-
PDF
- Split View
-
Views
-
Cite
Cite
Joomi Lee, Dai-Yun Wu, Jih-Hsuan (Tammy) Lin, Jooyoung Kim, Sun Joo (Grace) Ahn, Using time travel in virtual reality (VR) to increase efficacy perceptions of influenza vaccination, Journal of Computer-Mediated Communication, Volume 28, Issue 3, May 2023, zmad010, https://doi.org/10.1093/jcmc/zmad010
- Share Icon Share
Abstract
This study examined the unique affordance of time travel in virtual reality (VR) to enhance the perceived efficacy of influenza vaccination. Effective vaccine communication hinges on raising awareness of the risk of contracting a contagious virus and spreading the infection to others. According to the extended-parallel process model, behavioral changes are achieved when an individual perceives sufficient levels of threat and efficacy to prevent negative health outcomes. Findings from a 2 (interactivity: active vs. passive) × 2 (virtual time travel: yes vs. no) between-subjects experiment (N = 178) indicated that virtual time travel to receive vaccination after experiencing negative consequences of influenza in VR increased participants’ perceived efficacy of vaccination for self-protection and community protection. Moreover, interactivity in VR enhanced vaccination intention, mediated by spatial presence, message involvement, and response efficacy.
Lay Summary
This study explored how virtual reality (VR) can be leveraged to facilitate effective vaccine communication. VR enables users to interact with virtual people and objects through real-time actions in a 3D space, allowing them to experience vaccine efficacy firsthand by engaging in preventive behaviors. We examined the impact of virtual time travel, a unique VR simulation technique, wherein unvaccinated participants observed negative consequences of influenza infection and then traveled back in time to receive the vaccine and defeat the virus. We found that virtual time travel increased efficacy perceptions and vaccination intention for both protecting the self and others in the community. Moreover, interactivity in VR through the use of handheld controllers to actively combat the viruses intensified the sense of “being there” in the virtual environment and personal involvement with the message, ultimately promoting efficacy perceptions and vaccination intention.
Communicating the importance of influenza vaccination to healthy adults is challenging because many adults only have mild-to-moderate illness after contracting the virus. However, vaccination efficacy is not only solely based on protecting healthy adults from severe illness, but also on preventing the spread of contagious illness to more vulnerable populations (CDC, 2021). Therefore, vaccine messages should effectively communicate both the direct benefits of vaccination to the individual and the indirect benefits for vulnerable others.
A recent study demonstrated the potential of immersive virtual reality (VR) in communicating key immunization concepts to healthy, vaccine-avoidant adults (Nowak et al., 2020). Compared with text or video modalities that delivered the same message content, VR enabled participants to feel as if they were “there” in the virtual space, undergoing the simulated experience at the moment (i.e., presence; Lombard & Ditton, 1997), which heightened concerns about the threat of the virus and increased vaccination intention.
Building on these findings, we aimed to identify the affordances of VR driving these changes and to shed insight into the psychological mechanisms of virtual experiences. This inquiry is guided by the extended-parallel process model (EPPM; Witte, 1992), focusing on how VR can bolster perceived efficacy and promote behavioral change when sufficient perceived threat is present. EPPM posits that without increasing perceived efficacy through clear, actionable mitigation plans, threat appeals in health messages may result in fear and avoidance, failing to promote the adoption of recommended behaviors (Witte, 1992). However, efficacy components in health messages, which are crucial to vaccine behavior, have been a less explored theoretical tenet of EPPM due to the challenges in convincingly and ethically manipulating efficacy via text-based information (Popova, 2012).
The affordances of VR provide a novel approach to operationalizing efficacy components in health messages as agentic experiences, allowing users to practice the recommended behavior and immediately observe the efficacy of the behavior. Specifically, this study employed virtual time travel (Ahn, 2021) to blend the efficacy information into enactive virtual experiences. Virtual time travel offers immersive and sensory-rich experiences of shifting the tempo and sequence of events in the mediated environment. In health messages, virtual time travel can help illustrate the threat of negative consequences resulting from undesirable health behaviors (e.g., not getting vaccinated), and allow users to travel back in time to modify their behavior (e.g., getting vaccinated) to boost efficacy.
Thus, we investigated the impact of virtual time travel on health attitudes and behaviors in the context of influenza vaccination by leveraging VR’s ability to control the tempo and sequence of virtual events. We also investigated how the psychological experiences of virtual time travel affect efficacy appraisals of VR health messages. Lastly, we demonstrated how strategically designed VR health messages can influence vaccination intentions for oneself and others in the community.
Communicating the critical importance of influenza vaccines
Seasonal influenza presents a substantial public health threat, causing 3–5 million severe cases annually worldwide (World Health Organization, 2018). Although healthy adults typically do not face high risks for serious illness, even mild illness from influenza can disrupt daily life and work performance (Nichol et al., 2005). Young adults are especially susceptible to the contagious virus in social and educational settings and can transmit the virus to others who are more vulnerable to serious illness (Kar-Purkayastha et al., 2009).
In 2019–2022, the influenza season coincided with the COVID-19 pandemic. Ecological reports indicated that influenza vaccination is crucial to reduce the burden on healthcare systems (Marín‐Hernández et al., 2021). When comorbidities are present, such as immunodeficiency and COVID-19, influenza infections pose a greater risk of severe illness and death. During health crises like a pandemic, uncertainty and a sense of helplessness may exacerbate fear and anxiety among the public, leading to misguided protective behaviors (Garfin et al., 2020).
The U.S. Centers for Disease Control and Prevention (CDC, 2021) recommends annual influenza vaccinations for everyone aged 6 months and older, with the exception of those with medical contraindications. However, influenza vaccination coverage in 2020–2021 among adults was only 50.2%, far below the U.S. government’s 70% target. Other countries also grapple with low vaccination rates. For example, Taiwan reported influenza vaccination rates of only 25%–31% across the entire country (Lee, 2020). Vaccine-hesitant individuals often express skepticism at the necessity and efficacy of the vaccine (Dubé et al., 2013). Individuals may delay or refuse to vaccinations when they do not feel threatened by infectious diseases and when they perceive vaccination as insufficient to overcome the threat. Psychological barriers to vaccine acceptance include low perceived effectiveness of the vaccine (low response efficacy) and the lack of perceived ability to get vaccinated to prevent the contraction and spread of the virus (low self-efficacy; Witte et al., 1996). These psychological aspects of vaccine hesitancy are also related to misconceptions about the vaccine and susceptibility to misinformation (Betsch et al., 2015). Therefore, emphasizing threat alone may not be sufficient to boost vaccination rates, particularly among vaccine-hesitant populations.
EPPM and the importance of efficacy appraisals
Fear appeals in health messages attempt to elicit fear by presenting threatening consequences of maladaptive health behavior to persuade audiences to adopt preventive actions (Witte & Allen, 2000). The EPPM (Witte, 1992, 1994) outlines conditions for the effectiveness of fear appeals based on the interaction between perceived threat and efficacy.
According to EPPM, individuals respond to fear-based health messages through two cognitive appraisals aimed at coping with emotional fear, influenced by threat and efficacy message components (Witte, 1992, 1994). Threat appraisal occurs when a message contains threat elements signaling the severity of the risk and individuals’ susceptibility to it. The audience assesses the degree of perceived threat, which consists of perceived severity (“beliefs about the significance or magnitude of the threat”) and perceived susceptibility (“beliefs about one’s risk of experiencing the threat”; Witte et al., 1996, p. 320). If perceived threat is sufficiently high, individuals experience fear and are motivated to perform efficacy appraisals by evaluating the extent to which the message provides efficacious and feasible preventive strategies. Perceived efficacy is determined by the perceived ability to implement the recommended behavior (i.e., self-efficacy) and the effectiveness of the behavior (i.e., response efficacy; Popova, 2012).
