorburger, Lancaster, & Sunnucks, 2003), but whether clonal diversity is generally associated with phenotypic and ecological diversity remains unknown. Genetic diversity in asexuals can be due to lineage diversification after the transition to asexuality (owing to mutation accumulation) and because asexuals have different sexual ancestors; that is they arose via independent transitions to asexuality (Janko, Drozd, Flegr, & Pannell, 2008). Such independent transitions are especially likely to generate ecological differentiation (Bell, 1982; Janko et al., 2008; King, Jokela, & Lively, 2011). More generally, it will be difficult to explain the maintenance of sex via its effect on genetic diversity if sexual species compete with genetically diverse rather than genetically uniform asexuals (Judson, 1997).
In this study, we test whether genetic differentiation in asexual Aptinothrips (Thysanoptera: Thripidae) grass thrips is neutral or ecologically relevant. Aptinothrips is a system where asexuality has evolved several times independently and is associated with a large geographic distribution and extreme genetic diversity (van der Kooi & Schwander, 2014). We test whether thirteen genetically different clonal lineages of Aptinothrips rufus and Aptinothrips stylifer occupy different ecological niches by examining their performance on seven common host plant species. In a breeding experiment and using the number of offspring produced as a proxy for fitness, we show that different clonal lineages indeed feature different ecological niches.
MATERIALS AND METHODS
Study species and breeding
Aptinothrips grass thrips are small (1–2 mm), wingless insects that feed on various species of Poaceae. For A. rufus, asexuality evolved at least twice independently (van der Kooi & Schwander, 2014) as inferred from phylogenies based on mitochondrial sequences. Asexual populations of this species can be found all over the world and exhibit very high levels of mitochondrial diversity, whereas sexual populations only occur in the Mediterranean region and exhibit a relatively low mitochondrial diversity (Fontcuberta García‐Cuenca et al., 2016; van der Kooi & Schwander, 2014). The species A. stylifer evolved asexuality independently from A. rufus (van der Kooi & Schwander, 2014), and different populations also comprise a pool of genetically different individuals (see Results). Nine and four genetically different females of A. rufus and A. stylifer were isolated from three locations in Switzerland (Supporting Information). These individuals were used to establish thirteen iso‐female lines (hereafter: clonal lineages); for each lineage, third‐generation individuals were used in the experiment.
Experimental design
We tested the performance of asexual females on seven grass species that are commonly found in natural habitats: meadow brome (Bromus erectus), alpine bluegrass (Poa alpina), common meadow‐grass (Poa pratensis), red fescue (Festuca rubra), sheep fescue (Festuca ovina), Timothy grass (Phleum pratense) and orchard grass (Dactylis glomerata). For each clonal lineage, 7–14 replicate individuals were tested on each host plant species; this yielded a total of 735 replicates (see Supporting Information). The experiment was performed in July–August 2016; for logistic reasons, it was performed in two batches separated by 4 days.
Data analysis
To test for ecological variation among species and clonal lineages of Aptinothrips, we used linear mixed models (LMMs) as implemented in the R package lme4 (Bates, Maechler, Bolker, & Walker, 2014) in R 3.4.2. We tested whether thrips species, independent transitions to asexuality within A. rufus, clonal lineage, the grass species and their interactions affected the number of offspring. Batch was included as a random factor in the model. Significance was tested using a permutation ANOVA (Supporting Information).
RESULTS
We observed clear differences in the number of offspring produced by asexual Aptinothrips (Figure 1). There was an effect of thrips species (df = 1, p < 0.01) and an effect of clade, that is, independent transitions to asexuality (df = 2, p < 0.01), on the number of offspring produced. In line with our hypothesis of ecological divergence, different clonal lineages are characterized by different ecologies, as revealed by significant clone‐by‐plant species interaction effects on clonal lineage performances (df = 72, p < 0.01). Ecological differences among clonal lineages are not solely explained by independent transitions to asexuality as clonal lineages within one A. rufus clade (clade B in Figure 1) also differ from each other (df = 12, p = 0.04); the small sample sizes in A. stylifer preclude performing a similar analysis.
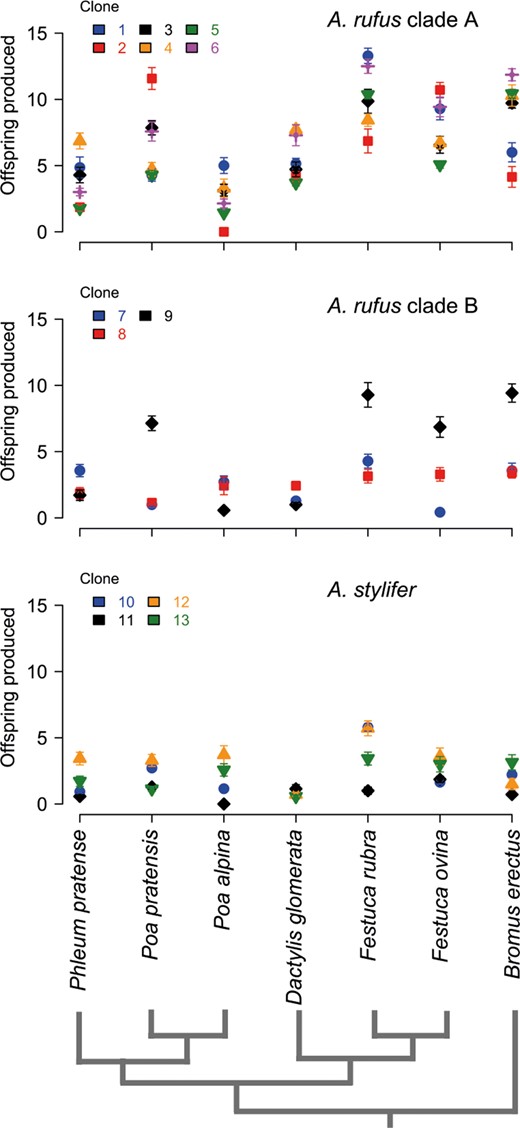
Number of offspring produced by different asexual Aptinothrips lineages on seven host plant species. Every panel depicts the asexual lineages of an independently evolved asexual clade (identified in van der Kooi and Schwander (2014)). For every lineage, the mean ± 95% confidence interval (assuming a normal distribution) is shown
DISCUSSION
Many asexual populations comprise a large number of different clonal lineages (Bell, 1982; Parker, 1979), but whether genetically different clones feature different ecologies is largely unknown. In this study, we showed that different clonal Aptinothrips lineages feature different ecological niches (Figure 1). Ecological divergence of clonal lineages is pronounced both within and between independently derived asexual clades. Although assessing the relative importance of independent transitions versus within clade divergence requires deeper sampling, the multiple origins of asexuality in Aptinothrips (van der Kooi & Schwander, 2014) have increased the genetic diversity in asexuals and likely contribute to their extreme ecological success.
The facts that asexual Aptinothrips populations exhibit a higher genetic diversity than sexuals (Fontcuberta García‐Cuenca et al., 2016; van der Kooi & Schwander, 2014) and that this genetic diversity translates to ecological divergence (this study) may explain why asexuals outcompete sexuals in mesocosm experiments (Lavanchy et al., 2016). Our findings further suggest that locations with different grass species would select for different sets of clonal lineages. High grass species diversity is expected to promote and maintain clonal diversity in the thrips population, because more grass species reflect more niches available for different clonal lineages. An interesting open question is whether temporal fluctuations in plant species frequency lead to fluctuating clone frequencies, as was previously found in the aphid Myzus persicae by Vorburger (2006).
A review of literature showed that clonal diversity is associated with ecological diversity in a suite of at least nine additional plant and animal species (Table 1). This includes aquatic and terrestrial asexual systems with very diverse ecologies. Taken together, these studies convincingly show that populations of clonal animals comprise ecologically relevant genetic variation and that clonal diversity should therefore not be disregarded in theory on the maintenance of sex in nature.