EPPM highlights that efficacy perceptions are crucial for predicting the persuasive success of fear appeals. When perceived threat is accompanied by high efficacy perceptions, individuals are motivated to prevent the foreseeable threat by forming intentions to adopt the recommended behavior (i.e., danger control). If perceived efficacy is insufficient to assuage perceived threat, people attempt to control their fear by rejecting the message, leading to maladaptive responses such as denial and avoidance (i.e., fear control). Therefore, to effectively promote adaptive coping strategies, health messages should clearly deliver efficacy information to help individuals mitigate fear.
Virtual time travel for efficacy appraisals of health messages
While threat elements in a message can be easily manipulated in empirical research to induce perceived threat, efficacy manipulations have been challenging and sparse in the EPPM literature (Popova, 2012). In text-based messages, for example, experimental manipulation of efficacy has often involved devaluing the information about the recommended behavior to manipulate low and high levels of efficacy elicitation, such as describing side effects of vaccines (Ort & Fahr, 2018), which could raise ethical concerns in some cases (Popova, 2012; Witte, 1994). Consequently, communication scholars have often resorted to treating efficacy as an individual difference (Witte & Morrison, 1995) or by not providing efficacy information in the control condition (Muthusamy et al., 2009).
Virtual simulation in VR may offer an alternative, serving as a powerful source of efficacy appraisals that offers users a chance to practice and execute problem-solving in a health-related risk scenario. According to social cognition theory (Bandura, 1977), common sources of self-efficacy include performance accomplishment (enactive experience), observational experience, and verbal persuasion, with the actual performance of the recommended action being the most effective. For vaccine communication, efficacy manipulation in VR may provide participants with the chance to receive the vaccine and immediately experience its benefits, instead of overstating or devaluing the information in the message. By directly and safely engaging in the recommended action in VR, individuals can practice how to act in real situations.
This study implements enactive experiences of health behavior via virtual time travel, allowing users to move backward in time in the mediated environment, impacting the tempo and the flow of the narrative (Ahn, 2016, 2021; Friedman et al., 2014). Humans have natural abilities for mental time travel by reconstructing past episodic memories and imagining hypothetical scenarios to prepare for foreseeable future events (Suddendorf et al., 2009). Virtual time travel integrates this cognitive ability of mental time travel to encourage audiences to consider different temporal points during message processing. Rich sensory details in VR can substantially reduce users’ cognitive effort to mentally construct past or future events while engaging in events that may not be possible in the real world (e.g., fighting against viruses inside the body).
Friedman et al. (2014) had participants intervene in a moral dilemma via virtual time travel in VR and found that time travel increased perceived guilt over the dilemma compared with those who experienced the same event without time travel. Ahn (2016) found that virtually experiencing negative future consequences of present-day health behaviors promoted the adoption of healthy attitudes and behaviors compared with the same health message presented as a pamphlet. Leveraging virtual time travel to manipulate the efficacy component of persuasive messages is anticipated to bring about significant cognitive, affective, and behavioral changes.
This study seeks to test the feasibility of using virtual time travel as a novel way to present and operationalize efficacy components as direct experiences in VR. Experiencing negative consequences in VR (i.e., contracting influenza) highlights the threat posed and elicits fear. Then, users may travel back in time for a chance to prevent the negative outcome. The time travel experience in this study will allow users to practice the preventive behavior by virtually receiving a vaccine to prevent infection (i.e., increase self-efficacy), as well as experience the effectiveness of the preventive behavior (i.e., increase response efficacy). If virtual time travel successfully elicits efficacy perceptions, having the opportunity to eliminate the threat in VR may alleviate the fear elicited during the threat appraisal stage. Successful efficacy appraisals activate danger control rather than fear control, prompting the adoption of the recommended behavior (Popova, 2012; Witte, 1994). Therefore, we ask the following research questions:
RQ1: How will virtual time travel elicit efficacy perceptions, comprising of (a) perceived self-efficacy and (b) response efficacy, and ultimately, (c) vaccination intention (i.e., danger control)?
RQ2: How will virtual time travel impact emotional fear (i.e., fear control)?
The moderating effect of interactivity
Structural features of VR also offer unique psychological experiences that bolster user engagement with the health message. In VR, users’ perceptual systems (e.g., visual, audio, and motor) are surrounded by the virtual environment and its content, characterizing immersion (Cummings & Bailenson, 2016; Slater & Sanchez-Vives, 2016). While immersed, users’ behavioral engagement is potentiated by interactivity that allows users to modify the form and content of mediated experiences and receive immediate feedback (Pan & Hamilton, 2018; Steuer, 1992; Sundar, 2008). This study aims to examine how varying levels of interactivity in VR can impact efficacy appraisals of the health message, thereby influencing attitudes and behavioral intentions.
Interactivity in VR is distinguished from other media (e.g., websites) by affording a range of activities for users to control mediated experience (Sundar, 2008). Unlike other digital media wherein users’ behavioral inputs are limited to indirect devices (e.g., mouse), VR interactivity affords direct interactions with virtual contents through locomotion, object manipulation, and changing perspectives, while engaging in full-body movements (Pan & Hamilton, 2018). Structural features of VR, such as head-body tracking and stereoscopic vision, contribute to facilitating these embodied activities. User activities can be further supported by contents of the virtual environment, such as various virtual objects that invite actions (e.g., opening the door and talking to a virtual agent).
According to Sundar (2008), interactivity is enriched through active media use. Virtual experiences can vary on a passivity–activity continuum. VR users may access content through low interactivity (e.g., mere observation and 360° movie) or high interactivity (e.g., active/agentic control). When VR affords only viewing of the mediated environment, users perceive the virtual experience as more indirect as if they were observing someone else’s behavior (Lin, 2013). As social cognition theory (Bandura, 1977) states, enactive, direct experiences yield strong efficacy perceptions than observations because personal experiences better indicate one’s ability to perform the action.
Therefore, interactivity in VR is anticipated to moderate the impact of virtual time travel by allowing users to actively engage with virtual events. This study operationalizes interactivity in VR based on Lin (2013) and social cognition theory (Bandura, 1977), wherein high interactivity is defined as mediated enactive experiences (active control) and low interactivity as mediated observational experiences (passive viewing). Active control during media use results in greater affective and cognitive outcomes than passive observers (Lin, 2013). Therefore, we hypothesize:
H1: Interactivity in VR will moderate the effect of efficacy appraisal via virtual time travel. High interactivity (active control) conditions will amplify perceived efficacy, leading to higher perceived (a) self-efficacy and (b) response efficacy regarding influenza vaccination, compared with low interactivity (passive viewing) conditions.
Mediators of message processing in VR: Spatial presence and message involvement
Immersive and interactive affordances of VR shape unique experiential states of spatial presence (place illusion), plausibility illusion, and embodiment that fundamentally shape users’ psychological and behavioral responses to mediated content (Pan & Hamilton, 2018; Slater, 2009; Slater & Sanchez-Vives, 2016; Wirth et al., 2007). This study seeks to clarify the role of VR interactivity in these experiential states and in facilitating users’ persuasive message processing (efficacy appraisal and intention), which were previously underexplored in the EPPM framework.
Interactivity in VR has been shown to increase users’ spatial presence—subjective feelings of “being there” in a virtual environment (Biocca, 1997; Lombard & Ditton, 1997; Slater, 2009; Wirth et al., 2007). Although nonsensory experiences can elicit general feelings of presence via mental construction, presence in digital environments is closely tied to sensory experiences and interaction qualities supported by possible actions, behavioral mapping, and immediate feedback (Pan & Hamilton, 2018, Wirth et al., 2007). For example, the stereoscopic display in VR immediately occupies users’ vision with the 3D virtual space, offering the illusion of being in the space. Users also continuously interact with mediated content via physical movements, embodied in their virtual body (avatar). Accordingly, dynamic visuomotor changes in users’ field of view corresponding to their actions create a plausibility illusion so that users feel as if the events were actually happening (Slater, 2009). Wirth et al. (2007) explicated the process model of spatial presence, linking users’ behavioral engagement with ongoing updates in mental representations, theorizing that spatial presence arises when users perceive their self-location within a mediated environment and the possible actions they can perform within the virtual environment.
Thus, interactivity in VR can promote spatial presence by offering a wide range of embodied action capabilities (Wirth et al., 2007). Previous work demonstrated that spatial presence in immersive VR intensifies the persuasive outcome of health messages (Ahn et al., 2019; Nowak et al., 2020). In the context of vaccination messages, VR enhanced spatial presence and vaccination intentions compared with static modalities that delivered the same message (e.g., video and e-pamphlet; Nowak et al., 2020).
Spatial presence in VR induces users to pay more attention to mediated experiences and become more readily involved in the message. We conceptualize individuals’ message involvement as motivation to process message components (Baker & Lutz, 2000) and to perceive the message as personally relevant (Wang, 2006). Previous research found that high interactivity in a website that delivered vaccination messages increased message involvement compared with a website with low interactivity (Kuang & Cho, 2016). Message involvement mediated the interaction among perceived threat, response efficacy, and intention for information seeking.
VR interactions are anticipated to invite direct engagement with users’ sensory systems and embodied movements, facilitating spatial presence. High spatial presence can mentally bind users to the mediated environment (Wirth et al., 2007), so that users feel as if what is happening in VR is happening to them (Slater, 2009), and thus highly relevant. Accordingly, users may be motivated to allocate more attention to the efficacy components of the message during virtual experiences (Baker & Lutz, 2000), facilitating efficacy appraisals as specified in EPPM (Witte, 1992):
H2: Interactivity in VR will be indirectly and positively related to vaccination intention, sequentially mediated through spatial presence, message involvement, and perceived efficacy (H2a: self-efficacy, H2b: response efficacy).
Expanding EPPM to consideration of others
VR affords the ability to observe the threat of health risks and the efficacy of preventive measures in others, as a result of the users’ actions in the virtual world (Nowak et al., 2020). To date, the EPPM framework has been primarily tested on threat and efficacy perceptions related to the self. Because the risk of contagious diseases like influenza involves concerns of both the self contracting the virus and spreading the virus to others, vaccination messages need to emphasize the importance of community immunity, defined as indirect protection of susceptible individuals when a large portion of a community is immune to an infectious disease (Anderson & May, 1985). Vaccination messages highlighting community immunity brought about increased vaccination intention, knowledge, and positive attitudinal changes (Hakim et al., 2019).
Applying EPPM to threat and efficacy perceptions for others is important in the context of influenza, for which healthy adults are a critical subpopulation in preventing the contagious respiratory illness from spreading to more susceptible people. Therefore, we asked if EPPM could expand to include considerations of others:
RQ3: How will patterns of perceived threat, perceived efficacy, and vaccination intention following exposure to a vaccination message in VR differ between individuals’ perceptions regarding the self versus perceptions regarding others?
Methods
Participants and design
We employed a 2 (interactivity: active vs. passive) × 2 (virtual time travel: yes vs. no) between-subjects experiment. Participants were randomly assigned to one of four experimental conditions. A priori power analysis using the pwr package in R (Champely, 2018) suggested a minimum of 32 participants per cell for detecting a moderate (ηp2 = 0.06) to large effect (ηp2 = 0.14) in a two-way analysis of variance with an α = 0.05 (Cohen, 1988). Although no similar studies were found to directly inform effect sizes, previous research using VR to fast-forward through time to simulate consequences of unhealthy behavior (Ahn, 2016) as well as research on the impact of interactivity on the effectiveness of health risk communication (Nah & Oh, 2021) suggested moderate to large effects. A total of 186 participants were recruited from a national university in Taiwan. People who had a history of contracting influenza, had been or planned to get vaccinated, or had any medical conditions that prevented them from vaccination were screened during recruitment. Eight participants were excluded as outliers. The final sample (N = 178) consisted of 48 males and 130 females aged 18–39 (M = 21.62, SD = 2.78). The protocol was approved following the Institutional Review Board guidelines (U.S. protocol: PROJECT00002756, Taiwan protocol: NCCU-REC-202001-I001).
Procedure and stimulus
After obtaining informed consent, participants were guided to a space measuring 3 × 2.5 m. A VR scenario was created using the Unity engine. All participants experienced the scenario in immersive VR using HTC Vive head-mounted display (HMD) from a first-person perspective. Participants did not see their avatars in a mirror or a third-person view so that their responses would not be influenced by avatar characteristics, but they could see their location and controller position based on their movements.
Interactivity manipulation
Participants in active control conditions were presented with the HMD and two-hand controllers to interact with virtual objects. Participants in passive viewing conditions did not have hand controllers and could watch the scenario using head orientation inside the HMD. All participants were allowed to walk freely in the physical space.
Common scenario
Participants first experienced the threat of contracting the influenza virus with a sneeze and visual effects showing the spreading of the virus to others in a restaurant. Then the narrative transitioned to the inside of the infected body and depicted that the immune system alone was unable to defeat the virus without antibodies from the vaccine. Participants in the active control condition experienced this scene as an interactive task with the ability to use hand controllers to distribute white blood cells against influenza viruses. The passive viewing condition presented a video of this in the HMD. The narrative then showed two people from vulnerable populations (a child and senior citizen) who were hospitalized due to influenza. At this point, the VR scenario ended for participants in the no virtual time travel condition.
Virtual time travel manipulation
After the hospital scene, participants in the virtual time travel condition were given the chance to travel back in time to get a flu vaccine. After vaccination, participants engaged in the task to fight against flu viruses again with (active) or without handheld controllers (passive) and could successfully protect the body with the antibodies from the vaccine. The scenario ended with participants back in the restaurant where they would not inadvertently spread the flu to others after vaccination.
After the VR experience, all participants received an information sheet that indicated when and where they could get the vaccine so that they could receive basic information about vaccination opportunities regardless of conditions. Participants then completed a post-test survey asking about perceived spatial presence, message involvement, and EPPM constructs. Full scripts and demonstration of the virtual world (Figure 1) are available on our Open Science Framework (OSF) page.1
Measures
The full list of measurement items and the item purification table can be found on our OSF page. All scales, unless otherwise noted, used 5-point scales ranging from strongly disagree to strongly agree.
Spatial presence
The Spatial Presence Experience Scale (Hartmann et al., 2015) assessed perceived spatial presence (α = 0.81, M = 3.6, SD = 0.63) with four items on the perceived self-location and four items on the extent to which participants could move and perform actions in the virtual environment (possible actions).
Message involvement
Five items adapted from Cox and Cox (2001) measured participants’ cognitive involvement with the message during the virtual experience (e.g., “The message presented in VR made me think.”), α = 0.74, M = 3.95, SD = 0.64.
EPPM constructs
Perceived threat included perceived severity and susceptibility dimensions, while perceived efficacy consisted of perceived self-efficacy and response efficacy dimensions. Each of these four constructs was assessed regarding the self (i.e., getting influenza) and others (i.e., spreading influenza to others). Each construct measurement used three items.