Overview of species that show ecological diversification of clonal lineages
Asexual species investigated | Common name | Approach | Results | Reference |
Erigeron annuus | Eastern daisy fleabane | Experimentally test the number and size of seeds, biomass allocation and seed germination for different lineages. | Variation in seed size, allocation to roots and seed germination for different lineages. | Stratton (1991) |
Taraxacum officinale | Dandelion | Experimentally test the effect of drought and shade on biomass allocation for different lineages. | Lineages often perform differently under different conditions. | Oplaat and Verhoeven (2015) |
Rhopalosiphum padi | Cherry oat aphid | Experimentally test fecundity and longevity of clones on different plant species at two temperatures. | Small differences between lineages; performance in laboratory experiment is correlated with abundance in natural populations. | Valenzuela, Ridland, Weeks, and Hoffmann (2010) |
Myzus persicae | Peach potato aphid | Experimentally test reproductive success of lineages on different host plant species. | Lineages perform differently on different host plant species. | Vorburger, Sunnucks, and Ward (2003) |
Folsomia candida | Springtail | Experimentally test reproductive investment and survival for different lineages. | Significant variation between lineages in number and size of offspring produced. | Tully and Ferrière (2008) |
Daphnia pulex | Water flea | Experimentally test lineage performance in different locations with different salinities. | Lineages perform differently in different regions, consistent with local adaptation. | Weider and Hebert (1987) |
Potamopyrgus antipodarum | Fresh water snail | Field sampling in different populations. | In different populations, different lineages feature specific dietary preferences. | Jokela et al. (1999) |
Heterontia binoei | Gecko | Field sampling of lineages in natural populations with different climates. | Lineages with different origins occupied different geographic regions and niches. | Kearney, Moussalli, Strasburg, Lindenmayer, and Moritz (2003) |
Lepidodactylus lugubris | Gecko | Field sampling of lineages in natural habitats. | Two common lineages feature different niches, though with some overlap. | Hanley, Bolger, and Case (1994) |
Aptinothrips rufus & A. stylifer | Grass thrips | Experimentally test performance of different lineages on seven common host plant species. | Lineages perform differently on different host plant species | This study |
Asexual species investigated | Common name | Approach | Results | Reference |
Erigeron annuus | Eastern daisy fleabane | Experimentally test the number and size of seeds, biomass allocation and seed germination for different lineages. | Variation in seed size, allocation to roots and seed germination for different lineages. | Stratton (1991) |
Taraxacum officinale | Dandelion | Experimentally test the effect of drought and shade on biomass allocation for different lineages. | Lineages often perform differently under different conditions. | Oplaat and Verhoeven (2015) |
Rhopalosiphum padi | Cherry oat aphid | Experimentally test fecundity and longevity of clones on different plant species at two temperatures. | Small differences between lineages; performance in laboratory experiment is correlated with abundance in natural populations. | Valenzuela, Ridland, Weeks, and Hoffmann (2010) |
Myzus persicae | Peach potato aphid | Experimentally test reproductive success of lineages on different host plant species. | Lineages perform differently on different host plant species. | Vorburger, Sunnucks, and Ward (2003) |
Folsomia candida | Springtail | Experimentally test reproductive investment and survival for different lineages. | Significant variation between lineages in number and size of offspring produced. | Tully and Ferrière (2008) |
Daphnia pulex | Water flea | Experimentally test lineage performance in different locations with different salinities. | Lineages perform differently in different regions, consistent with local adaptation. | Weider and Hebert (1987) |
Potamopyrgus antipodarum | Fresh water snail | Field sampling in different populations. | In different populations, different lineages feature specific dietary preferences. | Jokela et al. (1999) |
Heterontia binoei | Gecko | Field sampling of lineages in natural populations with different climates. | Lineages with different origins occupied different geographic regions and niches. | Kearney, Moussalli, Strasburg, Lindenmayer, and Moritz (2003) |
Lepidodactylus lugubris | Gecko | Field sampling of lineages in natural habitats. | Two common lineages feature different niches, though with some overlap. | Hanley, Bolger, and Case (1994) |
Aptinothrips rufus & A. stylifer | Grass thrips | Experimentally test performance of different lineages on seven common host plant species. | Lineages perform differently on different host plant species | This study |
Overview of species that show ecological diversification of clonal lineages
Asexual species investigated | Common name | Approach | Results | Reference |
Erigeron annuus | Eastern daisy fleabane | Experimentally test the number and size of seeds, biomass allocation and seed germination for different lineages. | Variation in seed size, allocation to roots and seed germination for different lineages. | Stratton (1991) |
Taraxacum officinale | Dandelion | Experimentally test the effect of drought and shade on biomass allocation for different lineages. | Lineages often perform differently under different conditions. | Oplaat and Verhoeven (2015) |
Rhopalosiphum padi | Cherry oat aphid | Experimentally test fecundity and longevity of clones on different plant species at two temperatures. | Small differences between lineages; performance in laboratory experiment is correlated with abundance in natural populations. | Valenzuela, Ridland, Weeks, and Hoffmann (2010) |