Perceived threat
Perceived severity (αself = 0.89, Mself = 3.83, SDself = 0.79; αother = 0.88, Mothers = 4.21, SDothers = 0.68) was measured with items asking participants to rate the seriousness of influenza infection (e.g., “The consequences of getting the flu are serious threats”) and items about the seriousness of spreading the infection to others, adapted from Witte et al. (1996). Perceived susceptibility (αself = 0.84, Mself = 3.84, SDself = 0.81; αother = 0.81, Mothers = 4.26, SDothers = 0.55) items measured judgments about the likelihood of experiencing the threat (e.g., “I am at risk for getting the flu.”; adapted from Witte et al., 1996) and spreading the threat to others (e.g., “If I spread the flu to someone, the person would be at risk for getting the flu”; adapted from Totzkay et al., 2020).
Perceived efficacy
Participants’ self-efficacy (αself = 0.83, Mself = 3.92, SDself = 0.75; αother = 0.87, Mothers = 3.81, SDothers = 0.81) was measured on their ability to receive a vaccine to prevent them from influenza infection (e.g., “I can get a vaccine to prevent the contraction of the flu.”) and to protect others from spreading the infection. Perceived response efficacy (αself = 0.78, Mself = 4.13, SDself = 0.52; αother = 0.87, Mothers = 3.98, SDothers = 0.69) comprised items on perceptions about the effectiveness of getting a flu vaccine to prevent self-contraction (e.g., “Getting a flu vaccination works in preventing the contraction of the flu.”) and protecting others (Gore & Bracken, 2005; Witte et al., 1996).
Vaccination intention
Behavioral intention was assessed using four items for personal intentions to get a flu vaccine (α = 0.89, M = 2.94, SD = 0.86) and four items for intentions to encourage others to get vaccinated (α = 0.93, M = 2.93, SD = 0.99), adapted from Totzkay et al. (2022).
Negative affect
To determine whether participants experienced emotional fear associated with the threat elements of the message, we measured six items of fear (α = 0.90, M = 2.04, SD = 0.82) from the Positive and Negative Affect Schedule-Expanded Form (PANAS-X; Watson & Clark, 1999). The six items of guilt (α = 0.94, M = 1.63, SD = 0.75) in PANAS-X were also included to measure negative affect associated with spreading the virus to others.
Covariates
Participants’ personal issue involvement (α = 0.88, M = 3.21, SD = 0.69) with influenza was measured as potential covariates using the nine-items scale adapted from Quick and Stephenson (2007) to determine participants’ existing interest. Participants’ familiarity associated with VR (M = 3.85, SD = 1.15) was also measured as a covariate using a single question on a 6-point scale (“How familiar are you with VR?”).
Results
Manipulation check
To evaluate the effectiveness of the interactivity manipulation, we measured participants’ perceived interactivity using seven items adapted from Park and Yoo (2020). Perceived interactivity was higher in the active control conditions (M = 3.48, SD = 0.58) than in the passive viewing conditions (M = 3.3, SD = 0.61), t(177) = 2.01, p = .023, d = 0.6.
Threat induction
Participants reported high levels of perceived threat, calculated by the mean of perceived severity and perceived susceptibility (Mself = 3.84, SDself = 0.6; Mothers = 4.23, SDothers = 0.52). The median and mode scores were 4 on a 5-point scale. Across all conditions, the mean values of perceived threat ranged from 3.81 to 4.31, indicating that the virtual experience induced sufficient levels of perceived threat for all conditions to warrant efficacy appraisal.
Confirmatory factor analysis
Confirmatory factor analysis (CFA) assessed the fit of two measurement models focusing on self and others using the maximum-likelihood estimation. Several guiding principles (e.g., Fornell & Larcker, 1981) were applied to both models to ensure reliability and validity of all measures. As a result, seven measurement items were excluded from the analyses (Levine et al., 2006). To ensure comparability between the self- and others-model, we aimed at keeping the two models consistent in terms of measurement structure (detailed item purification table: https://osf.io/cdyxg).
Table 1 presents internal consistencies and validity indices for the final CFA models. Each model had eight factors including four EPPM constructs (perceived severity, susceptibility, self-efficacy, and response efficacy), spatial presence, message involvement, fear (self-model), guilt (others-model), and behavioral intention. We defined spatial presence as a second-order factor comprising self-location and possible action, based on Hartmann et al. (2015). Due to the large number of observed variables (number of items = 38) for the given sample size (N = 178; variable-to-sample ratio = 4.71), two intention constructs (vaccination intention, α = 0.89 and intention to encourage others, α = 0.93) were averaged to manage the variable-to-sample ratio to larger than 5 (final ratio = 6.88; Bentler & Chou, 1987). The final models fit the data well for the self-model: χ2 (267) = 349.75, p < .05, CFI = 0.96, RMSEA = 0.04 confidence interval (CI) [0.03–0.05], SRMR = 0.06, and the others-model: χ2 (269) = 398.67, p < .05, CFI = 0.95, RMSEA = 0.05 CI [0.04–0.06], SRMR = 0.05.
Composite reliability, validity indices, and correlations among latent variables
Direct effects . | CR . | AVE . | MSV . | F1 . | F2 . | F3 . | F4 . | F5 . | F6 . | F7 . | F8 . |
---|---|---|---|---|---|---|---|---|---|---|---|
Perceptions for self(S) | |||||||||||
F1. Spatial presence | 0.85 | 0.73 | 0.34 | 0.86 | |||||||
F2. Message involvement | 0.75 | 0.5 | 0.34 | 0.59*** | 0.71 | ||||||
F3. Severity(S) | 0.9 | 0.81 | 0.14 | 0.09 | 0.37*** | 0.9 | |||||
F4. Susceptibility(S) | 0.85 | 0.74 | 0.05 | −0.11 | −0.03 | 0.09 | 0.86 | ||||
F5. Self-efficacy(S) | 0.83 | 0.71 | 0.38 | 0.13 | 0.2* | 0.28** | 0.02 | 0.84 | |||