Myzus persicae | Peach potato aphid | Experimentally test reproductive success of lineages on different host plant species. | Lineages perform differently on different host plant species. | Vorburger, Sunnucks, and Ward (2003) |
Folsomia candida | Springtail | Experimentally test reproductive investment and survival for different lineages. | Significant variation between lineages in number and size of offspring produced. | Tully and Ferrière (2008) |
Daphnia pulex | Water flea | Experimentally test lineage performance in different locations with different salinities. | Lineages perform differently in different regions, consistent with local adaptation. | Weider and Hebert (1987) |
Potamopyrgus antipodarum | Fresh water snail | Field sampling in different populations. | In different populations, different lineages feature specific dietary preferences. | Jokela et al. (1999) |
Heterontia binoei | Gecko | Field sampling of lineages in natural populations with different climates. | Lineages with different origins occupied different geographic regions and niches. | Kearney, Moussalli, Strasburg, Lindenmayer, and Moritz (2003) |
Lepidodactylus lugubris | Gecko | Field sampling of lineages in natural habitats. | Two common lineages feature different niches, though with some overlap. | Hanley, Bolger, and Case (1994) |
Aptinothrips rufus & A. stylifer | Grass thrips | Experimentally test performance of different lineages on seven common host plant species. | Lineages perform differently on different host plant species | This study |
Asexual species investigated | Common name | Approach | Results | Reference |
Erigeron annuus | Eastern daisy fleabane | Experimentally test the number and size of seeds, biomass allocation and seed germination for different lineages. | Variation in seed size, allocation to roots and seed germination for different lineages. | Stratton (1991) |
Taraxacum officinale | Dandelion | Experimentally test the effect of drought and shade on biomass allocation for different lineages. | Lineages often perform differently under different conditions. | Oplaat and Verhoeven (2015) |
Rhopalosiphum padi | Cherry oat aphid | Experimentally test fecundity and longevity of clones on different plant species at two temperatures. | Small differences between lineages; performance in laboratory experiment is correlated with abundance in natural populations. | Valenzuela, Ridland, Weeks, and Hoffmann (2010) |
Myzus persicae | Peach potato aphid | Experimentally test reproductive success of lineages on different host plant species. | Lineages perform differently on different host plant species. | Vorburger, Sunnucks, and Ward (2003) |
Folsomia candida | Springtail | Experimentally test reproductive investment and survival for different lineages. | Significant variation between lineages in number and size of offspring produced. | Tully and Ferrière (2008) |
Daphnia pulex | Water flea | Experimentally test lineage performance in different locations with different salinities. | Lineages perform differently in different regions, consistent with local adaptation. | Weider and Hebert (1987) |
Potamopyrgus antipodarum | Fresh water snail | Field sampling in different populations. | In different populations, different lineages feature specific dietary preferences. | Jokela et al. (1999) |
Heterontia binoei | Gecko | Field sampling of lineages in natural populations with different climates. | Lineages with different origins occupied different geographic regions and niches. | Kearney, Moussalli, Strasburg, Lindenmayer, and Moritz (2003) |
Lepidodactylus lugubris | Gecko | Field sampling of lineages in natural habitats. | Two common lineages feature different niches, though with some overlap. | Hanley, Bolger, and Case (1994) |
Aptinothrips rufus & A. stylifer | Grass thrips | Experimentally test performance of different lineages on seven common host plant species. | Lineages perform differently on different host plant species | This study |
Asexuals are frequently more ecologically successful than related sexuals, which is often believed to be due to the reproductive assurance conferred by asexuality (e.g., Bell, 1982), but this may not be the sole explanation. For example, a recent large‐scale comparative study showed that asexuals have 2–3.5 times as many host species and occur in up to five times as many countries compared to their sexual counterparts, highlighting the (ecological) success of asexuality (van der Kooi, Matthey‐Doret, & Schwander, 2017). Our current study shows that in addition to the reproductive advantage of asexuality, high genetic diversity can contribute to the success of asexuals.
To summarize, genetic diversity in asexual Aptinothrips lineages is associated with ecological diversity. Under such ecological differentiation of clonal lineages, which appears to occur in a suite of asexual animal and plant systems (Table 1), natural selection may maintain clonal diversity rather than sex. Future theory on the maintenance of sex would benefit from incorporating clonal diversity, different clonal diversity‐generating mechanisms and their ecological significance.
ACKNOWLEDGMENTS
We acknowledge the members of the Schwander group for interesting discussions.
CONFLICT OF INTEREST
The authors declare no competing interests.
AUTHOR CONTRIBUTIONS
CJvdK, KG and TS designed the study. CJvdK, DA and KG carried out the experiment and analysed the data. CJvdK and KG wrote the first draft of the manuscript. All authors edited the manuscript and approved the final version.
REFERENCES
Author notes
Funding information
This research was funded by Grant PP00P3_139013 of the Swiss National Science Foundation (SNSF).
Shared first authorship.
Supplementary data