F6. Response efficacy(S) | 0.78 | 0.65 | 0.38 | 0.01 | 0.3** | 0.34*** | 0.22* | 0.62*** | 0.8 | ||
F7. Negative affect-fear | 0.91 | 0.63 | 0.04 | 0.1 | 0.2* | 0.09 | 0.05 | −0.03 | −0.16 | 0.8 | |
F8. Behavioral intention | 0.78 | 0.64 | 0.22 | 0.37** | 0.41*** | 0.29** | 0.16 | 0.32** | 0.47*** | −0.03 | 0.81 |
Perceptions for others(O) | |||||||||||
F1. Spatial presence | 0.84 | 0.72 | 0.36 | 0.85 | |||||||
F2. Message involvement | 0.75 | 0.5 | 0.36 | 0.6*** | 0.71 | ||||||
F3. Severity(O) | 0.89 | 0.79 | 0.21 | 0.19 | 0.3** | 0.89 | |||||
F4. Susceptibility(O) | 0.81 | 0.68 | 0.21 | 0.09 | 0.07 | 0.46*** | 0.83 | ||||
F5. Self-efficacy(O) | 0.87 | 0.76 | 0.65 | 0.11 | 0.16 | 0.15 | 0.25** | 0.88 | |||
F6. Response efficacy(O) | 0.87 | 0.78 | 0.65 | −0.06 | 0.13 | 0.19* | 0.36*** | 0.81*** | 0.87 | ||
F7. Negative affect-guilt | 0.94 | 0.71 | 0.08 | 0.03 | 0.29** | 0.12 | 0.13 | −0.05 | −0.16 | 0.85 | |
F8. Behavioral intention | 0.79 | 0.65 | 0.17 | 0.36** | 0.41*** | 0.29** | 0.29** | 0.29** | 0.15 | 0.02 | 0.81 |
Direct effects . | CR . | AVE . | MSV . | F1 . | F2 . | F3 . | F4 . | F5 . | F6 . | F7 . | F8 . |
---|---|---|---|---|---|---|---|---|---|---|---|
Perceptions for self(S) | |||||||||||
F1. Spatial presence | 0.85 | 0.73 | 0.34 | 0.86 | |||||||
F2. Message involvement | 0.75 | 0.5 | 0.34 | 0.59*** | 0.71 | ||||||
F3. Severity(S) | 0.9 | 0.81 | 0.14 | 0.09 | 0.37*** | 0.9 | |||||
F4. Susceptibility(S) | 0.85 | 0.74 | 0.05 | −0.11 | −0.03 | 0.09 | 0.86 | ||||
F5. Self-efficacy(S) | 0.83 | 0.71 | 0.38 | 0.13 | 0.2* | 0.28** | 0.02 | 0.84 | |||
F6. Response efficacy(S) | 0.78 | 0.65 | 0.38 | 0.01 | 0.3** | 0.34*** | 0.22* | 0.62*** | 0.8 | ||
F7. Negative affect-fear | 0.91 | 0.63 | 0.04 | 0.1 | 0.2* | 0.09 | 0.05 | −0.03 | −0.16 | 0.8 | |
F8. Behavioral intention | 0.78 | 0.64 | 0.22 | 0.37** | 0.41*** | 0.29** | 0.16 | 0.32** | 0.47*** | −0.03 | 0.81 |
Perceptions for others(O) | |||||||||||
F1. Spatial presence | 0.84 | 0.72 | 0.36 | 0.85 | |||||||
F2. Message involvement | 0.75 | 0.5 | 0.36 | 0.6*** | 0.71 | ||||||
F3. Severity(O) | 0.89 | 0.79 | 0.21 | 0.19 | 0.3** | 0.89 | |||||
F4. Susceptibility(O) | 0.81 | 0.68 | 0.21 | 0.09 | 0.07 | 0.46*** | 0.83 | ||||
F5. Self-efficacy(O) | 0.87 | 0.76 | 0.65 | 0.11 | 0.16 | 0.15 | 0.25** | 0.88 | |||
F6. Response efficacy(O) | 0.87 | 0.78 | 0.65 | −0.06 | 0.13 | 0.19* | 0.36*** | 0.81*** | 0.87 | ||
F7. Negative affect-guilt | 0.94 | 0.71 | 0.08 | 0.03 | 0.29** | 0.12 | 0.13 | −0.05 | −0.16 | 0.85 | |
F8. Behavioral intention | 0.79 | 0.65 | 0.17 | 0.36** | 0.41*** | 0.29** | 0.29** | 0.29** | 0.15 | 0.02 | 0.81 |
Note. * p < .05, ** p < .01, *** p < .001.
Composite reliability, validity indices, and correlations among latent variables
Direct effects . | CR . | AVE . | MSV . | F1 . | F2 . | F3 . | F4 . | F5 . | F6 . | F7 . | F8 . |
---|---|---|---|---|---|---|---|---|---|---|---|
Perceptions for self(S) | |||||||||||
F1. Spatial presence | 0.85 | 0.73 | 0.34 | 0.86 | |||||||
F2. Message involvement | 0.75 | 0.5 | 0.34 | 0.59*** | 0.71 | ||||||
F3. Severity(S) | 0.9 | 0.81 | 0.14 | 0.09 | 0.37*** | 0.9 | |||||
F4. Susceptibility(S) | 0.85 | 0.74 | 0.05 | −0.11 | −0.03 | 0.09 | 0.86 | ||||
F5. Self-efficacy(S) | 0.83 | 0.71 | 0.38 | 0.13 | 0.2* | 0.28** | 0.02 | 0.84 | |||
F6. Response efficacy(S) | 0.78 | 0.65 | 0.38 | 0.01 | 0.3** | 0.34*** | 0.22* | 0.62*** | 0.8 | ||
F7. Negative affect-fear | 0.91 | 0.63 | 0.04 | 0.1 | 0.2* | 0.09 | 0.05 | −0.03 | −0.16 | 0.8 | |
F8. Behavioral intention | 0.78 | 0.64 | 0.22 | 0.37** | 0.41*** | 0.29** | 0.16 | 0.32** | 0.47*** | −0.03 | 0.81 |
Perceptions for others(O) | |||||||||||
F1. Spatial presence | 0.84 | 0.72 | 0.36 | 0.85 | |||||||
F2. Message involvement | 0.75 | 0.5 | 0.36 | 0.6*** | 0.71 | ||||||
F3. Severity(O) | 0.89 | 0.79 | 0.21 | 0.19 | 0.3** | 0.89 | |||||
F4. Susceptibility(O) | 0.81 | 0.68 | 0.21 | 0.09 | 0.07 | 0.46*** | 0.83 | ||||
F5. Self-efficacy(O) | 0.87 | 0.76 | 0.65 | 0.11 | 0.16 | 0.15 | 0.25** | 0.88 | |||
F6. Response efficacy(O) | 0.87 | 0.78 | 0.65 | −0.06 | 0.13 | 0.19* | 0.36*** | 0.81*** | 0.87 | ||
F7. Negative affect-guilt | 0.94 | 0.71 | 0.08 | 0.03 | 0.29** | 0.12 | 0.13 | −0.05 | −0.16 | 0.85 | |
F8. Behavioral intention | 0.79 | 0.65 | 0.17 | 0.36** | 0.41*** | 0.29** | 0.29** | 0.29** | 0.15 | 0.02 | 0.81 |
Direct effects . | CR . | AVE . | MSV . | F1 . | F2 . | F3 . | F4 . | F5 . | F6 . | F7 . | F8 . |
---|---|---|---|---|---|---|---|---|---|---|---|
Perceptions for self(S) | |||||||||||
F1. Spatial presence | 0.85 | 0.73 | 0.34 | 0.86 | |||||||
F2. Message involvement | 0.75 | 0.5 | 0.34 | 0.59*** | 0.71 | ||||||
F3. Severity(S) | 0.9 | 0.81 | 0.14 | 0.09 | 0.37*** | 0.9 | |||||
F4. Susceptibility(S) | 0.85 | 0.74 | 0.05 | −0.11 | −0.03 | 0.09 | 0.86 | ||||
F5. Self-efficacy(S) | 0.83 | 0.71 | 0.38 | 0.13 | 0.2* | 0.28** | 0.02 | 0.84 | |||
F6. Response efficacy(S) | 0.78 | 0.65 | 0.38 | 0.01 | 0.3** | 0.34*** | 0.22* | 0.62*** | 0.8 | ||
F7. Negative affect-fear | 0.91 | 0.63 | 0.04 | 0.1 | 0.2* | 0.09 | 0.05 | −0.03 | −0.16 | 0.8 | |
F8. Behavioral intention | 0.78 | 0.64 | 0.22 | 0.37** | 0.41*** | 0.29** | 0.16 | 0.32** | 0.47*** | −0.03 | 0.81 |
Perceptions for others(O) | |||||||||||
F1. Spatial presence | 0.84 | 0.72 | 0.36 | 0.85 | |||||||
F2. Message involvement | 0.75 | 0.5 | 0.36 | 0.6*** | 0.71 | ||||||
F3. Severity(O) | 0.89 | 0.79 | 0.21 | 0.19 | 0.3** | 0.89 | |||||
F4. Susceptibility(O) | 0.81 | 0.68 | 0.21 | 0.09 | 0.07 | 0.46*** | 0.83 | ||||
F5. Self-efficacy(O) | 0.87 | 0.76 | 0.65 | 0.11 | 0.16 | 0.15 | 0.25** | 0.88 | |||
F6. Response efficacy(O) | 0.87 | 0.78 | 0.65 | −0.06 | 0.13 | 0.19* | 0.36*** | 0.81*** | 0.87 | ||
F7. Negative affect-guilt | 0.94 | 0.71 | 0.08 | 0.03 | 0.29** | 0.12 | 0.13 | −0.05 | −0.16 | 0.85 | |
F8. Behavioral intention | 0.79 | 0.65 | 0.17 | 0.36** | 0.41*** | 0.29** | 0.29** | 0.29** | 0.15 | 0.02 | 0.81 |
Note. * p < .05, ** p < .01, *** p < .001.
The impact of virtual time travel and interactivity
A two-way MANCOVA was conducted to examine RQ1 regarding the feasibility of virtual time travel to elicit perceived efficacy (self-efficacy, response efficacy) and behavioral intention (vaccination intention, intention to encourage others), and H1 regarding the moderating effect of interactivity, controlling for familiarity with VR and personal issue involvement as covariates. The Levene’s test indicated equality of error variance except for two dependent variables (self-efficacy and response efficacy for others). For these two efficacy variables, a conservative alpha level (α = 0.01) was used when evaluating ANOVA results (Allen & Bennett, 2007).
Overall, there was a significant main effect of virtual time travel on the combined dependent variables, F(5, 167) = 5.6, p < .001, ηp2 = 0.17, Wilks’ Λ = 0.83. However, interactivity in VR did not have significant main effect nor moderate the effects of virtual time travel; therefore, H1 was not supported. Table 2 lists test statistics of univariate ANOVAs. Personal issue involvement was a significant covariate, F(5, 167) = 8.25, p < .001, ηp2 = 0.23, Wilks’ Λ = 0.77, while VR familiarity was not significant.
Variables . | F . | p . | ηp2 . | F . | p . | ηp2 . |
---|---|---|---|---|---|---|
Effects: Virtual time travel | Effects: Interactivity | |||||
Perceived efficacy: Self | ||||||
Self-efficacy | 0.62 | .43 | 0 | 0.32 | .57 | 0 |
Response efficacy | 7.99 | .005** | 0.04 | 1.87 | .17 | 0.01 |
Perceived efficacy: Others | ||||||
Self-efficacy | 15.92 | <.001*** | 0.09 | 0.02 | .89 | 0 |
Response efficacy | 12.25 | .001** | 0.07 | 0.78 | .38 | 0.01 |
Intention to get vaccinated | 9.23 | .003** | 0.05 | 0.03 | .86 | 0 |
Intention to encourage others | 9.95 | .002** | 0.06 | 0.45 | .5 | 0 |
Variables . | F . | p . | ηp2 . | F . | p . | ηp2 . |
---|---|---|---|---|---|---|
Effects: Virtual time travel | Effects: Interactivity | |||||
Perceived efficacy: Self | ||||||
Self-efficacy | 0.62 | .43 | 0 | 0.32 | .57 | 0 |
Response efficacy | 7.99 | .005** | 0.04 | 1.87 | .17 | 0.01 |
Perceived efficacy: Others | ||||||
Self-efficacy | 15.92 | <.001*** | 0.09 | 0.02 | .89 | 0 |
Response efficacy | 12.25 | .001** | 0.07 | 0.78 | .38 | 0.01 |
Intention to get vaccinated | 9.23 | .003** | 0.05 | 0.03 | .86 | 0 |
Intention to encourage others | 9.95 | .002** | 0.06 | 0.45 | .5 | 0 |
Note. * p < .05, ** p < .01, *** p < .001.
Variables . | F . | p . | ηp2 . | F . | p . | ηp2 . |
---|---|---|---|---|---|---|
Effects: Virtual time travel | Effects: Interactivity | |||||
Perceived efficacy: Self | ||||||
Self-efficacy | 0.62 | .43 | 0 | 0.32 | .57 | 0 |
Response efficacy | 7.99 | .005** | 0.04 | 1.87 | .17 | 0.01 |
Perceived efficacy: Others | ||||||
Self-efficacy | 15.92 | <.001*** | 0.09 | 0.02 | .89 | 0 |
Response efficacy | 12.25 | .001** | 0.07 | 0.78 | .38 | 0.01 |
Intention to get vaccinated | 9.23 | .003** | 0.05 | 0.03 | .86 | 0 |
Intention to encourage others | 9.95 | .002** | 0.06 | 0.45 | .5 | 0 |
Variables . | F . | p . | ηp2 . | F . | p . | ηp2 . |
---|---|---|---|---|---|---|
Effects: Virtual time travel | Effects: Interactivity | |||||
Perceived efficacy: Self | ||||||
Self-efficacy | 0.62 | .43 | 0 | 0.32 | .57 | 0 |
Response efficacy | 7.99 | .005** | 0.04 | 1.87 | .17 | 0.01 |
Perceived efficacy: Others | ||||||
Self-efficacy | 15.92 | <.001*** | 0.09 | 0.02 | .89 | 0 |
Response efficacy | 12.25 | .001** | 0.07 | 0.78 | .38 | 0.01 |
Intention to get vaccinated | 9.23 | .003** | 0.05 | 0.03 | .86 | 0 |
Intention to encourage others | 9.95 | .002** | 0.06 | 0.45 | .5 | 0 |
Note. * p < .05, ** p < .01, *** p < .001.
Perceived efficacy and vaccination intention regarding the self
Answering RQ1, there was a significant main effect of virtual time travel on response efficacy of vaccination for self-protection, exhibiting higher response efficacy among participants who experienced the virtual time travel (M = 4.24, SD = 0.51) than those who did not travel back in time (M = 4.02, SD = 0.52), F(1, 174) = 7.99, p = .005, ηp2 = 0.04. However, no significant effect was found on self-efficacy for getting vaccinated. Virtual time travel led to higher vaccination intentions (M = 3.11, SD = 0.81) compared with no travel back in time (M = 2.76, SD = 0.88), F(1, 174) = 9.23, p = .003, ηp2 = 0.05 (Figure 2).
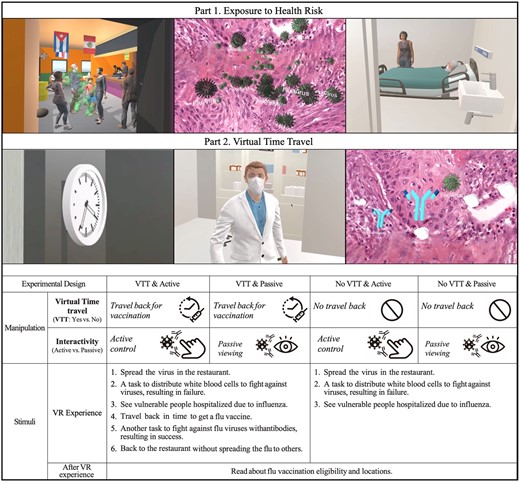
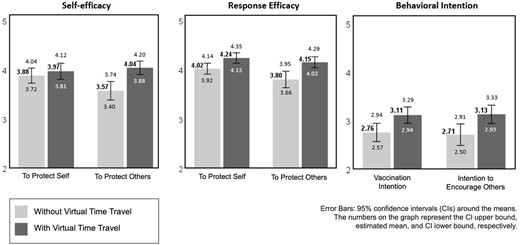
Effects of virtual time travel on efficacy perceptions and intention.
Perceived efficacy and vaccination intention regarding others
Participants in the virtual time travel condition reported higher self-efficacy to protect others (M = 4.04, SD = 0.66) than the no time travel condition (M = 3.57, SD = 0.89), F(1, 174) = 15.92, p < .001, ηp2 = 0.09. The virtual time travel also led to higher response efficacy of vaccination for protecting others (M = 4.15, SD = 0.54) than those without time travel (M = 3.8, SD = 0.78), F(1, 174) = 12.25, p < .001, ηp2 = 0.07. The main effect of virtual time travel led to significantly higher intentions to encourage others to get vaccinated (M = 3.13, SD = 0.91) than not having virtual time travel (M = 2.71, SD = 1.04), F(1, 174) = 9.95, p = .002, ηp2 = .06. Therefore, virtual time travel successfully enhanced efficacy perceptions and vaccination intention for protecting others in the community.
Reducing fear via virtual time travel
RQ2 asked whether experiencing virtual time travel would impact fear. A one-way ANOVA revealed that participants who had virtual time travel reported significantly reduced feelings of fear (M = 1.85, SD = 0.79) than those who did not experience the time travel (M = 2.24, SD = 0.8), F(1, 176) = 10.95, p = .001, ηp2 = 0.06.
Spatial presence and message involvement as mediators
H2 predicted that the effects of interactivity on behavioral intention would be sequentially mediated by spatial presence, message involvement, and perceived efficacy (H2a: self-efficacy, H2b: response efficacy). A set of mediation analyses were conducted using the PROCESS macro (model 6) with 5,000 bootstrap resamples (Hayes, 2018), with interactivity as a categorical independent variable (passive: 0, active: 1). Personal issue involvement and previous experience with VR were retained as covariates.
The indirect effect of interactivity in VR on behavioral intention was sequentially mediated through spatial presence, message involvement, and response efficacy, as a 5-step mediation, b = 0.01, SE = 0.01, 95% CI: [0.001, 0.025] (Figure 3). Interactivity in VR was positively linked to spatial presence, which in turn enhanced message involvement, followed by increased response efficacy and behavioral intention. However, the same 5-step mediation model involving self-efficacy was not significant, b = 0.002, SE = 0.003, 95% CI: [−0.001, 0.009]. Similarly, 5-step mediation models of efficacy for others did not yield a statistically significant result. Only H2a was supported regarding self-related perceptions.2
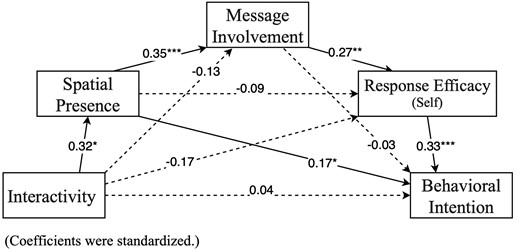
Path coefficients and indirect effects for serial mediation model (self-related perceptions).
Structural comparisons between the self- and others-EPPM models
For RQ3, we performed structural equation modeling (SEM) to explore and compare the structure of theoretical models focusing on the self- versus others-related perceptions. Spatial presence and message involvement were expected to predict cognitive (perceived severity, susceptibility, self-efficacy, and response efficacy) and affective processing (fear or guilt) of the health message. The model focusing on self included fear as negative affect. For the others-model, guilt was included because guilt appeal is often implemented in health messages for contagious diseases (e.g., Carcioppolo et al., 2017) and is relevant in the context of harming or protecting others.
Figure 4 shows predicted relationships and results for the structural model. Initial goodness-of-fit evaluation suggested a good fit for the self-model, but a mediocre fit for the others-model (CFI > 0.90, RMSEA close to 0.06, SRMR close to 0.08; Hu & Bentler, 1999), mainly due to the high error term correlation between self-efficacy and response efficacy, suggested by the modification index (MI). Correlating these error terms improved the model fit (self-model: χ2(277) = 376.88, p < .05, CFI = 0.95, RMSEA = 0.05 CI [0.03–0.06], SRMR = 0.08; others-model: χ2 (279) = 469.25, p < .05, CFI = 0.92, RMSEA = 0.06 CI [0.04–0.06], SRMR = 0.08).3 In both models, significant positive links were found between spatial presence → message involvement (βself = 0.55, βother = 0.57, p < .001), message involvement → perceived severity (βself = 0.35, p < .001, βother = 0.31, p < .05), and message involvement → negative affect (βself-fear = 0.27, βother-guilt = 0.26, p < .05). However, message involvement increased response efficacy only in the self-model (β = 0.24, p < .05). In the others-model, message involvement → self-efficacy path was marginally significant (β = 0.17, p = .082). Furthermore, perceived severity of oneself predicted both self-efficacy (β = 0.23, p < .05) and response efficacy (β = 0.26, p < .05) in the self-model, whereas perceived susceptibility of others increased self-efficacy (β = 0.24, p < .01) and response efficacy (β = 0.34, p < .001) in the others-model.
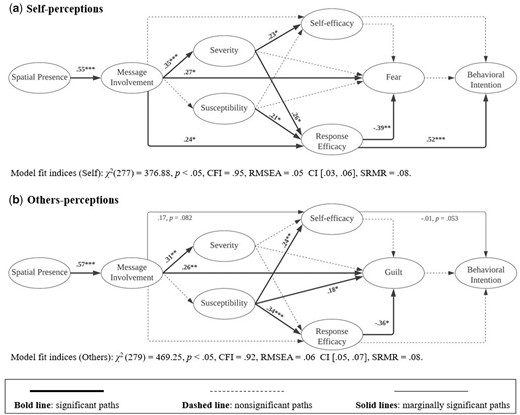
Structural equation models comparing perceptions about the self and others.
For outcome variables leading to behavioral intention, increased response efficacy significantly reduced fear (β = −0.39, p < .01) and enhanced behavioral intention (β = 0.52, p < .001) in the self-model. Although response efficacy reduced guilt (β = −0.36, p < .05), it did not predict behavioral intention in the others-model.
Discussion
VR affordances that allow users to interactively practice preventive behaviors can serve as a powerful source of efficacy perceptions in a health risk scenario. This study successfully operationalized efficacy components of vaccination communication via virtual time travel in VR, where participants could directly experience vaccine efficacy via behavioral engagement. Virtual time travel after experiencing threats of influenza increased participants’ perceived efficacy for both self-protection and preventing transmission to others, leading to vaccination intention. Furthermore, active engagement with interactive VR positively impacted spatial presence, enhancing message involvement, and ultimately increasing perceived response efficacy and behavioral intention. The affordances of interactivity and spatial presence may be fundamental to constructing high-impact health messages via immersive and interactive modalities to promote attitudinal and behavioral changes.
Efficacy information experienced as agentic interactions
VR simulations create novel and fictional scenarios that may be impossible to experience in real life while eliciting realistic cognitive, affective, and social responses. Unlike mental simulation, which relies on an individual’s cognitive abilities to imagine hypothetical scenarios (Taylor et al., 1998), VR affords agentic interactions so that user experiences directly and convincingly connect to health risk perception and prevention. In this study, virtual time travel was combined with a plausible real-world situation (i.e., contracting influenza without vaccination) to transcend temporal constraints of the real world.
Interactive experiences in VR can also transform how people access, process, and manipulate mediated content. For example, this study’s inner-body scenes transformed the scale of the participant’s perspective, allowing them to manipulate antibodies and white blood cells while simultaneously observing the outcome (i.e., defeating viruses) inside the human body. The virtual experience delivered health information through a microscopic perspective of vaccine efficacy in a more direct, unobtrusive, and experiential way than using a microscope as an external tool. Despite the fictitious narrative, spatial presence encouraged users to think and behave as if the virtual experiences were real (Lombard & Ditton, 1997), translating to real-world impact on attitudes and behaviors (Ahn et al., 2016).
Virtually rehearsing vaccination behaviors in VR seemed to positively impact efficacy perceptions for both the self and others, regardless of the interactivity level. In addition to enhanced efficacy perceptions, the current results demonstrated the robust effect of virtual time travel on mitigating fear, thereby promoting intentions to receive the vaccine and to encourage others to be vaccinated. These findings suggest that enactive experiences (Bandura, 1977) of vaccination (both real-life and virtual) may be critical in shaping vaccine efficacy perceptions for the self and others.
Underlying mechanisms of virtual experiences on message processing
This study delineated relationships between interactivity in VR and cognitive appraisals of message content as predicted in EPPM by extending previous discourse on passive to active interactivity (Lin, 2013; Sundar, 2008) to VR health messages. High spatial presence through interactivity encourages users to actively engage with message contents, facilitating cognitive and behavioral outcomes. When VR affords greater action capabilities, users can readily explore the content of the virtual environment, including objects, agents, and situations, positively affecting health message processing (threat and efficacy appraisals) as well as attitudinal and behavioral changes (behavioral intention).
Our results also echo previous work on message involvement as a significant predictor of attitude changes (Cauberghe et al., 2009; Ewoldsen et al., 2004). Message involvement was a critical mediator between spatial presence and response efficacy, which led to increased behavioral intention. Even when the modality offers high interactivity and spatial presence, users must be sufficiently involved with the message to process the threat and efficacy information. We suggest areas of expansion for EPPM with regard to emerging media platforms, to consider how the relationship between unique media features (e.g., interactivity) and user perceptions (e.g., spatial presence, message involvement) can impact health attitudes and behaviors.
Virtual health message processing to protect others
When copresent with others (real or virtual) in VR and engaging in social interactions, users feel as if they were with real people, having an awareness of others’ thoughts and emotions (i.e., social presence, Biocca, 1997; Oh et al., 2018). By allowing users to observe the threat of health risks and the efficacy of preventive measures in others, as a result of users’ actions in VR, we tested the extension of EPPM to include efficacy perceptions to protect others in the community.
Effects of virtual time travel on perceived efficacy for protecting others and for self-protection are distinct. While spatial presence in VR facilitated message involvement in both self- and others-models, causal patterns leading to efficacy perceptions and behavioral intention differed when considering the self versus others. Specifically, perceived severity of influenza for the self activated efficacy appraisals for self-protection, whereas perceived others’ susceptibility to influenza promoted efficacy appraisals to protect others. Response efficacy increased behavioral intention for the self, whereas response efficacy for others did not predict behavioral intention. The weak relationships in the others-model may be due to a third-person effect, or people’s tendency to believe others to be more influenced by media messages (Davison, 1983). Participants may think that others are more likely to be swayed by vaccine messages and seek vaccination without their encouragement.
Overall, our structural modeling confirmed that although perceiving spatial presence in VR can facilitate threat and efficacy appraisal and mitigate negative affect, successful danger control is only achieved through message involvement. Our exploration of structural models indicated that novel VR affordances can extend the tenets of EPPM to predict message effects on people’s behaviors to protect others, but the routes to behavioral changes for the self and others are distinct.
Limitations and future directions
The present findings identified the promising impact of virtual time travel in promoting perceived efficacy for influenza vaccination, but some limitations should be considered when interpreting the results. For instance, operationalizing efficacy message components as the enactive experience of virtual rehearsal (time travel) might have tested the role of the presence and absence of efficacy information. Because efficacy components of the health message were integrated into behavioral interactions in this study, participants in the no time travel condition did not receive corresponding efficacy information. Though comparing the presence and absence of efficacy information is a common research paradigm in EPPM research (Ahn, 2016; Muthusamy et al., 2009), addressing potential information discrepancies across conditions will minimize experimental confounds. For example, including an additional condition that provides equivalent efficacy information without virtual time travel could be considered.
In the results, virtual time travel only enhanced perceived response efficacy of vaccination, not self-efficacy in the ability to get vaccinated to protect oneself. Although a MANCOVA found significant effects of virtual time travel on self-efficacy and response efficacy for protecting others, mediation analyses and SEM did not yield sufficient statistical power. This may be due to unclear distinctions between efficacy components in the virtual experience. That is, the response efficacy components (effectively defeating the virus) could have been successfully blended into agentic experiences, whereas self-efficacy components (e.g., the perceived ability to get the vaccine) were not as detailed in these experiences. Future research should carefully design self-efficacy components in virtual experiences by employing various simulation techniques to help overcome different barriers to preventing a health threat (e.g., fear of side effects and economic hardship).
Additionally, MANCOVA results did not find significant main or interaction effects of VR interactivity. This could be because users in passive viewing conditions could still perceive some degree of interactivity in VR from its immersive features and head tracking, even with limited action capabilities. Future research may enhance variability between interactivity conditions by providing more interactive virtual objects.
Although EPPM was developed in the context of fear appeals, recent approaches suggest that emotional appeals focusing on positive or negative affect other than fear (e.g., pride and annoyance) can effectively persuade the audience when the message delivers high threat and high efficacy (Lewis et al., 2007). We found that high perceived efficacy significantly reduced emotional fear. However, successfully defeating the virus after the virtual time travel could have simultaneously elicited positive emotions, such as excitement and satisfaction. Moreover, VR users often express positive reactions and acceptance toward novel technology, exhibiting curiosity and interest (Schutte, 2020), which could have counter-balanced participants’ perceived threat, emotional fear, and/or presence. Therefore, measuring various discrete emotional states is encouraged.
The between-subject design employed in this study is a commonly accepted form of experimental test, but recent findings suggested that between-subject designs involving virtual embodiment can suffer from replicability issues (Richard et al., 2022). Future work should use a longitudinal design to examine how users’ affective states change over time in different stages of message processing and to clarify the effects of repeated exposure to virtual experiences on vaccination message processing and outcomes.
This study recruited university students as a vaccine-avoidant adult sample, but specific characteristics of young adults may not be generalizable to all adults. For example, younger adults are more resistant to cybersickness than older adults (Kim et al., 2021). Future research should test the effect of virtual time travel on health message processing using diverse community samples. In doing so, the effects of structural factors of VR that could impact user experiences should be carefully considered. Specifically, exposure time to VR and cybersickness, which varies greatly due to individual differences and can undermine user experiences, should be measured and controlled in analyses.
Conclusion
The power of VR lies in its affordances that facilitate user interaction with the message. When users perceive high spatial presence through interactivity in VR, they are encouraged to actively engage with meaningful components of health messages (e.g., threat and efficacy components), motivating intentional changes toward desirable health outcomes. Implications of current findings are not limited to influenza, and can extend to broader contexts of health communication that focus on the importance of preventive behaviors to protect not just the self but others in the community.
Supplementary material
Supplementary material is available at Journal of Computer-Mediated Communication online.
Data availability
The de-identified data file and supplemental materials are available at https://doi.org/10.17605/OSF.IO/B2UCX
Endnotes
We conducted several path analyses to explore mediation of spatial presence and message involvement on various dependent variables. These additional results are in OSF Supplementary Materials.
We justified correlating error terms of latent factors based on perceptual similarities between self-efficacy and response efficacy, which was stronger in the others-model (r = 0.81), than the self-model (r = 0.62).
Funding
This project was partially supported by the National Science and Technology Council, R.O.C. under grants #MOST 107-2410-H-004-061-SS3 and #109-2423-H-004-004-SS4.
Conflicts of interest: None declared.
Acknowledgments
The authors thank David Walker for developing the virtual environment stimulus; Chien Ho for reproducing the voice-over; and Tzu-Ching Chiu, Wai-Kin Wong, Wei-Lin Hsiao, Wei-Peng He, and Pei-Ching Sun for assisting with data collection.
References
